Overview of Primary Acetabular Components
Michael D. Hellman
Roshan P. Shah
Craig J. Della Valle
Harry E. Rubash
Cemented Acetabular Components
Introduction
Sir John Charnley performed his first six human cases of low friction total hip arthroplasty with methylmethacrylate cement in 1958 (1). Thereafter, the use of bone cement proliferated in Europe. The United States followed when the Food and Drug Administration approved widespread use of methylmethacrylate as bone cement in 1971. Since that time, improvements in implant design and surgical technique have helped increase the clinical outcomes and longevity of cemented acetabular components (2,3).
Clinical survivorship for cemented acetabular components has been excellent. Berry et al. (4) reported survivorship in 2,000 patients, and found 15-year rates of 96.5% and 25-year rates of 89.9%. However, since loosening usually occurs asymptomatically, these results may underestimate the true rate of failure (5). Radiographic studies have shown that cemented acetabular components fail approximately twice as often as what reported revision rates suggest (Fig. 52.1) (6,7,8,9). Callaghan et al. (9) showed an exponential increase in cemented acetabular failure after 10 years by combining data for revisions for aseptic loosening and radiographic acetabular loosening (Fig. 52.1B). They reported a survivorship rate of only 64% at 25 years. The mode of loosening appears to be biologic over mechanical (10). Schmalzried et al. (10) showed that there is a progressive, three-dimensional resorption of trabecular bone immediately adjacent to the cement. This occurs secondary to a macrophage reaction induced by high-density polyethylene particulates migrating along the cement–bone interface.
Today, cemented acetabular components are used uncommonly in North America. However, with increasing scrutiny on healthcare costs and clinical outcomes there may be a role for these implants in certain patient populations in the future.
Design Considerations
Cement Thickness, the Flange Design, and Cement Spacers
Properly cementing the acetabular component is vital to ensure implant survival. Concern with cementing technique has been voiced by expert hip surgeons since Charnley’s era. Although all agreed that cement thickness is important in the success of acetabular fixation, the ideal cement thickness was debated, ranging from 2 (11,12) to 4 mm (13,14), to even as thick as 5 to 6 mm (15). Heaton-Adegbile et al. (15) showed a reduction of tensile strains on the bone–cement interface by 50% with a thicker mantel (p < 0.001) and concluded that a 5- to 6-mm cement mantle may preserve the structural integrity of principal load-bearing quadrants of the acetabulum better than a cement mantle of 2 to 3 mm. Thickness of the cement mantle has also been correlated with decreased strain of subchondral bone (13,15). A thin or grossly deficient cement mantle may cause cement fracture, which leads to early loosening (16). Furthermore, rapid polyethylene wear has been correlated with a thin cement mantle in the weight-bearing area (17).
To increase the penetration of the cement mantle into host bone, Charnley designed a peripheral flanged polyethylene liner (Fig. 52.2) (18). The purpose was to obstruct the egress of polymethylmethacrylate around the periphery, therefore increasing the pressurization at the cement–bone interface. Simulation models showed that flanged sockets have a markedly better cement intrusion rate and cement intrusion volume when compared to nonflanged sockets (18). Further, Hodgkinson et al. (19) showed that radiographic signs of loosening were significantly less when comparing flanged cemented cups to nonflanged. Radiologic appearance of the cement–bone interface was better for the flanged group both at the time of implantation and at 10-year follow-up. At 10 years, 42.7% of flanged sockets still showed no evidence of radiologic loosening, versus only 30.3% of nonflanged sockets showed no evidence of radiologic loosening. Their conclusions were that the flange design significantly improves the initial polymethylmethacrylate cement penetration, which is vital to long-term implant survival (19).
