2 | Pathophysiology and Classification of Osteomyelitis |
Introduction
Postoperative and posttraumatic infections of bones, soft tissues, and joints still present a large problem and are among the most serious complications despite rapid advances in the field of medicine.
Osteomyelitis basically means inflammation of bone and bone-marrow components. This could be of bacterial origin, but may also result from tuberculosis or syphilis and, depending on the immune status of the host, may even be of fungal or parasitic (echinococci, toxoplasma) origin.
The infectious organisms reach the bones either through the bloodstream (endogenous) or by direct colonization (exogenous). The bacteria reach the bones through skin lesions, soft-tissue necroses, or operation wounds. The term osteomyelitis has achieved worldwide acceptance.
From a pathologic-anatomical point of view, osteomyelitis can be differentiated into a focal form—referred to as a bone abscess—and one with diffuse spread.
Pathophysiology of Osteomyelitis
Bone provides supportive tissue consisting of an organic matrix and cellular elements. Because of the morphology of bone structure, osteomyelitis is not limited to the bone surface, but extends to all components of the infected osseous area. Osteocytes are distributed throughout the bone matrix and are connected to neighboring cells and blood vessels through cytodendrites in tiny canals (Fig. 2.1).
This canal system is of central importance for metabolic processes. Longitudinal vascular canals (haversian canals) run through the center of each bone lamella, and Volkmann’s canals run through the compact layer. These two systems are interconnected, thus when bone infection occurs the inflammatory process usually affects all osseous components. Moreover, inflammatory resorptive processes create wide connecting canals between the individual osteons.
Surgical implantation of foreign materials, for example plates, nails, screws, and fixator pins, as well as joint prostheses and bone cement, is associated with a higher infection rate than operative procedures in which no foreign material is implanted (Southwood et al. 1985, Gristina 1987).
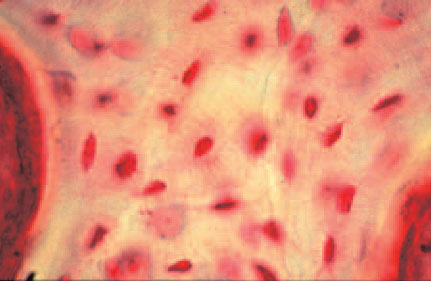
Fig. 2.1 Osteocytes with cytoplasmatic projections.
After implantation, a “battle” evolves for colonization of the surface of the foreign body in which cell or tissue integration and bacterial adhesion simultaneously compete. This is referred to as the “race for the surface” (Gristina 1987, 1990).
The bacteria stick to the surfaces in order to survive, whereby approximately 90% of the physiologic bacteria survive in the adherent biofilm (Gristina 1987).
For the manifestation of an infection, for example following wound contamination, the first 5 hours are decisive, during which the invading organisms are attacked by the immune cells. Antibiotics help reduce the number of pathogens during this phase (Gristina et al. 1989, König et al. 1998).
Since healthy bone tissue is highly resistant to infection, additional factors other than contamination must be present to promote an infection (Andriole et al. 1973, Burri 1979, Klemm 1979). Implants reduce the natural resistance of bones to infection by a factor of 1000, whereby the threshold number of pathogens/infectious dose which can cause an infection in healthy bone tissue is reduced from 108 to 105 pathogens (Rüter et al. 1995).
If the pathogens have initially reached or entered the bone, specific mechanisms enable them to adhere to the surface of the implants. These include bacterial factors, surface characteristics of the implants, and host factors. Adherence of the pathogens is achieved by polysaccharides in their capsules and surface components resembling fimbria. Electrostatic and hydrophobic interactions (van der Waals forces) between bacteria and the implant surface also play an important role.
On the part of the host, the function of fibronectin is particularly important in pathogen adhesion to plates, nails, and screws (Fischer et al. 1996).
Bacterial adhesion to the surface of implants is a decisive prerequisite for the development of a biofilm. The term biofilm can be defined as a structured collection of bacteria (microcolonies) which produces a glycocalix through the synthesis of an extracellular slimy substance or exopolysaccharides and can be found on the surface of both vital and inert structures. This resultant slime protects the pathogens from the body’s own immune defenses and also from antibiotics (Peters et al. 1982). The biofilm can be mono- or polymicrobial and is considered a potential source of spread of infection (Berendet 1999, Costerson et al. 1999, O’Gara et al. 2001, Donlan 2002; Fig. 2.2).
This creates the foundation for the proliferation of pathogens in bone tissue or on the implant covering the bone. Local ischemia and devitalized bone fragments following an operation or trauma decisively promote the proliferation of bacteria (Norden and Kennedy 1970, Emslie and Nade 1983).
Microembolisms, endotoxin release, and granulation tissue further decrease blood circulation and can disturb the medullary and periosteal circulation and lead to sequestration (Khouri and Shaw 1989).
