FIGURE 7-1 Typical age (and range) of development of the secondary ossification centers of the epiphyses in the (A) upper extremity and (B) lower extremity.
FIGURE 7-2 Typical age (and range) of closure of physes in the (A) upper extremity and (B) lower extremity.
Microscopic Structure
Physis is highly organized, yet dynamic structure that consists of chondrocytes undergoing proliferation, differentiation, and formation of complex extracellular matrix. The extracellular matrix is composed of type II collagen fiber network, aggrecans, and noncollagenous proteins, such as cartilage oligomeric protein and matrilin-3. Type IX and XI collagens are minor collagens found in the physis. Type X collagen is also found in the physis; however, its synthesis is limited to the hypertrophic zone and is a distinguishing feature of hypertrophic chondrocyte.
Understanding of physeal injuries requires knowledge of normal physeal morphology.135 Histologically, the physis is divided into four zones oriented from the epiphysis to the metaphysis: Germinal (reserve), proliferative, hypertrophic, and provisional calcification (Fig. 7-3). The proliferative zone is the location of cellular proliferation, whereas the hypertrophic and provisional calcification zones are characterized by extracellular matrix production, cellular hypertrophy, apoptosis, extracellular matrix calcification, and vascular invasion of the lacunae of the terminal hypertrophic chondrocytes. Collagen fiber orientation is horizontal in the germinal zone whereas it is vertical in the proliferative and hypertrophic zones, in line with growth and columnar arrangement of cells.8 Collagen content is lower in the proliferative and hypertrophic zones compared with the germinal zone. The differences in the collagen content and fiber orientation of different physeal zones have important implications in the mechanical behavior of each zone to mechanical loading.9 For instance, greater strains are observed in the proliferative and hypertrophic zones compared with the germinal zone following compression loading.
FIGURE 7-3 Schematic diagram of the organization of the physis. Four zones are illustrated: The germinal, proliferative, hypertrophic, and provisional calcification (or enchondral ossification) layers. Note also the groove of Ranvier and the perichondral ring of LaCroix.
The peripheral margin of the physis comprises two specialized areas important to the mechanical integrity and peripheral growth of the physis (Fig. 7-3). The zone (or groove) of Ranvier is a triangular microscopic structure at the periphery of the physis, containing fibroblasts, chondroblasts, and osteoblasts. It is responsible for peripheral growth of the physis. The perichondral ring of LaCroix is a fibrous structure overlying the zone of Ranvier, connecting the metaphyseal periosteum and cartilaginous epiphysis, and has the important mechanical function of stabilizing the epiphysis to the metaphysis.
The epiphysis and secondary ossific nucleus must receive blood supply for viability.155 Dale and Harris46 identified two types of blood supply to the epiphysis (Fig. 7-4). Type A epiphyses (such as the proximal humeral and proximal femoral epiphyses) are nearly completely covered with articular cartilage; therefore, most of the blood supply to the epiphysis must enter from the perichondrium in a distal to proximal direction. The blood supply to these epiphyses may be easily compromised by epiphyseal separation. A complete disruption of the epiphyseal vasculature, however, may not produce an extensive ischemic damage to the physis if the metaphyseal vasculature is intact.87 The studies using multiphoton microscopy also suggest that growth plate nutrition is not unidirectional from the epiphysis to the metaphysis as traditionally believed but is contributed by the epiphyseal, metaphyseal, and circumferential perichondrial vasculature.53,161 Type B epiphyses (such as the proximal and distal tibia and the distal radius) have only a portion of their surface covered with articular cartilage and are theoretically less susceptible to devascularization from epiphyseal separation.
FIGURE 7-4 Classification of epiphyseal blood supply according to Dale and Harris. A: Type A epiphyses are nearly completely covered by articular cartilage. Blood supply must enter via the perichondrium. This blood supply is susceptible to disruption by epiphyseal separation. The proximal femur and proximal humerus are examples of type A epiphyses. B: Type B epiphyses are only partially covered by articular cartilage. Such epiphyses are more resistant to blood supply impairment by epiphyseal separation. The distal femur, proximal and distal tibia, and distal radius are clinical examples of type B epiphyses.