To ensure a uniform cement mantle, Nelson et al. (20) introduced polymethylmethacrylate cement spacers in 1980. These spacers are polyethylene pegs of a certain thickness that are evenly distributed around the backside of a polyethylene cup (Fig. 52.2). The spacer was developed to more consistently achieve correct placement of the polyethylene
cup and to ensure adequate cement thickness surrounding the cup. But intermediate and long-term clinical results have shown less favorable outcomes than expected (9,21). Faris et al. (22) found a significantly higher incidence of early failures when comparing cups with spacers to cups without spacers (p < 0.038). He concluded that a spacer likely prevents additional cement pressurization when the cup is being seated (22).
cup and to ensure adequate cement thickness surrounding the cup. But intermediate and long-term clinical results have shown less favorable outcomes than expected (9,21). Faris et al. (22) found a significantly higher incidence of early failures when comparing cups with spacers to cups without spacers (p < 0.038). He concluded that a spacer likely prevents additional cement pressurization when the cup is being seated (22).
Polyethylene Thickness
Charnley demonstrated that the thickness of polyethylene directly affects the stresses within the cement–bone interface by using a photoelastic model (1). His thinner polyethylene model had 50% higher stresses transmitted to surrounding tissues, and was concentrated over a smaller arc than a thicker polyethylene model. Finite element analysis has helped confirm Charnley’s work by showing that a thicker polyethylene decreases the stresses in the subchondral bone (23), and within the polyethylene itself (24). Decreasing stress within a liner will help decrease liner wear rates, associated osteolysis, and ultimately increase implant survival. Berry et al. reported an increased risk of polyethylene wear and liner fracture when the thickness is less than 5 mm. Bartel et al. (24) endorsed a liner of at least 6 mm thick and ideally 8 mm thick.
Metal-backed Components
Metal-backed cemented acetabular cups were first reported in 1971 by Harris (25). He designed a chromium-cobalt shell with a modular high-density polyethylene liner. He wanted to improve longevity of the implant by making the polyethylene liner replaceable without disturbing the cement–bone interface. This was the first modular acetabular component with a polyethylene liner that could be exchanged at a later date if wear occurred. Two-dimensional finite element analysis showed that a 2-mm metal backing significantly reduced the stresses on the polyethylene liner, cement, and
subchondral bone (26). Soon modular and nonmodular metal-backed, cups became popular.
subchondral bone (26). Soon modular and nonmodular metal-backed, cups became popular.
Early studies showed good short-term clinical results, and so these components were adopted into wide use throughout the 1980s (27,28,29). Intermediate-term results were not as favorable. Harris and Penenberg (30) reported on 48 hips at an average follow-up of 11 years that 21% of metal-backed cemented sockets required revision surgery and 41% showed radiographic loosening of the sockets. Similarly, Mohan et al. (31) reviewed their 10-year results of the Howse II nonmodular cemented metal-backed acetabular cups and found that the survivor rate was only 42%. Reasons for poor outcomes are theorized to be due to accelerated wear of the polyethylene liner. Unlike the two-dimensional models originally reported for metal backed cemented cups, the three-dimensional modeling showed that there were peak stresses in the polyethylene and cement (32). Nonmodular metal-backed designs also did not have a true mechanical bonding of the metal with the polyethylene allowing for motion and backside wear (31). Further, the metal backing caused the polyethylene liner to be thinner which has been shown to decrease survival rates (33). Because of these poor results, the metal-backed implant has been abandoned, and all-polyethylene cups remain the gold standard for cemented acetabular components.
Cementless Acetabular Components
Introduction
Cementless acetabular components were originally designed to only achieve mechanical fixation. First-generation designs had several different geometries. Morscher described five main types: (a) a cylindrical socket, (b) a square socket, (c) various types of cones, (d) an ellipsoid threaded ring, and (e) a hemispherical cup (34). The threaded type was introduced in 1974 and was among the most commonly used of first-generation designs (Fig. 52.3) (35). A number of studies showed these implants had initial satisfactory results but longer-term follow-up showed they exhibited unacceptably high migration rates of up to 56%, and revision rates for aseptic loosening from 4% to 31% at mean follow-ups of 3.5 to 10 years (36,37,38,39,40,41).
