The initial level of injury and severity of volitional motor and clinically detectable sensory impairment has been considered the most reliable for predicting neurologic recovery of function after spinal cord injury (SCI). This consensus implies a limited expectation for physical rehabilitation interventions as important in the facilitation of recovery of function. The development of pharmacologic and surgical interventions has always been pursued with the intent of altering the expected trajectory of recovery after SCI, but only recently physical rehabilitation strategies have been considered to improve recovery beyond the initial prognosis. This article reviews the recent literature reporting emerging activity-based therapies that target recovery of standing and walking based on activity-dependent neuroplasticity. A classification scheme for physical rehabilitation interventions is also discussed to aid clinical decision making.
Clinicians, including physicians and therapists, have developed prognostications for expectations of functional outcomes after spinal cord injury (SCI) . Outcomes expected 1 year after injury are based on the initial level of injury and the initial degree of voluntary strength of the muscles below the level of injury . Rehabilitation planning and outcomes relative to independence, self-care, and mobility are based on the degree of neurologic impairment assessed by a standardized neurologic evaluation developed by the American Spinal Injury Association (ASIA) and termed the ASIA Impairment Scale (AIS) . The magnitude and rate of recovery depends on the initial injury severity and whether it is complete (AIS A), sensory incomplete (AIS B), or motor incomplete (AIS C or D) , with motor incomplete injuries showing a more rapid rate of recovery. The rate of recovery is measured by the relative change in the motor and sensory scores over time and declines substantially after 6 months to 1 year after SCI .
The loss of standing and walking after human SCI has been attributed to the dominance of supraspinal mechanisms over spinal mechanisms in the control of locomotion . Thus, pharmacologic and surgical interventions have been the predominant focus for altering the course of natural recovery by improving functional outcomes after SCI. These interventions are associated with medical management during the acute phase after SCI to diminish the damaging sequelae of acute SCI including swelling or cord impingement. From this vantage point, recovery has been equated with improvements according to the AIS evaluation of voluntary strength and sensation and associated functional gains in abilities .
Physical rehabilitation as compensation for irremediable deficits and new skill development
Physical rehabilitation after SCI has relied substantially on compensatory strategies for identified nonremediable impairments and deficits, because significant recovery of motor function was not expected beyond that defined by the clinical assessments. In addition, health care provider limitations have significantly reduced the number and duration of therapy sessions necessitating that therapists target immediate patient needs in preparation for discharge . New behavioral strategies are taught to accomplish tasks including rolling over in bed, getting up from the floor to a chair, and transferring in and out of a wheelchair . Each of these new skills relies on strengthening of muscles above the level of the lesion and use leverage, momentum, and substitution to aid in moving a weak or paralyzed body for new mobility skills . These individuals with SCI usually do not recover their preinjury ability to roll over in bed, dress, stand up, grasp a glass, or get in and out of a car, but instead develop an entirely new repertoire of movement strategies to accomplish daily activities or they remain dependent on others for assistance. The process of learning these new skills requires practice and repetition, and the ability to problem-solve to find unique approaches to maneuver successfully through the myriad of circumstances and environments of daily life.
Additionally, wheelchairs, assistive devices, and braces are incorporated into the new skill learning associated with mobility . Wheelchairs offer a means of alternative mobility from a seated position and require the acquisition of new skills to propel and maneuver the equipment using the arms, head, chin, breath, or hand controls. Leg braces and assistive devices provide stability for joints that cannot be activated voluntarily and when muscle strength is not adequate to support upright posture during standing and walking. For example, walking using leg braces and a walker is highly dependent on voluntary motor control above the level of lesion and assistance from the devices to achieve a new approach to stability and mobility. However, accomplishing the activities of daily living using compensatory strategies is not equivalent to recovery of motor control that restores preinjury capabilities (ie, walking upright at normal speed, negotiating obstacles with balance responses, and climbing stairs) .