Regulation of Growth
Various systemic (hormonal) and local (paracrine) factors, as well as the mechanical factors, regulate and influence the longitudinal growth.115 Systemic factors that influence physeal function include growth and thyroid hormones, androgen, estrogen, vitamin D, and glucocorticoids. Estrogen and not androgen controls the growth spurt and normal physeal closure at skeletal maturity in both sexes.149 Local factors that influence chondrocyte proliferation and differentiation include parathyroid hormone-related protein, Indian hedgehog protein, transforming growth factor-B, insulin-like growth factor-1, and fibroblast growth factor. In a physis, chondrocyte hypertrophy contributes most to the longitudinal growth followed by extracellular matrix production and cell division.163 The physes with more rapid growth, such as the proximal tibial physis in comparison to the proximal radial physis, have a larger increase in cell size. Experimental studies show that static, sustained loading decreases chondrocyte proliferation, cell height, and the thickness of the hypertrophic zone.158 Hueter–Volkmann law states that abnormal compression inhibits growth whereas distraction stimulates it. A varying degree of dynamic physiologic loading, however, has not been shown to significantly alter longitudinal bone growth.114
Contributions to Longitudinal Growth and Maturation Characteristics of Selected Physes
Growth of long bones is more complex than simple elongation occurring at their ends. However, as a generality, the physes at the end of long bones contribute known average lengths in percentage of total bone growth and percentage contributions to the total length between two physes at either end of a long bone. This information has come from observations of longitudinal growth by a number of authors.11–13,64,71,102 Knowledge of this information is paramount for the surgeon managing physeal injuries to long bones. Figure 7-5 outlines the generally accepted percentage of longitudinal growth contribution of pairs of physes for each long bone in the upper and lower extremities. Table 7-1 outlines the average amount of growth in millimeters per year of skeletal growth contributed by the same physes mentioned above. These are estimations only, and growth tables should be consulted when more specific information is required.12,13,64,71,102
TABLE 7-1 Average Growth per Year (in mm) of Specific Physes of the Upper and Lower Extremitiesa
FIGURE 7-5 Approximate percentage of longitudinal growth provided by the proximal and distal physes for each long bone in the upper (A) and lower (B) extremities.
Mechanical Features of the Physis and Patterns of Injury
An understanding of the microscopic characteristics of the physeal zones permits an understanding of the theoretical line of least resistance (and hence fracture) within the physis. The germinal and proliferative zones are characterized by an abundance of extracellular matrix, whereas the hypertrophic and provisional calcification zones are primarily cell hypertrophy, apoptosis, and vascular channels. As a consequence, fracture lines can be predicted to pass through the hypertrophic and provisional calcification zones, a finding that Salter and Harris reported in their experimental investigation in rats.138 Theoretically, Salter–Harris types I and II fractures should involve these zones only, not affecting the germinal and proliferative zones, and thus should be at lower risk for subsequent growth disturbance. However, types III and IV physeal fractures traverse the entire physis, including the germinal and proliferative zones. In addition, displacement between bone fragments containing portions of the physis may occur. Consequently, growth disturbance is more likely from type III or IV injuries.
