Pathogenesis and treatment of impaired fracture healing
Introduction
Bone is a unique tissue capable of repair and regeneration to its original state. This involves several processes including recruitment of osteoprogenitor cells to the fracture site and their induction into chondrocytes and osteoblasts. In the final stage, osteoconduction directs bone production into a mechanically stable three-dimensional array [1]. Despite being a well-orchestrated biological process, an estimated 5–10% of the fractures occurring annually in the United States exhibit some degree of impaired healing [2]. This not only results in considerable morbidity including loss of productivity and independence, but also has significant financial implications. Treatment of tibial nonunion is estimated to cost an average of US$11,332 per case [3].
Nonunion is the process in which bone is unable to achieve continuity and regain its function after injury [4]. Generally, this is considered to have occurred if a fracture has failed to heal within 6–8 months. Clinically, there may be pain and motion at the fracture site with the fracture gap consisting predominantly of fibrous tissue with varying amounts of cartilage [5]. Atrophic nonunions characteristically contain very little callus around a fibrous tissue-filled fracture gap. In contrast, hypertrophic nonunions demonstrate abundant callus formation without bridging the skeletal defect. In many instances, the cause is unknown and may be related to inadequate reduction, instability, distraction of the fracture fragments by fixation devices or traction, excessive periosteal stripping, the systemic state of the patient, or the nature of the traumatic insult itself [6–10] (Table 1.2.2-1). In addition, there are certain areas within the appendicular skeleton that have a predilection to impaired healing due to aspects of the local biomechanical environment. Examples include the subtrochanteric region of the femur where, prior to the introduction of locked intramedullary nailing, controlling the mechanical environment was challenging [11], and forearm fractures. In 1949, Knight and Purvis [12] reported a nonunion rate of up to 46% in their patients with radial and ulnar shaft fractures treated with open reduction and internal fixation. Following the introduction of modern internal fixation devices, this rate has dramatically decreased to between 1.4 and 2.7% [13].
Biological factors | Mechanical factors |
Patient age | Soft-tissue attachments to bone |
Comorbid medical conditions | Stability (extent of immobilization) |
Functional level | Anatomical location |
Nutritional status | Level of energy imparted |
Nerve function | Extent of bone loss |
Vascular injury | |
Hormones | |
Growth factors | |
Health of the soft-tissue envelope | |
Sterility (in open fractures) | |
Cigarette smoke | |
Local pathological conditions | |
Level of energy imparted | |
Type of bone affected | |
Extent of bone loss |
Factors influencing fracture repair
Fracture healing involves the complex interplay of a milieu of systemic and local factors. Any disruption leads to alterations in the bone-healing process, resulting in delayed unions or nonunions. In order to develop a systematic method to determine which key factors are involved, it is useful to divide them into local and systemic subcategories.
Local factors
Blood supply
Preserving sufficient blood supply to the fracture segments is thought to be the most essential factor. This can be seen in cases of proximal pole fractures of the scaphoid, which are more likely to progress to nonunion than other scaphoid fractures because of their precarious vascularity [15]. Vulnerable blood supplies to the femoral head and talus similarly predispose these bones to impaired healing and nonunion after injury [16].
The nature and severity of the injury has a significant effect on the bone′s ability to heal as there may be comminution of bony fragments and decreased blood supply at the fracture site [17]. Comminution of bone fragments usually indicates that there is also significant injury to the soft-tissue envelope. The most affected area of bone corresponds to the zone of injury. As a result of the shearing forces, the bone-periosteum functional vascular unit will be disrupted from the surrounding muscles, resulting in devascularization of the fracture fragments. This in turn provokes impaired vascular proliferation and cellular response, resulting in impaired healing [18]. According to Hierner and Wood [19], this is most evident in the tibia, owing to significant soft-tissue injury associated with the fractures. Open injuries further exacerbate the problem as exposed bone and soft tissue desiccate, thereby increasing the volume of necrotic tissue and subsequent risk of infection. Additional insult by the surgery might further compromise healing potential. The current interest in minimally invasive osteosynthesis and soft-tissue sparing techniques is a reflection of that concern. Techniques and implants do now allow the surgeon to respect “fracture biology”.
The importance of the periosteal blood supply in fracture healing has been the subject of several studies [20, 21] (Fig 1.2.2-1). Reed et al [22] demonstrated that derangements in the vascular response to injury during the early stages of the repair process may result in nonunion. Numerous studies have now shown a pivotal role of vascular endothelial growth factor (VEGF) in helping generate an optimal environment for bone regeneration [23]. The hypoxia inducible factor-1α (HIF-1α) pathway has been shown to be a critical mediator of neoangiogenesis required for skeletal repair [24]. Mathematical models have been able to confirm the essential role of angiogenesis in normal fracture healing [25].