Conventional gait training post-SCI is routinely conducted over ground. A tilt table, parallel bars, assistive devices, and braces are all used to achieve upright standing and compensate for lower extremity and trunk weakness or paralysis and possibly upper extremity weakness . Walking in the overground environment is certainly the end goal and thus readily experienced in this environment. However, without substantial evidence of recovery of voluntary control below the lesion, those with SCI are instructed to achieve walking via compensatory strategies. These strategies use passive external lower extremity support (braces) and upper extremity weight bearing (assistive devices) to overcome sensorimotor deficits.
Emerging physical rehabilitation: activity-based therapies for recovery of function after spinal chord injury
Evidence from basic and applied science for activity-dependent plasticity of the neural axis, including the spinal cord, has provided a new perspective on the role of physical rehabilitation for the recovery of motor function after SCI . Research studies in animals and humans that have found that retraining after SCI using the intrinsic physiologic properties of the nervous system can facilitate the recovery of function . This potential for retraining is based on activity-dependent plasticity driven by repetitive task-specific sensory input to spinal networks. These studies show that the spinal cord integrates supraspinal and afferent information and with repetitive practice can improve motor output. With the translation of these scientific findings to the human condition, rehabilitation strategies emerged that use the intrinsic processes of the nervous system in response to task-specific activity to advance and improve recovery of function after SCI .
Activity-based therapy has recently been promoted at prominent rehabilitation centers in the United States to describe their therapeutic interventions . However, the term is used ubiquitously often to describe the usual compensatory approaches to regain the ability to perform specific functions. In the scientific literature, activity-dependent plasticity is a term that has general consensus to indicate changes in the nervous or muscular systems that are driven by repetitive activity . Thus, activity-based therapy specifically refers to interventions that provide activation of the neuromuscular system below the level of lesion with the goal of retraining the nervous system to recover a specific motor task. The approach is to evaluate the neurophysiologic state below the level of lesion and phase of recovery and then use repetitive practice of the desired task to functionally reorganize the nervous system . So an activity-based therapy is an intervention that results in neuromuscular activation below the level of the lesion to promote recovery of motor function with the activation driven by the nervous system as most desirable.
The most prominent and well-developed activity-based therapy (physical rehabilitation) to date is “locomotor training” (LT) . A series of guiding principles for training has emerged in the translation of findings from basic science to the human condition . Scientists, examining the role of the spinal cord in controlling walking, discovered that cats with complete mid-thoracic transactions of the spinal cord could generate a stepping response after intense daily and long-term practice of the task of walking. Walking was facilitated by manual trainers assisting limb flexion, reflex extensor activity via pinching of the tail and/or anal region, while providing partial body weight support to the trunk by a sling suspension . Training involved optimizing normative stepping parameters including speed of stepping and appropriate kinematics and kinetics.
Emerging physical rehabilitation: activity-based therapies for recovery of function after spinal chord injury
Evidence from basic and applied science for activity-dependent plasticity of the neural axis, including the spinal cord, has provided a new perspective on the role of physical rehabilitation for the recovery of motor function after SCI . Research studies in animals and humans that have found that retraining after SCI using the intrinsic physiologic properties of the nervous system can facilitate the recovery of function . This potential for retraining is based on activity-dependent plasticity driven by repetitive task-specific sensory input to spinal networks. These studies show that the spinal cord integrates supraspinal and afferent information and with repetitive practice can improve motor output. With the translation of these scientific findings to the human condition, rehabilitation strategies emerged that use the intrinsic processes of the nervous system in response to task-specific activity to advance and improve recovery of function after SCI .