Not surprisingly, mechanical and clinical studies of microscopic fracture patterns have demonstrated that fracture lines through the physeal layers are more complex than this simplistic view, and often undulate through the various zones.28,63,80,112,148,159 Smith et al.148 reported a Salter–Harris type I fracture of the distal tibia examined microscopically after associated traumatic lower leg amputation. In this high-energy injury, they found that the fracture line involved all four layers of the physis, in part because of the relatively straight plane of fracture and the undulations of the physis. Bright et al.28 in a study of experimentally induced physeal fractures in immature rats, found that not only was the fracture line usually complex, involving all four layers of the physis, but also that the physis contained a number of horizontal “cracks” separate from the fracture itself. They also observed a statistically significant lower force required to produce a physeal fracture in male and prepubescent animals, which might have clinical relevance to the epidemiologic aspects of physeal fractures (see “Epidemiology”). The rate, direction, and magnitude of force are also factors that contribute to the histologic pattern of physeal fractures. Moen and Pelker,112 in an experimental study in calves, found that compression forces produced fractures in the zone of provisional calcification and metaphysis, shear caused fractures in the proliferative and hypertrophic zones, and torque produced fracture lines involving all four layers of the physis. Finally, the energy of injury is a factor in the extent of physeal injury. Distal femoral physeal fractures are a good example of the overriding significance of the energy of injury in potential for subsequent growth disturbance. High-energy mechanisms of injury are frequent in this region, and the risk of subsequent growth disturbance is high.99,134
Our current understanding of how a bone bridge forms following a physeal injury is limited. The experimental studies show a sequence of inflammatory, fibrogenic, and osteogenic responses in the time course of bone bridge formation following a drill hole injury to the proximal tibial physis in a rat model.165 More in-depth study using microarray analysis showed that several molecular pathways including those involved with skeletal development, osteoblast differentiation, BMP signaling, and Wnt signaling are involved in the bone bridge formation.100 A better understanding of mechanisms involved with the bone bridge formation may lead to new treatments that can prevent this complication.
PHYSEAL INJURIES
Etiology of Physeal Injuries
Physes can be injured in many ways, both obvious and subtle. Obviously, the most frequent mechanism of injury is fracture. Most commonly, physeal injury is direct, with a fracture involving the physis itself. Occasionally, physeal injury from trauma is associated with a fracture else wherein the limb segment, either as a result of ischemia125 or perhaps compression1,10,25,76,107,113,157 (see discussion of Salter–Harris type V physeal fractures below). Other mechanisms of injuries to the physes include infection,19,23,91,123 disruption by tumor, cysts,150 and tumor-like disorders, vascular insult,125 repetitive stress,7,26,39,40,98,168 irradiation,34,136 and other rare etiologies.18,32,38,141,168
Infection
Long bone osteomyelitis or septic arthritis (particularly of the shoulder, hip, and knee) can cause physeal damage resulting in either physeal growth disturbance or frank growth arrest.14,19,23,52,65,78,88,91,123 These septic injuries may be further complicated by joint disruption resulting from associated epiphyseal destruction, articular cartilage damage, and capsular adhesions, particularly in the hip and shoulder.
Multifocal septic arrests can produce significant deformity requiring multiple surgical procedures. The most common causes are fulminant neonatal sepsis, particularly in premature infants or those with neonatal sepsis associated with maternal diabetes, and multiple septic arrests associated with meningococcemia (Fig. 7-6). In the latter case, physeal damage may also result from the cardiovascular collapse and disseminated intravascular coagulation known as purpura fulminans.14,65,78,88
FIGURE 7-6 Standing anteroposterior lower extremity radiograph of a 12-year-old boy with multifocal physeal disturbance from purpura fulminans associated with meningococcemia. Radiograph abnormalities are present in the left proximal femur; both distal femoral epiphyses, including partial arrest of the left distal femoral physis; and both distal tibial epiphyses. The patient also has digital amputations and extensive soft tissue scarring resulting from this septic event.
Tumor
Both malignant and benign tumors and tumor-like disorders can disrupt normal physeal architecture, resulting in direct physeal destruction. In the case of malignant tumors, the extent of growth lost as the result of local irradiation or limb salvage surgery must be taken into consideration in planning and recommending the therapeutic reconstruction to be undertaken.
Benign tumors and tumor-like conditions can result in destruction of all or part of a physis. Examples include enchondromata, either isolated or multiple (Ollier disease) (Fig. 7-7), and unicameral bone cysts.150 Growth disturbance as a consequence of physeal damage from these disorders generally cannot be corrected by surgical physeal arrest resection (see “Physeal Arrests”), and other treatment strategies must be adopted as clinically indicated.
FIGURE 7-7 Valgus deformity of the distal femur associated with the presence of an enchondroma of the distal lateral femur involving the lateral physis.