Mechanical stability
Mechanical stability is a major requirement for normal fracture healing, especially when there is an extensive soft-tissue injury or when the blood supply to the fracture site is compromised. Understanding the role of mechanical stability and interfragmentary motion on fracture healing is rapidly evolving. Pauwels and Urist already explained in detail (histomorphological) aspects of normal healing and pseudarthrosis formation [26]. Bone cells are able to sense their environment, which can profoundly affect their growth and differentiation. It is here that the treating surgeon can play a large role by the choice of treatment. Perren′s strain theory of fracture healing helps understand this concept [27]. His theory proposes that hydrostatic pressure steers pluripotent mesenchymal cells towards a chondrogenic pathway; shear or tensile stress leads to fibrogenesis, whereas a combination of hydrostatic pressure and shear or tensile strain gives way to fibrocartilage [26, 27]. It should, however, be noted that the “ideal” healing environment from a mechanical perspective is still unclear. To further complicate understanding: different fractures and different areas of the skeleton respond in different ways.
Excessive interfragmentary motion, secondary to inadequate stabilization, also results in disruption of fracture hematoma, preventing fracture callus formation and leading to development of a nonunion. Clinically, this can be seen with fractures of the femoral neck, where altered biomechanics play a significant role in the generation of a nonunion. Frangakis [28] noted this to be especially important in displaced fractures, where the incidence of avascular necrosis and nonunion was higher in cases without adequate fixation. The effects of mechanical instability can be seen at the cellular level. Page et al [29] used a rabbit tibia model to study the effects of mechanical stability on the connective tissues produced during fracture healing and noted cancellous bone formation over the entire periosteal surface by 5–7 days in mechanically stable fractures with concomitant type I collagen production. In contrast, within the unstable fractures, cancellous bone formed away from the fracture gap with a predominance of type II collagen, a marker of cartilage formation. This was gradually replaced by type I collagen during endochondral ossification. Hankemeier et al [30] demonstrated that this prolonged chondrogenesis in unstable fractures may be attributable to alterations in macrophage expression. Claes et al [31] postulated that this discrepancy in callus-tissue differentiation may be due to differences in local vascularization.
Geris et al [32] have developed mechanobioregulatory models, in which the influence of both the mechanics and the biology on fracture healing is captured. They will help us to further understand the interactions between mechanics and angiogenesis in bone healing. These complex models have shown good agreement with experimental studies investigating the interplay between osteogenesis and angiogenesis.
Fracture fragment distraction
Lack of fracture fragment apposition has been shown to contribute to impaired healing. Frangakis [28] reported a 20% nonunion rate in 76 femoral neck fractures treated with internal fixation and found that the greater degree of fracture displacement, the more likely the fracture was to progress to a nonunion. In a study of 138 patients with femoral neck fractures, Nilsson et al [33] also showed that the development of nonunion and segmental collapse of the femoral head was significantly influenced by fracture displacement.
Reducing the fracture gap results in greater bony contact, thereby decreasing the volume of repair tissue. This is especially important in injuries with significant soft-tissue stripping as the biology of the repair process is already impaired [34, 35]. Segmental fractures also pose significant challenges to fracture union. This may be related to the fact that these injuries are almost always caused by hig-energy direct trauma and are frequently associated with significant soft-tissue and vascular injuries. These problems occur most frequently in segmental fractures of the tibia, especially at the distal fracture site. This was highlighted by the review of Rommens et al [36] of the postoperative problems of 40 patients and noted impaired bone healing in 29% of patients in relation to the distal fracture. Segmental fractures of the femur less commonly form nonunions, presumably because of better soft-tissue coverage and better blood supply.
Soft-tissue interposition
Soft-tissue interposition, including muscle, fascia, tendon, and nerves between fracture fragments, act as a physical barrier to the repair process. Interposition should be suspected when the bone fragments cannot be aligned anatomically using closed reduction maneuvers. Under these circumstances, an open reduction may be necessary to remove the interposed tissue and facilitate reduction of the fracture [37].
Infection
Open fractures can have a devastating effect upon the biology of fracture healing. Not only can they be associated with extensive soft-tissue damage, disruption of the blood supply and fracture comminution, but the high likelihood of infection in the fracture site only further compounds the problem. Malik et al [38] conducted a logistical regression analysis of 214 long-bone fractures to identify the factors affecting rates of infection and nonunion after intramedullary nailing. They found that open fractures were found to be associated with nearly a threefold increase in deep infections compared to closed injuries. In addition, opening the fracture at the time of surgery was found to be significantly associated with the development of nonunion after intramedullary nailing.
During the fracture-healing process, cells from the marrow, periosteum, and neighboring soft tissues are recruited and stimulated. If the fracture site is infected, these cells are diverted away from their primary reparative roles to wall off and eliminate the infection. Infection also causes necrosis, tissue edema, and thrombosis of blood vessels, thereby further compounding the problem. Trying to eradicate this is a complex surgical problem. Toh and Jupiter [39] treated 37 patients with infected tibia nonunions. Patients were evaluated at an average of 61 months after treatment, and union with eradication of infection was achieved in 35 patients. These results highlight the problem that infected nonunions of the tibia represent a chronic and debilitating disorder with a lasting impact for both the patient and treating surgeon.