Activity-based therapy has recently been promoted at prominent rehabilitation centers in the United States to describe their therapeutic interventions . However, the term is used ubiquitously often to describe the usual compensatory approaches to regain the ability to perform specific functions. In the scientific literature, activity-dependent plasticity is a term that has general consensus to indicate changes in the nervous or muscular systems that are driven by repetitive activity . Thus, activity-based therapy specifically refers to interventions that provide activation of the neuromuscular system below the level of lesion with the goal of retraining the nervous system to recover a specific motor task. The approach is to evaluate the neurophysiologic state below the level of lesion and phase of recovery and then use repetitive practice of the desired task to functionally reorganize the nervous system . So an activity-based therapy is an intervention that results in neuromuscular activation below the level of the lesion to promote recovery of motor function with the activation driven by the nervous system as most desirable.
The most prominent and well-developed activity-based therapy (physical rehabilitation) to date is “locomotor training” (LT) . A series of guiding principles for training has emerged in the translation of findings from basic science to the human condition . Scientists, examining the role of the spinal cord in controlling walking, discovered that cats with complete mid-thoracic transactions of the spinal cord could generate a stepping response after intense daily and long-term practice of the task of walking. Walking was facilitated by manual trainers assisting limb flexion, reflex extensor activity via pinching of the tail and/or anal region, while providing partial body weight support to the trunk by a sling suspension . Training involved optimizing normative stepping parameters including speed of stepping and appropriate kinematics and kinetics.
Guiding principles of locomotor training
Guidelines for locomotor training provide a framework for clinical decision making, as well as a reference point for evaluating the potential application of any new modality, equipment, or therapeutic component within LT. Clinical choices can be made that are consistent with the framework (ie, no weight bearing on the upper extremities during training on the treadmill) for recovery or that are inconsistent (ie, use of a long-leg brace) and reflect a choice for compensation. Although training protocols used by researchers and clinicians vary , these guidelines represent a structured translation from basic science evidence for the neural control of walking to therapeutic principles for retraining walking. These guidelines will continue to be refined and clarified as research advances the science of locomotor training.
Four guiding principles of LT are built on the premise of robustly approximating the sensorimotor experience of walking through repetitive practice:
- 1.
Maximize load bearing by the lower extremities and minimize load bearing by the upper extremities. Increases in limb electromyographic amplitude are associated with increasing load bearing in both animals and humans after SCI as well as able-bodied individuals . This physiologic response to the sensory input associated with load bearing and translated into a guiding principle provides the opportunity to improve activation in muscles that under voluntary conditions (ie, manual muscle testing) are weak or do not produce a contraction. Visintin and Barbeau observed that shared load bearing between the upper and lower limbs diminished electromyographic (EMG) activity in the lower limbs. In contrast, providing partial body weight support through vertical suspension produced a relative increase in lower limb EMG activity. Thus, minimizing upper limb loading during retraining by use of hand rails or parallel bars is discouraged, and increasing vertical load bearing through the legs is encouraged.
- 2.
Optimize the sensory cues for walking. Normal walking speed for an adult ranges from 0.8 to 1.2 m/s and affords the spatial-temporal sequence of inputs that contribute to the characteristic sensorimotor experience of walking. Whether normal walking speed is a necessary component to the ensemble of activity-dependent experience producing a beneficial effect of LT continues to be a question of researchers . Beres-Jones and Harkema and Lunenburger and colleagues observed velocity-dependent modulation of EMG activity in persons with both incomplete and complete SCI and able-bodied individuals. Again, in an effort to increase muscle activation, the higher speeds may provide a stronger stimulus response.
- 3.
Optimize the kinematics (ie, trunk and extremities) for each motor task. One critical kinematic component to successful walking is the transition from stance to swing. This transition may be neurally activated by the sensory input associated with hip extension (relative to an upright trunk) and limb load bearing (muscle/tendon stretch, proprioception, cutaneous input), followed by unloading of the limb while transferring weight to the other limb. These two sensory elements, extension and load, are part of the essential ensemble of afferent input affording the transition and generation of activity from stance to swing or extension to flexion . Incorporating these elements into the training regime is critical to initiating and generating flexion in the gait cycle.Because the arms typically swing in reciprocal coordination with the lower limbs while walking, this automatic pattern and kinematic component may be of benefit in achieving a more complete sensory experience of walking. Furthermore, armswing may contribute to activity-dependent plasticity and development of appropriate balance responses and is thus encouraged by some researchers and trainers as opposed to a supportive function by the upper extremities.