Vascular Insult
Known vascular insult is a rare cause of physeal injury.125 Partial or complete growth arrests can occur from a pure vascular injury to an extremity (Fig. 7-8). Unrecognized vascular insult may represent the mechanism of subsequent growth disturbance after an injury in an adjacent part of a limb and may play a role in Salter–Harris type V injuries; the most common location for this is the tibial tubercle after femoral shaft or distal femoral physeal fractures. In addition, ischemia may be the cause of physeal damage associated with purpura fulminans.14,65,78,88
FIGURE 7-8 Physeal injury from presumed vascular insult. A: The patient’s leg was caught under heavy pipes rolling off a rack, resulting in stripping of the soft tissues from the distal thigh, open comminuted fracture of the distal femur, and popliteal artery injury. B: In follow-up, after arterial and soft tissue reconstruction, the patient has physeal growth arrests of the distal femur and proximal tibia. The mechanism of injury to the proximal tibial physis was presumed to be vascular because of the associated femoral artery injury.
Repetitive Stress
Repetitious physical activities in skeletally immature individuals can result in physeal stress–fracture equivalents.7,39,40 The most common location for such injuries is in the distal radius or ulna, as seen in competitive gymnasts (Fig. 7-9); the proximal tibia, as in running and kicking sports such as soccer (Fig. 7-10); and the proximal humerus, as in baseball pitchers.39 These injuries should be managed by rest, judicious resumption of activities, and longitudinal observation to monitor for potential physeal growth disturbance.
FIGURE 7-9 Stress injury of the distal radius and ulna in both wrists of a competitive gymnast. There was no history of specific injury. The wrists were tender to touch. Note distal radial and ulnar physeal widening and irregularity.
FIGURE 7-10 Stress injury of the proximal tibia in an elite soccer player. A: Anteroposterior radiograph film demonstrates subtle proximal tibial physeal widening. B: Lateral radiograph shows widening, a metaphyseal Thurston–Holland fragment, and some posterior displacement of the proximal epiphysis. C: Significant radiograph improvement noted after discontinuing athletic activities for 3 months.
Miscellaneous (Irradiation, Thermal Injury, Electrical, Unrecognized)
Rare causes of physeal injury, usually recognized from consequent growth disturbance, include irradiation (Fig. 7-11)34,136; thermal injury, especially phalangeal physeal injury from frostbite (Fig. 7-12)32,38; burns; and electrical injuries. A recent report noted progressive genu valgum associated with obesity and theorized that repetitive microtrauma superimposed on genetic factors might play a role in growth disturbance.168 On other rare occasions, physeal growth disturbance noted on clinical findings and radiographs has no identifiable cause. Presumably, such events represent unrecognized trauma, infection, or vascular insult involving the physis.
FIGURE 7-11 Proximal tibial physeal growth disturbance with angular deformity after irradiation for Ewing sarcoma.
FIGURE 7-12 Premature closure of the distal phalangeal physes after a frostbite injury to the digits.
Historical Review of Physeal Fractures
Physeal fractures have been recognized as unique since ancient times. Hippocrates is credited with the first written account of this injury. Poland (see “Classification of Physeal Fractures”) reviewed accounts of physeal injuries in his 1898 book, Traumatic Separation of the Epiphysis.132 Poland is also credited with the first classification of the patterns of physeal fracture, and the publication of his text closely followed Roentgen’s discovery of radiographs in 1895.
Classification of Physeal Fractures
Poland132 proposed the first classification of physeal fractures in 1898. Modifications to Poland’s original scheme have been proposed by a number of authors,2–5,46,49,101,117,119,126,127,130,138 including Aitken,4 Salter and Harris,138 Ogden et al.119,107 and Peterson.126,127 Classifications of physeal fractures are important because they alert the practitioner to potentially subtle radiographic fracture patterns, can be of prognostic significance with respect to growth disturbance potential, and guide general treatment principles based on that risk and associated joint disruption. To some extent, fracture pattern provides some insight into mechanism of injury and the extent of potential physeal microscopic injury (“Normal Physeal Anatomy” and “Mechanical Features of the Physis and Patterns of Injury”).