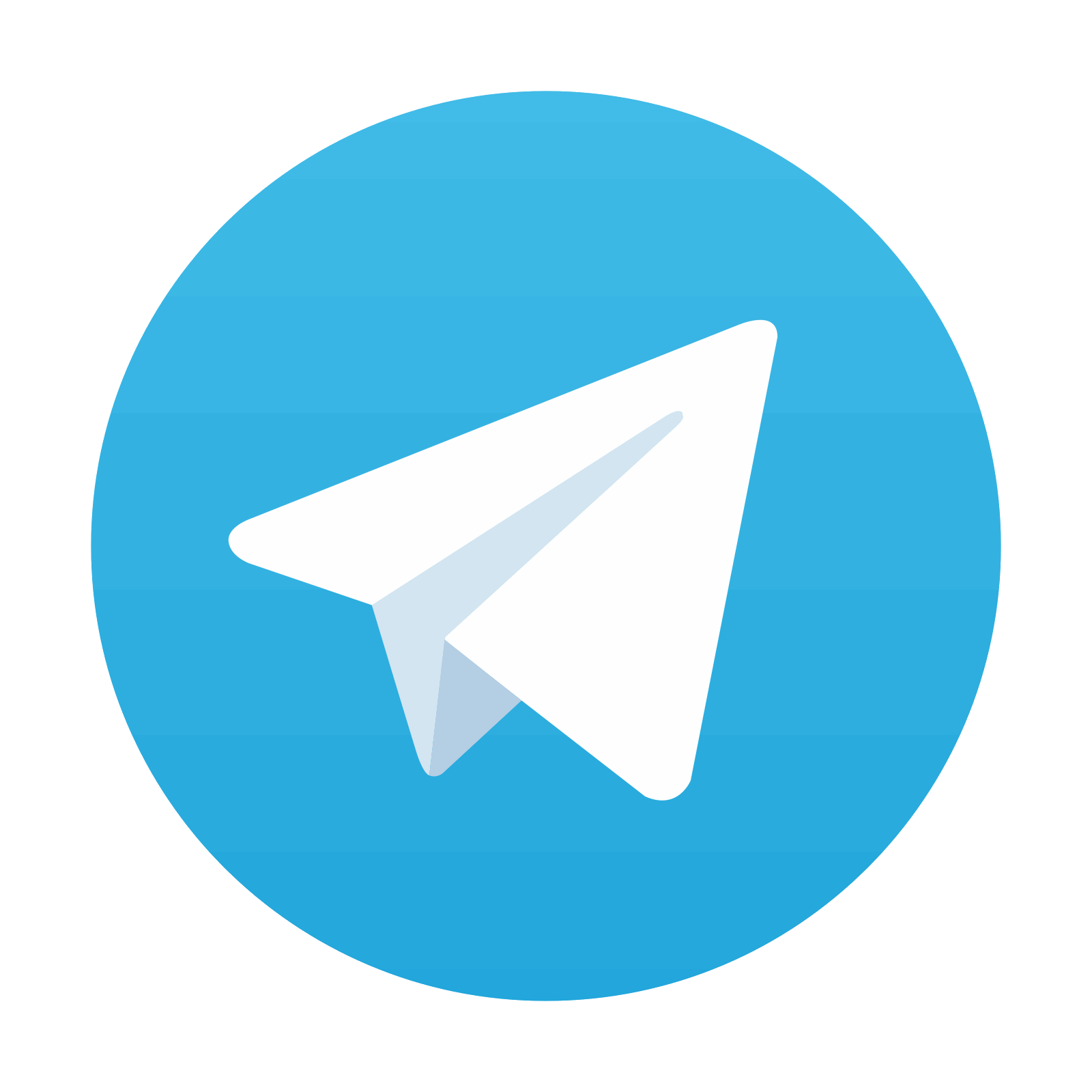
Stay updated, free articles. Join our Telegram channel

Full access? Get Clinical Tree
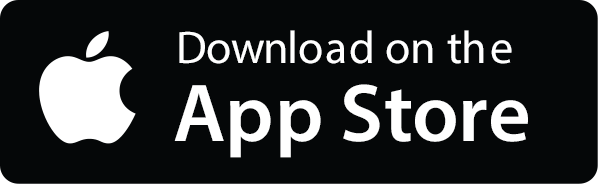
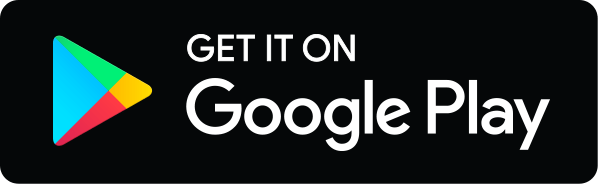