- 4.
Maximize recovery strategies and minimize compensation strategies. Recovery strategies promote use of the inherent biology of the nervous system to generate motor responses within the usual kinematic framework. Visintin and Barbeau concluded that using parallel bars for upper extremity (UE) support produced a forward flexed trunk, asymmetry in gait, and use of compensatory strategies for swing initiation such as “hip-hiking” (eg, the trunk flexes laterally while raising the hip and advancing the leg forward with the knee extended). By comparison, vertical support provided a more upright trunk, hip extension and loading promoting the transition from stance to swing, and relatively less compensatory movement strategies while walking. The latter experience would be more consistent with the LT principles and a more appropriate choice for retraining the nervous system. Throughout LT, individuals are encouraged to attempt movements but are assisted, as needed, to perform them to achieve the task-specific sensory experience, ie, thus, movement without compensatory pattern.
Locomotor training environments and progression
Body weight support and treadmill environment
For LT, the primary retraining for the capacity to walk occurs in the treadmill environment. Barbeau and colleagues first extended the training environment of the animal model studies to clinical application for humans after SCI. He and his colleagues developed an overhead suspension system attached to a body harness worn by the subject while walking on a treadmill . Early experiments assessed the simple effect of body weight support (BWS) on gait in able-bodied subjects . Studies continue today to examine the effect of types of BWS and harness systems . Manufacturers of BWS systems offer varying specifications of control that offer relatively different approximations of the body’s center of mass during walking and the ground reaction forces during loading and propulsion .
The body weight support and treadmill (BWST) environment provides a permissive environment in that it may afford the individual a walking experience that more closely approximates the actual sensorimotor pattern of walking when compared with walking overground. In addition, the BWST environment provides a heightened degree of control and quantification of the sensorimotor experience relative to treadmill speed and BWS. In this environment, the spinal and supraspinal networks for locomotion are functionally reorganized. This new training environment meets the demands of retraining by affording the sensory experience of walking as well as the necessary intensity of repetition and practice . The number of steps that may be achieved in one session in this environment when compared with overground may be a critical component to successful retraining of the nervous system. In some instances, BWS may not be necessary and simply the treadmill may provide an adequate stimulus to practice walking while approximating normal walking speeds .
Many individuals, however, cannot move a limb or do so awkwardly with considerable physical and cognitive effort to achieve a step and often a kinematic incorrect step. These individuals require manual assistance of trainers to (1) provide upright trunk support, (2) facilitate flexion and extension patterns of limb activity, and (3) promote pelvic rotation and weight transfer during loading of the stance limb . Trainers in the clinical context provide assistance as needed to promote activation of muscles within the context of the limb trajectories for the stepping pattern, pelvic movement, and trunk control. Proper ergonomic seats for the trainers at the level of the treadmill with back and leg supports are a necessity for the repetitive task of manual training . A more detailed explanation of manual assistance is provided in several case study reports .
Robotic-assistance was designed to provide an automated system of moving the legs in a stepping pattern on a treadmill using BWS . A robot consisting of an exoskeleton with motors at the hip and knee joints and integrated with the BWST provides a consistent pattern of flexion and extension for stepping. Pelvic and trunk stabilization are provided via straps and supports that minimize or eliminate movement. As a computer-controlled system, BWS and treadmill speed can be varied in conjunction with the spatial-temporal pattern controlled by hip and knee range of motion (ROM) and step length. The robotic system may offer greater consistency of limb trajectories for patterned stepping by eliminating therapist fatigue and thus provides a greater duration of therapy. However, the robotic assistance may provide a more stereotyped and consistent pattern of limb movement than the alternative, manual assistance. In addition, manual assistance allows for real-time decision-making by the therapist to adapt immediately to the patient to achieve proper limb kinematics and the spatio-temporal pattern. Further studies are needed to identify the most efficacious approach to providing the needed assistance during retraining and the potential differential effects in the process of recovery of function.