Currently, the Salter–Harris classification, first published in 1963,138 is firmly entrenched in the literature and most orthopedists’ minds. Therefore, evolution and specifics of the nature of physeal fractures of the various classification schemes are discussed relative to the Salter–Harris classification. The reader also should be aware of some deficiencies in that classification, as pointed out by Peterson.126–128
Poland Classification of Physeal Fractures
Poland’s classification, published in 1898,132 consisted of four types of physeal fractures (Fig. 7-13). Types I, II, and III were the foundation of the Salter–Harris classification, as described below. Poland’s type IV fracture was effectively a T-condylar fracture of the epiphysis and physis.
FIGURE 7-13 Poland classification of physeal fractures compared to the Salter–Harris classification. Poland type I: Epiphyseal separation without metaphyseal fragment, or extension into the epiphysis. Poland type II: Physeal fracture line extends into the metaphysis. Poland type III: Fracture extends from the articular surface to the physis and continues peripherally through the physis. Poland type IV: T-condylar fracture of the epiphysis and physis.
Aitken Classification of Physeal Fractures
Aitken4 in 1936 included three patterns of physeal fracture in his classification (Fig. 7-14). His type I corresponded to Poland and Salter–Harris type II fractures, his type II to Poland and Salter–Harris type III fractures, and his type III was an intra-articular transphyseal metaphyseal–epiphyseal fracture equivalent to a Salter–Harris type IV fracture.
FIGURE 7-14 Aitken classification of physeal fractures: Types I, II, and III. Type III is equivalent of Salter–Harris type IV.
Salter–Harris Classification of Physeal Fractures
Salter and Harris published their commonly used five-part classification of physeal injuries in 1963.138 The first four types were adopted from Poland (types I, II, and III) and Aitken (Aitken type III became Salter–Harris type IV) (Fig. 7-15). Salter and Harris added a fifth type, which they postulated was an unrecognized compression injury characterized by normal radiographs and late physeal closure. Peterson and Burkhart challenged the existence of true type V injuries,128 but other authors have subsequently documented its existence in some form.1,10,17,25,74,76,86,128,157 Because we believe that delayed physeal closure can occur after some occult injuries, we have chosen to retain this type of injury in our preferred classification scheme.
FIGURE 7-15 Salter–Harris classification of physeal fractures. In Salter–Harris type I fractures, the fracture line is entirely within the physis, referred to by Poland as type I. In Salter–Harris type II fractures, the fracture line extends from the physis into the metaphysis; described by Poland as type II and Aitken as type I. In Salter–Harris type III fractures, the fracture enters the epiphysis from the physis and almost always exits the articular surface. Poland described this injury as type III and Aitken as type II. In Salter–Harris type IV, the fracture extends across the physis from the articular surface and epiphysis, to exit in the margin of the metaphysis. Aitken described this as a type III injury in his classification. Salter–Harris type V fractures were described by Salter and Harris as a crush injury to the physis with initially normal radiographs with late identification of premature physeal closure.
Type I. Salter–Harris type I injuries are characterized by a transphyseal plane of injury, with no bony fracture line through either the metaphysis or the epiphysis. Radiographs of undisplaced type I physeal fractures, therefore, are normal except for associated soft tissue swelling, making careful patient examination particularly important in this injury. In the Olmstead County Survey of physeal fractures,129 type I fractures occurred most frequently in the phalanges, metacarpals, distal tibia, and distal ulna. Epiphyseal separations in infants occur most commonly in the proximal humerus, distal humerus, and proximal femur. If an urgency to make the diagnosis is deemed necessary for patients suspected of having a type I injury, further imaging by ultrasound, magnetic resonance imaging (MRI),36,44,79,130,147 or intraoperative arthrography may be helpful.6,67,105 Stress radiographs to document displacement are generally unnecessary and probably unwise. Ultrasound is particularly helpful for assessing epiphyseal separations in infants (especially in the proximal femur and elbow regions) without the need for sedation, anesthetic, or invasive procedure.30,48,50,73,140
The fracture line of type I injuries is usually in the zone of hypertrophy of the physis, as the path of least resistance during the propagation of the injury (see “Normal Physeal Anatomy”) (Fig. 7-16). As a consequence, in theory, the essential resting and proliferative zones are relatively spared, and, assuming that there is no vascular insult to these zones as a consequence of the injury, subsequent growth disturbance is relatively uncommon. As discussed above, however, studies have shown this to be a simplistic view of the fracture line through a physis, and that, because of uneven loading and macroscopic undulations in the physis, any zone of the physis can be affected by the fracture line.27,80,112,137,141,148
FIGURE 7-16 Scheme of theoretic fracture plane of Salter–Harris type I fractures. Because the hypertrophic zone is the weakest zone structurally, separation should occur at this level. Experimental and clinical studies have confirmed that the fracture plane is more complex than this concept and frequently involves other physeal zones as well.