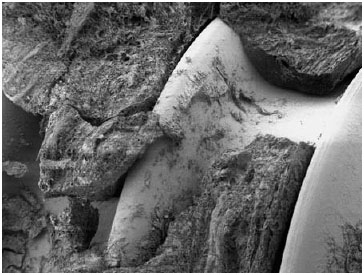
Fig. 2.2 Biofilm formation by S. aureus on a screw.
Sequestra constitute an ideal nutrient medium for the further spread of bacteria, which can evade endogenous immune defenses and antibiotic therapy in this necrotic tissue (Notzli et al. 1989, Klemm 1993).
Pathogens
Staphylococci are the most common pathogens detected in connection with osteomyelitis, and they often appear in mixed infections (Gentry 1988, Perry et al. 1991, Haas and McAndrew 1996, Schnettler et al. 1997; Table 2.1, Fig. 2.3). Staphylococcus aureus is the most common species, accounting for 30% (Gentry 1988) to 70% (Perry et al. 1991, Haas and McAndrew 1996, Schnettler et al. 1997) of bone infections; however, coagulase-negative staphylococci, like Staphylococcus epidermidis, also deserve mention. Staphylococcus epidermidis is found in up to 90% of bone infections following intraoperative implantation of foreign material, like joint prostheses and internal fracture fixation devices (Quie and Belani 1987, Mahan et al. 1991). This is normally a nonvirulent bacillus which colonizes the skin, but its common ability to form a biofilm predestines it to colonize implants and endanger the patient (von Eiff et al. 2002). Besides staphylococci, Pseudomonas species and other Gram-negative pathogens are detected, though less frequently (Gentry 1988, Perry et al. 1991, Haas and McAndrew 1996, Schnettler et al. 1997).
During the past years, an increasing number of multi-resistant pathogens have been identified as causative agents for infections in many medical fields. In one study in the USA the proportion of nosocomial infections with methicillin-resistant S. aureus (MRSA) increased from 2.4% in 1971 to 29% in 1991 (Panlilio et al. 1992). In the same study, this figure exceeded 38% in large hospitals with over 500 beds. The incidence of MRSA infections is increasing, especially in intensive care units. In 1995 a European prevalence study reported that up to 60% of infections in intensive care units were caused by MRSA (Vincent et al. 1995).
The fields of emergency and orthopedic surgery have also witnessed an increase (not only in absolute terms, but also in terms of percentage) in infections with multi-resistant pathogens, for example, of joint prostheses (Kilgus et al. 2002). Treating infections with multiresistant pathogens is much more difficult and costly than infections with nonmultiresistant pathogens (Kilgus et al. 2002, Herr et al. 2003).
Table 2.1 Confirmed pathogens in osteomyelitis (Gentry 1988).
Pathogen | 1987 (n = 192) | |
---|---|---|
Staphylococcus aureus | 57 | (30%) |
Pseudomonas aeruginosa | 18 | (9%) |
Mixed infection | 70 | (36%) |
Other Gram-positive pathogens | 17 | (9%) |
Other Gram-negative pathogens | 25 | (13%) |
Unclassified bacteria | 5 | (3%) |
Staphylococcal resistance to methicillin and all other β-lactam antibiotics is associated with the production of the penicillin-binding protein PBP2a, which is not found in methicillin-sensitive Staphylococcus species (Pierre et al. 1990, Chambers 1997). The PBP2a protein is encoded by the mecA gene, whose regulation is controlled by the mecR1-mecI inducer-repressor system (Matuhashi et al. 1986, Tesch et al. 1990, Hackbarth and Chambers 1993, Sharma et al. 1998). The mecR1 gene encodes a β-lactam-detecting transmembranous signal protein; the mecI gene encodes a repressor protein for the mecA gene. Loss or inactivation of the mecI gene removes the inhibition of the mecA gene, thereby leading to resistance to methicillin and other β-lactam antibiotics (Suzuki et al. 1993, Shimaoka et al. 1994, Weller et al. 1999).
For a detailed discussion of antibiotic treatment and special precautions against MRSA and other multiresistant pathogens, see Chapter 4.
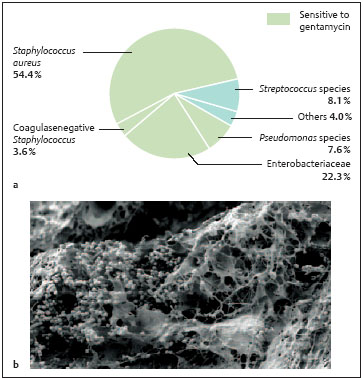
Fig. 2.3a, b Pathogen spectrum. S. aureus
Risk Factors
Local:
• Disturbances in arterial and venous circulation
• Tissue damaged by radiation
• Extensive exposure of the operation site
• Extensive soft-tissue defects
• Extensive hematomas
• Remaining hollow spaces
Systemic:
• Diabetes mellitus
• Nicotine and drug use
• Disturbances of liver and kidney function
• Severe infections with immunosuppression
Besides bacteria-host interactions, there exist interactions between the host and implants, independent of bacterial colonization. Polymorphonuclear neutrophilic leukocytes are part of endogenous immunity and are activated not only by bacteria, but also by implant surfaces. With the aid of the enzymes in their cell membranes they produce not only nitrogen and intermediate oxygen products, but also hypochloric acid and proteases and release antibacterial peptides, also known as defensins, for antimicrobial resistance. These aggregate, integrate into the pathogens’ cell membranes, and perforate them (Kaplan et al. 1999, Schiefer 2003).