LT-overground
With the ultimate aim of LT to improve or restore walking ability overground, skills acquired in the treadmill environment must be assessed and translated to overground. The same guiding principles are extended from the treadmill to overground environment. Thus, use of the LT principles has ramifications for how training occurs in the treadmill and overground environments, both in the clinic and at home. For instance, to maintain hip extension while walking with an assistive device introduced overground, the individual must maintain an upright posture and minimize the upper extremity load-bearing on a device. Translation of the training principles beyond the treadmill environment has been developed and studied as an integral component of a locomotor training program by several researchers .
Furthermore, a new generation of assistive devices and options for use of standard devices may develop that are consistent with the goal of recovery of function. For instance, use of a walking or trekking pole (or pair) may promote a more upright posture with minimal balance and weight-bearing support in contrast to the conventional use of a single point cane. The standard four-point walker used for gait training when reversed (with the cross bar positioned behind the patient and open-end forward of the patient) may also promote an upright posture with less weight bearing on the arms.
LT as a rehabilitative strategy has been successful for many people with acute and chronic incomplete SCI; however, varied results are reported . This variability may be caused by the differences among therapists in the relative level of knowledge of the principles underlying retraining of the nervous system, their skill in applying these principles, and the effectiveness of the decisions that are made to progress the recovery as well as the intensity and duration of the intervention. Therapists who are aware of the potential of the spinal networks and sensory signals to modulate muscle activation patterns will have the best chance of optimizing LT for their patients. Implementing LT in the clinic is in the relatively early stages of development, and future efforts should focus on education, training, and establishing standards. Optimizing the protocols for specific patient populations is continually evolving as we simultaneously learn more from ongoing studies. Efficient and effective translation of scientific and clinical evidence to routine clinical practice will take collaborative efforts among scientists, clinicians, and administrators.
Electrical stimulation to activate the neuromuscular system during walking and standing
Although LT induces neuromuscular activation below the lesion by providing the appropriate sensory information back to the nervous system, use of electrical stimulation achieves standing and walking by stimulating muscles that are impaired. Electrical stimulation to the common peroneal nerve to generate a flexor withdrawal response is often used during walking on the treadmill with BWS and overground. The stimulation and flexor response are timed to synchronize with the initiation of the swing phase. Training with swing phase assist using electrical stimulation while walking in the BWST environment has improved walking speed in individuals with AIS C classification and asymmetrical LE function . Similarly, Fung and Barbeau used repeated conditioning of the H-reflex to generate a similar flexor response coordinated with the transition from terminal stance to swing in individuals with incomplete SCI. Electrical stimulation is an alternative means of activating sensory afferents and generating flexor responses within the task of walking. Removal of the electrical stimulation has resulted in sustained improvements and thus represents relatively permanent adaptability by the nervous system in individuals who could already take steps . In addition to use of electrical stimulation to generate a flexor response, electrical stimulation has been also used to assist knee extension during the stance phase of walking . Functional electrical stimulation (FES) has an immediate effect on the gait and serves as a neuroprosthesis. Long-term use of FES-assisted walking has also resulted in some increases in maximal overground walking speed .
Activity-based therapies
In the long term, LT will provide a behavioral therapy that independently supports positive outcomes. It may also serve as a catalyst in tandem with electrical stimulation or pharmacologic agents that when combined form an even better response. Already, researchers have explored the combined use of drugs and LT as well FES and LT to advance recovery . Thus, a continuum of physical rehabilitation interventions may be considered and categorized as activity-based therapies ( Table 1 ). The criteria, activity-based versus compensation, intrinsic versus extrinsic activation of the nervous system, and activation of the nervous system below or above the lesion are used to categorize rehabilitation strategies for mobility.