Because the articular surface and, at least in theory, the germinal and proliferative layers of the physis are not displaced, the general principles of fracture management are to secure a gentle and adequate reduction of the epiphysis on the metaphysis and stabilize the fragments as needed.
Type II. Type II injuries have physeal and metaphyseal components; the fracture line extends from the physeal margin peripherally across a variable portion of the physis and exits into the metaphysis at the opposite end of the fracture (Fig. 7-17). The epiphyseal fragment thus comprises all of the epiphysis and some portion of the peripheral metaphysis (the Thurston–Holland fragment or sign). The physeal portion of this fracture has microscopic characteristics similar to those of type I injuries, but the fracture line exits the physis to enter the metaphysis (i.e., away from the germinal and proliferative layers) at one margin. Similar to type I injuries, these fractures should have a limited propensity to subsequent growth disturbance as a consequence of direct physeal injury. However, the metaphyseal “spike” of the diaphyseal/metaphyseal fragment may be driven into the physis of the epiphyseal fragment, which can damage the physis (Fig. 7-18). Similar to type I injuries, the articular surface is not affected and the general principles of fracture management are effectively the same.
FIGURE 7-17 Fracture plane of Salter–Harris type II fractures. The fracture extends from the physis into the periphery of the metaphysis.
FIGURE 7-18 Potential mechanism of physeal arrest development after Salter–Harris type II fracture of the distal radius. A: Dorsally displaced type II fracture of the distal radius. Note the evidence of impaction of the epiphyseal fragment (with the physis) by the dorsal margin of the proximal fragment metaphysis. B: One year later, there is radiographic evidence of physeal arrest formation in the distal radial physis.
Type III. Salter–Harris type III fractures begin in the epiphysis (with only rare exception) as a fracture through the articular surface and extend vertically toward the physis. The fracture then courses peripherally through the physis (Fig. 7-19). There are two fracture fragments: A small fragment consisting of a portion of the epiphysis and physis, and a large fragment consisting the remaining epiphysis and long bone. This fracture pattern is important for two main reasons: The articular surface is involved (Fig. 7-20) and the fracture line involves the germinal and proliferative layers of the physis. In addition, type III injuries are often associated with high-energy or compression mechanisms of injury, which imply greater potential disruption of the physis and higher risk of subsequent growth disturbance. Anatomic reduction (usually open) and stabilization are required to restore the articular surface and to minimize the potential for growth disturbance.
FIGURE 7-19 Scheme of fracture plane in Salter–Harris type III fractures. The fracture plane extends from the physis into the epiphysis and articular surface. “Extra-articular” type III fractures in which the articular surface is intact have been reported but are quite rare.
FIGURE 7-20 A: Salter–Harris type III fracture of the distal femur. B: Fixation with cannulated screws.
On occasion, particularly in the distal femur and the distal humerus, high-energy injuries produce either a T-condylar or other complex pattern of injury, with at least three fragments, resulting in a combination of physeal and epiphyseal injuries (Fig. 7-21).
FIGURE 7-21 Complex fracture of the distal femur. There is a Salter–Harris type II fracture of the distal femoral physis. In addition, there is an additional coronal plane epiphyseal fracture of the major portion of the lateral femoral condyle, not involving the physis, which was not recognized at the time of initial treatment. The type II component was treated by closed reduction and cross-pinning. The epiphyseal fracture was treated separately and subsequently by open reduction and headless screw fixation. A: Initial anteroposterior radiograph showing what appears to be simple Salter–Harris type II fracture of the distal femur. B: Lateral radiograph after reduction appears acceptable; however, careful review demonstrates the coronal plane, intra-articular fracture of the lateral condyle. C: CT scan demonstrates the epiphyseal fracture of the lateral femoral condyle. D, E: Radiograph appearance after healing of the fractures. Patient was asymptomatic and recovered full knee motion. In follow-up, the patient developed symmetric distal physeal closure not requiring further treatment.