Colonization with pathogens on the surface of implants, prostheses, and/or bones causes infection with a typical pathologic appearance:
• Acute, inflammatory reaction around the implant or bone with all signs of infection
• Chronic inflammatory reaction with loss of bone on the surface in contact with the implant caused by activation of osteoclasts and recruiting of cells originating from monocytes
NOTE
The main characteristic of an infected implant or bone is the presence of an inflammatory infiltration.
In principle, the disease can be categorized according to:
• Clinical symptoms (acute or chronic)
• Infective mechanism (exogenous or endogenous-hematogenous)
• Wound status (simple wound, chronic ulcer, multiple fistula with and without contact with the body surface)
• Host reaction (abscess formation or not)
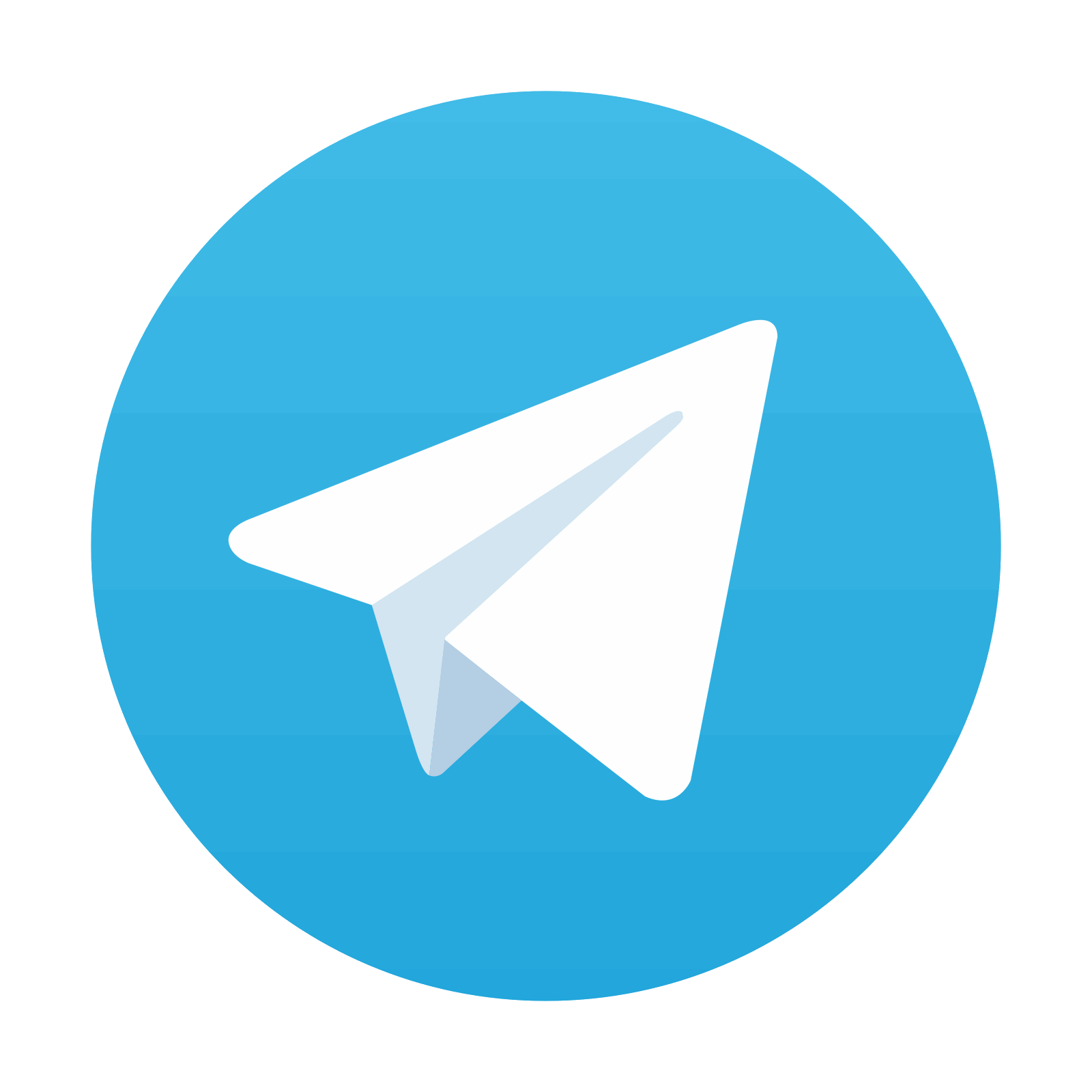