Rehabilitation for mobility | |||||
---|---|---|---|---|---|
Activity-based | Activity/compensation | Compensation | |||
Intervention | Neuromuscular activity | Intervention | Neuromuscular activity | Intervention | Neuromuscular activity |
Locomotor training for stepping | Intrinsic below lesion level | Walking with FES for individual movements or joints | Intrinsic below lesion level with extrinsic activation for deficits | Walking with reciprocating gait orthosis (RGO) or long-leg braces | None; bracing and mechanical device for deficits |
Ankle foot orthotic use during walking | Intrinsic below lesion level with bracing for deficits | Power or manual wheelchair | None | ||
Ambulation with FES implanted electrodes | Extrinsic below lesion level | ||||
Locomotor training for standing | Intrinsic below lesion level |
|
| Standing frame | Minimal |
Muscle strengthening below lesion level | Intrinsic |
| None | ||
FES cycling | Extrinsic below level of the lesion | ||||
Voluntary cycling | Intrinsic below level of the lesion |
For example, even individuals with clinically complete injury can experience activity-based therapies with manual or robotic assistance and when electrical stimulation is used on muscles below the level of the lesion during stand and step training . In individuals with incomplete injury, this can also occur with many different interventions (ie, FES cycling?) but with a primary aim of facilitating activation of the neuromuscular system below the level of lesion . A further consideration is the activation of the nervous system by extrinsic means, such as electrical stimulation, compared with activation intrinsically by the nervous system. An example of intrinsic activation is the increase in leg extensor muscle EMG activity in response to increasing vertical load while standing in both nondisabled persons and persons with complete and incomplete spinal cord injuries . Also important is a distinction between an outcome that achieves a functional goal compared with recovery of a function. Learning to drive a powered wheelchair with a mouthpiece is considered a “functional goal,” whereas improving the ability to stand or step is an example of functional recovery of the neuromuscular system.
FES may be a tool to improve standing or stepping. If appropriate sensory information is important for retraining, then can current stimulation parameters be effective in relearning or can future FES stimulation paradigms be incorporated with the intrinsic properties of the spinal cord? Whether FES is used through implanted electrodes or surface stimulation to muscles, recovery of function will be established if the stimulation is no longer needed to perform the task. If the FES remains necessary and the nervous system is quiescent in its absence, then it is likely that FES under those conditions is not contributing to the recovery of function. In this instance, FES is acting in a compensatory role as a neuroprosthesis. Further studies are needed to understand the most optimal approach to retraining the nervous system and to determine the most appropriate use of electrical stimulation in severely impaired individuals.
Strengthening muscles above the level of the lesion is a compensation-based therapy; however, activating and strengthening muscles below the level of the lesion is an activity-based therapy. Whether intrinsically driving or extrinsically activating muscles below the level of the lesion , neuromuscular adaptations result in greater voluntary torque and increased rate of torque production in persons with incomplete and complete SCI. Persistence of such benefits is dependent on the capacity of the individual to continue neural activation within the context of daily function or remain dependent on extrinsic input for activation. Whether FES cycling promotes recovery of muscle activation specific to the task of walking has yet to be determined.
Outcome measures: functional goals and recovery of function
The outcome measures studied are critical to interpreting the effect and clinical meaningfulness of compensation-based and activity-based therapies, including LT. Because physical rehabilitation as an agent for recovery is a new perspective, comparable outcome measures need to be developed that measure recovery. Current clinical measures address the ability to perform a task as a functional goal but may not specifically address recovery of function . A task may be performed using a compensatory strategy or via recovery of the neuromuscular control specific to that task. Achievement of the compensatory behavior is consistent with gaining a new ability to accomplish a goal (eg, use of forearm crutches to bear partial weight while using a head–hip strategy to advance a pair of long-leg braces forward for ambulation), whereas retraining and recovery of function entails regaining the specific function associated with the task (eg, activating limb extension and support for standing and flexor activity for swing).