Type IV. Type IV fractures are effectively vertical shear fractures, extending from the articular surface to the metaphysis (Fig. 7-22A). These fractures are important because they disrupt the articular surface, violate all the physeal layers in crossing from the epiphysis to the metaphysis, and, with displacement, may result in metaphyseal–epiphyseal cross union (Fig. 7-22B).41,62 The latter occurrence almost invariably results in subsequent growth disturbance. This fracture pattern is frequent around the medial malleolus, but may occur in other epiphyses. Lateral condylar fractures of the distal humerus and intra-articular two-part triplane fractures of the distal tibia may be thought of as complex Salter–Harris type IV fractures.
FIGURE 7-22 Scheme of the Salter–Harris type IV fracture. A: The fracture line extends across the physis from the epiphysis and articular surface into the peripheral metaphysis. B: Displacement of the fragments can lead to horizontal apposition (and cross union) of the epiphyseal and metaphyseal bones.
General treatment principles include obtaining anatomic reduction and adequate stabilization to restore the articular surface and prevent metaphyseal–epiphyseal cross union.
Type V. The type V fracture described by Salter and Harris was not described by Poland or Aitken. Salter and Harris postulated that type V fractures represented unrecognized compression injuries with normal initial radiographs that later produced premature physeal closure. The existence of true type V injuries was questioned by Peterson128 and subsequently became a subject of debate.1,10,17,25,74,76,86,157 We believe that delayed physeal closure clearly occurs. The most common example of such an injury is closure of the tibial tubercle, often with the development of recurvatum deformity of the proximal tibia, after fractures of the femur or distal femoral epiphysis (Fig. 7-23).25,74,76 Although the mechanism of such injuries may be unclear (perhaps vascular rather than compression trauma), the traditionally held view that such injuries occurred as a result of inadvertent direct injury during the insertion of proximal tibial skeletal traction pins has been unequivocally discounted in some cases.25,74,76 Other locations and case reports of late physeal closure after extremity injury and apparently normal initial radiographs exist in the literature.1,10,17,19,111,157 By definition, this pattern of injury is unrecognized on initial radiographs. Undoubtedly, more sophisticated imaging of injured extremities (such as with MRI) will identify physeal injuries in the presence of normal plain radiographs (Fig. 7-24). Although the mechanism of injury in type V injuries may be in dispute, in our opinion, the existence of such injuries is not.
FIGURE 7-23 Posttraumatic closure of the anterior proximal tibial physis after displaced Salter–Harris type II fracture of the distal femoral physis. A: Lateral radiographs after reduction. No injury to the proximal tibia was noted at the time of treatment of the distal femoral injury. B: At follow-up, distal femoral physeal growth disturbance with flexion deformity is apparent. C: At skeletal maturity, proximal tibial extension deformity with sclerosis of the tibial tubercle area is evident, suggestive of arrest in this area. The patient has undergone a distal femoral extension osteotomy.
FIGURE 7-24 MRI of patient after injury with normal radiographs. MRI clearly documents the presence of a Salter–Harris type II fracture of the distal femur.
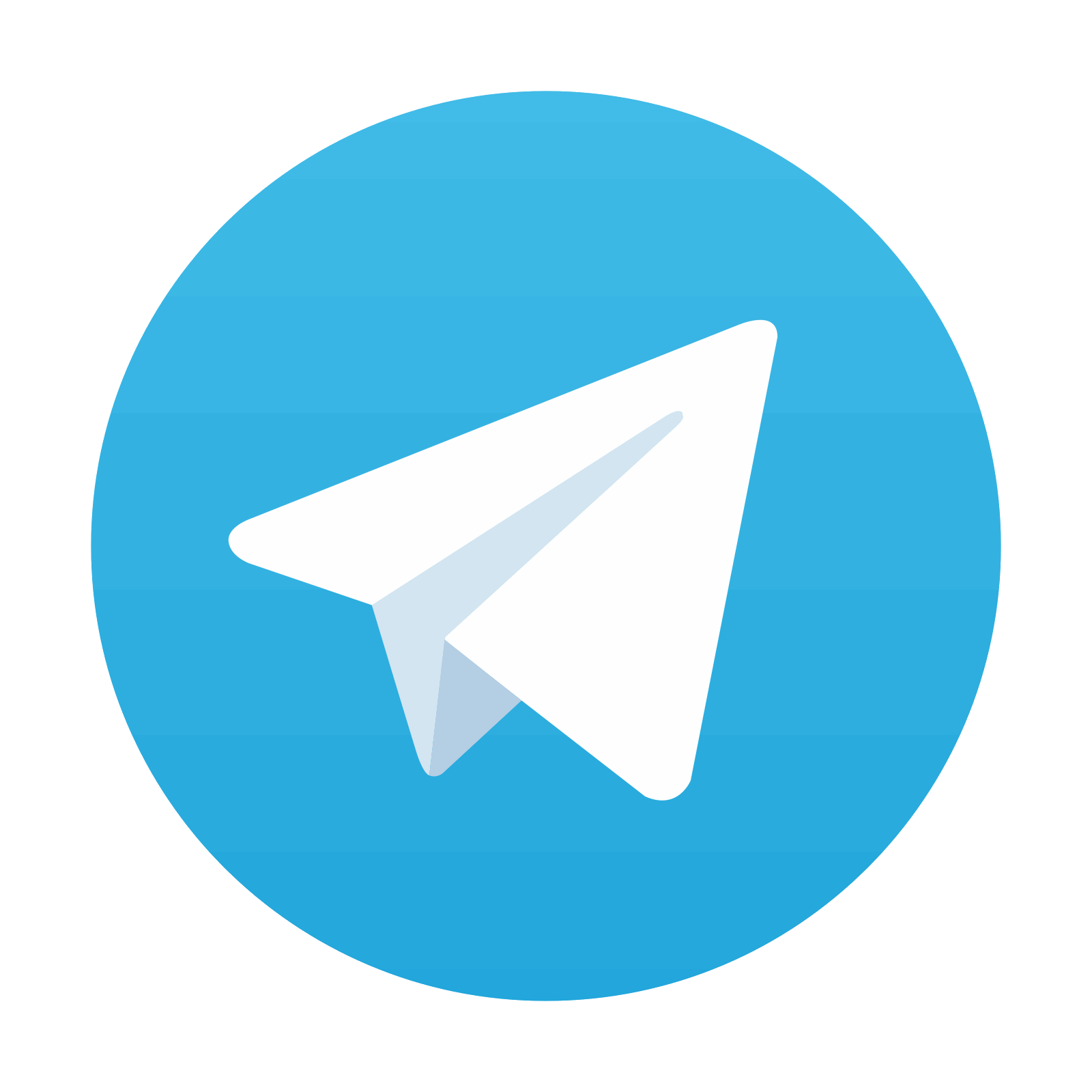
Stay updated, free articles. Join our Telegram channel

Full access? Get Clinical Tree
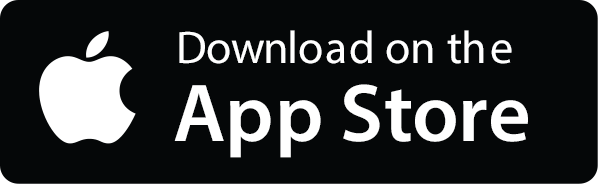
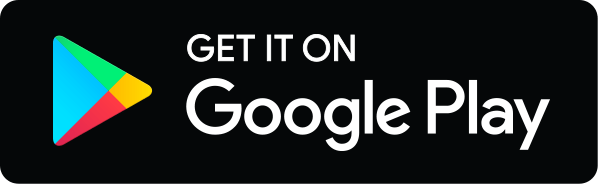