Clinical outcome measures target achieving the task goal, for example, walking performance . Outcome measures include walking speed (m/s) at self-selected and fastest speeds (treadmill or overground), time to traverse prescribed distances (50 m walk test), endurance–distance walked in set time, amount of assistance required (ie, braces, assistive device, or physical assistance) (WISCI II) , ability to negotiate obstacles, and balance ability. The assessment of clinical meaningfulness of an outcome is particularly important. Gait speed, as a continuous variable, may provide the most meaningful outcome relative to the achievement of speeds standard for adults (1.2 m/s) and required for community ambulation for safe street crossing at traffic lights . Percent changes in gait speed may be statistically significant but may portray an inflated view of meaningful value. For example, when a 100% improvement in gait speed reflects a change from 0.05 m/s to 0.1 m/s the change may be negligible to the observer and, unfortunately, of little consequence as a behavioral gain. However, a 100% improvement in gait speed from 0.4 to 0.8 m/s may be a meaningful change and reflect a functional shift from household to community ambulator. For gait speed to be a measure of recovery, the variability of the measure and the clinical meaning of improved speed should be established, as well as consider the influence of different assistive devices.
Walking recovery, regaining the specific functions that afford walking capacity, will require new measures reflecting interim, yet, progressive changes across training time. Instruments that measure specific function for walking entail the capacity to produce a reciprocal stepping pattern, balance during propulsion, and adaptation to the environment . A single measure is unlikely to reflect the recovery of function of an individual or subpopulations. Composite outcome measures or targeted measures for specific phases of recovery may be more reflective of recovery. Assessing the neuromuscular capacity below the level of lesion may provide a more objective and sensitive assessment during recovery , The presence of clonus and spasticity may also now be considered as a positive indication that neural networks are active and have the potential for functional reorganization rather than as a consequence of loss of supraspinal input that prohibits recovery of motor function that should be eliminated by pharmacologic or surgical interventions .
As the treadmill environment affords a permissive and controlled environment for retraining, it may also offer an environment for assessing the nervous systems’ capacity to step, balance, and adapt as well as assessing the recovery of walking specific functions. In this environment, for instance, BWS required to maintain lower limb extension or upright posture may be titrated and quantified indicating increments of capacity and recovery from an initial evaluation through a training process. Incremental gains observed in the training environment are likely to be more sensitive and informative of capacity/recovery when compared with the gains observed by routine clinical measures overground (eg, self-selected gait speed). Thresholds of recovery specific to walking capacity may ultimately correlate with clinical gains overground. For example, a patient demonstrated no significant gains in gait speed overground BWS, and manual assistance had decreased and been eliminated while walking in the BWS environment . Thus, the continual decline in BWS and manual assistance relative to improved control of the trunk, upright posture, and limb flexion during walking can identify changes in recovery before the current outcomes. Other means of measurement may afford greater quantification in this environment and are a critical area of ongoing and future development. Secondary and tertiary benefits of therapy on the ability to perform activities of daily living, health, and quality of life may also be considered as outcome measures .
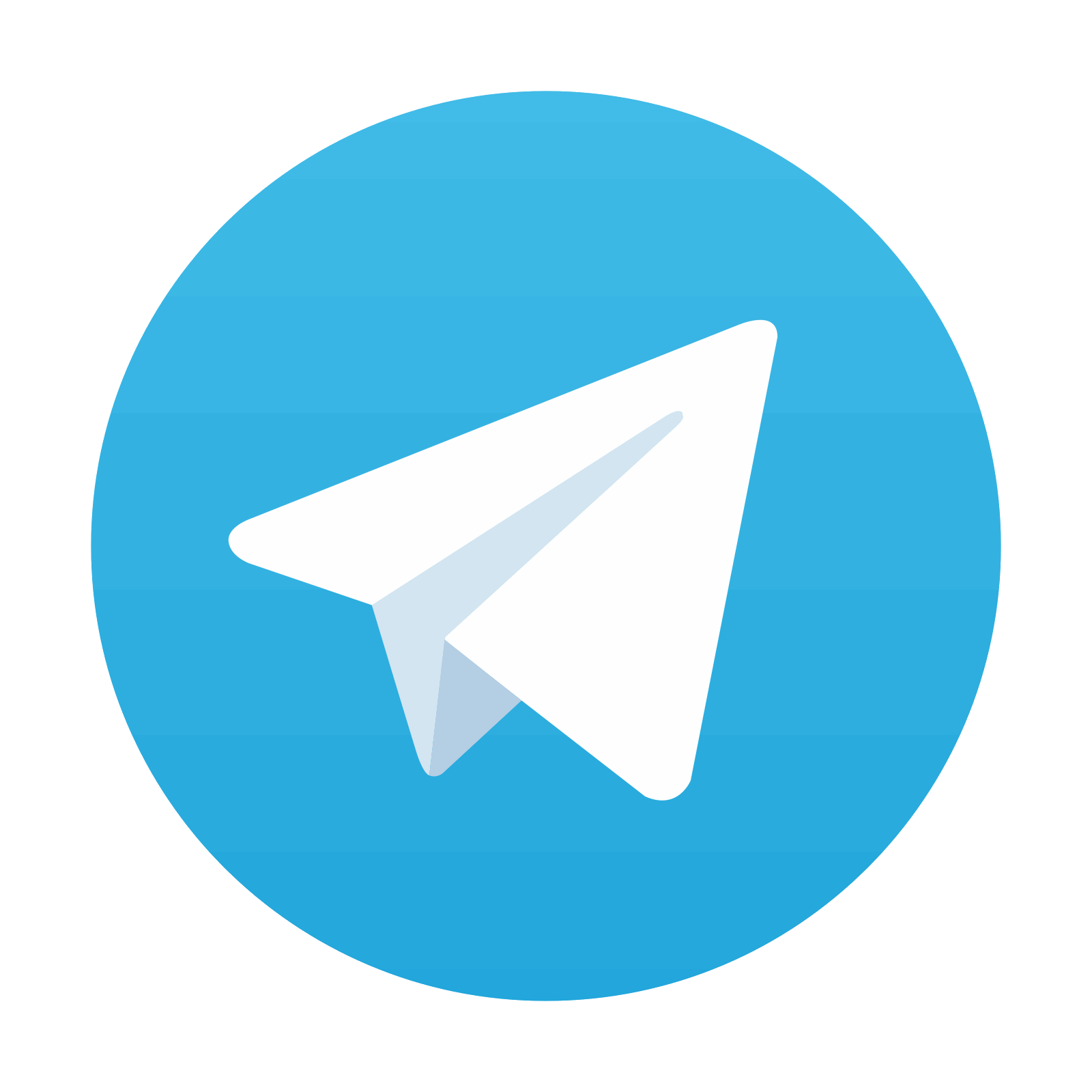
Stay updated, free articles. Join our Telegram channel

Full access? Get Clinical Tree
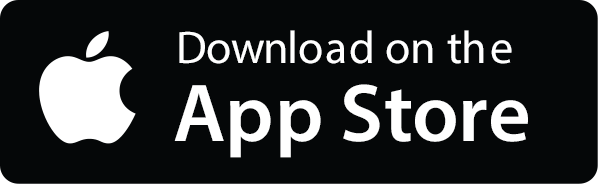
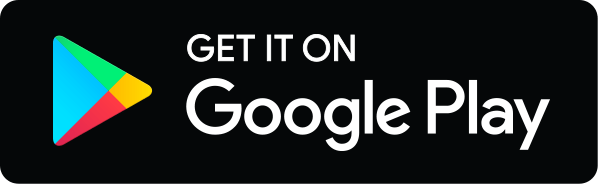