Second-generation cementless acetabular components were designed to achieve both mechanical fixation and lasting biologic fixation. The addition of a porous coating to provide for bone ingrowth showed more promising results for the threaded ring design. A matched pair analysis of an otherwise identical cup design, with a nonporous threaded cup (S-ROM Anderson, Joint Medical Products) and a porous-coated threaded cup (S-ROM super cup), showed significantly better performance of the porous-coated design at 2- to 4-year follow-up, with a 0% radiographic loosening of the porous cup compared to a 29% loosening of the nonporous cup (41). Despite these better results, the threaded shape remained fraught with problems including difficulty of insertion and high rates of polyethylene wear (41,42,43). Furthermore, a threaded acetabular component has poor bone contact for biologic fixation, with as little as 9% surface area in contact with the pelvis (44,45). These problems resulted in a move to alternative designs and the hemispherical cup gained significant momentum.
The hemispherical design has proven to be most successful shape for cementless acetabular components and virtually all porous-coated acetabular components available today have a hemispherical or modified hemispherical shape. They are most commonly made with pure titanium, titanium-base alloy, or chromium-cobalt alloy. Almost simultaneously, the first porous-coated hemispherical implants were introduced: the Anatomic acetabular component (PCA; Howmedica, Rutherford, NJ) and the Harris-Galante porous-coated acetabular component (HG-1; Zimmer, Warsaw, IN) (Fig. 52.4). Soon after, Anatomic Medullary Locking (AML; DePuy, Warsaw, IN) also was introduced. The PCA was composed of chromium-cobalt alloy with a double layer of sintered chromium-cobalt beads and two peripheral pegs for rotational stability. The HG-1 porous acetabulum was composed of a titanium shell with titanium fiber metal diffusion bonded to the acetabular shell with screw holes for additional fixation. The AML acetabular component was composed of cobalt-chromium alloy with sintered beads and three spikes for rotational stability. Based on the excellent early results with these devices, numerous similar implants have been developed, differing only slightly in shell
geometry, metallurgy, or mechanism of fixation. Now, with over 20-year favorable radiographic and clinical results, the hemispherical, porous-coated, cementless acetabular device with or without adjunctive screw fixation remains the implant of choice in North American centers for primary total hip arthroplasty (46).
geometry, metallurgy, or mechanism of fixation. Now, with over 20-year favorable radiographic and clinical results, the hemispherical, porous-coated, cementless acetabular device with or without adjunctive screw fixation remains the implant of choice in North American centers for primary total hip arthroplasty (46).
Design Considerations
Porous-coated Metal Implants
In an effort to improve long-term fixation of acetabular components, the concept of biologic fixation, via bone ingrowth, into porous-coated acetabular components was introduced. The requirements for bone ingrowth are that the implant materials are biocompatible, and that the surface has an optimal pore size. The material needs to be in intimate contact with viable bone and have adequate initial stability during the incorporation process in order for bone ingrowth to occur consistently (47,48,49). Earlier canine studies demonstrated encouraging results with porous-coated acetabular components to form an enduring biologic bond to the skeleton (50). Thus, porous-coated acetabular components were introduced into clinical practice in 1983 and 1984 (51,52,53).
The manufacturing process involves applying sintered cobalt-chromium beads, titanium fiber metal, cancellous structured titanium, or plasma-sprayed titanium particles onto a metal cup while ensuring a proper pore size. The attachment of the porous-coated surfaces to the underlying acetabular shell has been an area of concern because of bead or particle detachment (52,54), but modern day manufacturing processes have allayed these concerns. During investigations into the surface structure of porous implants, Bobyn et al. (55) found strength of fixation to be greatest in implants with pore sizes ranging from 100 to 400 μm.
Both chromium-cobalt and titanium are biocompatible and capable of supporting bone ingrowth. Qualitatively, these metals show no difference in bone ingrowth observed (56); but quantitatively, titanium has shown increased bone density, deeper penetration, and a greater degree of mean bone ingrowth as compared to chromium-cobalt alloy (57). Titanium also has a lower modulus of elasticity, closer to that of bone, which decreases the effect of stress remodeling of the host bone (58
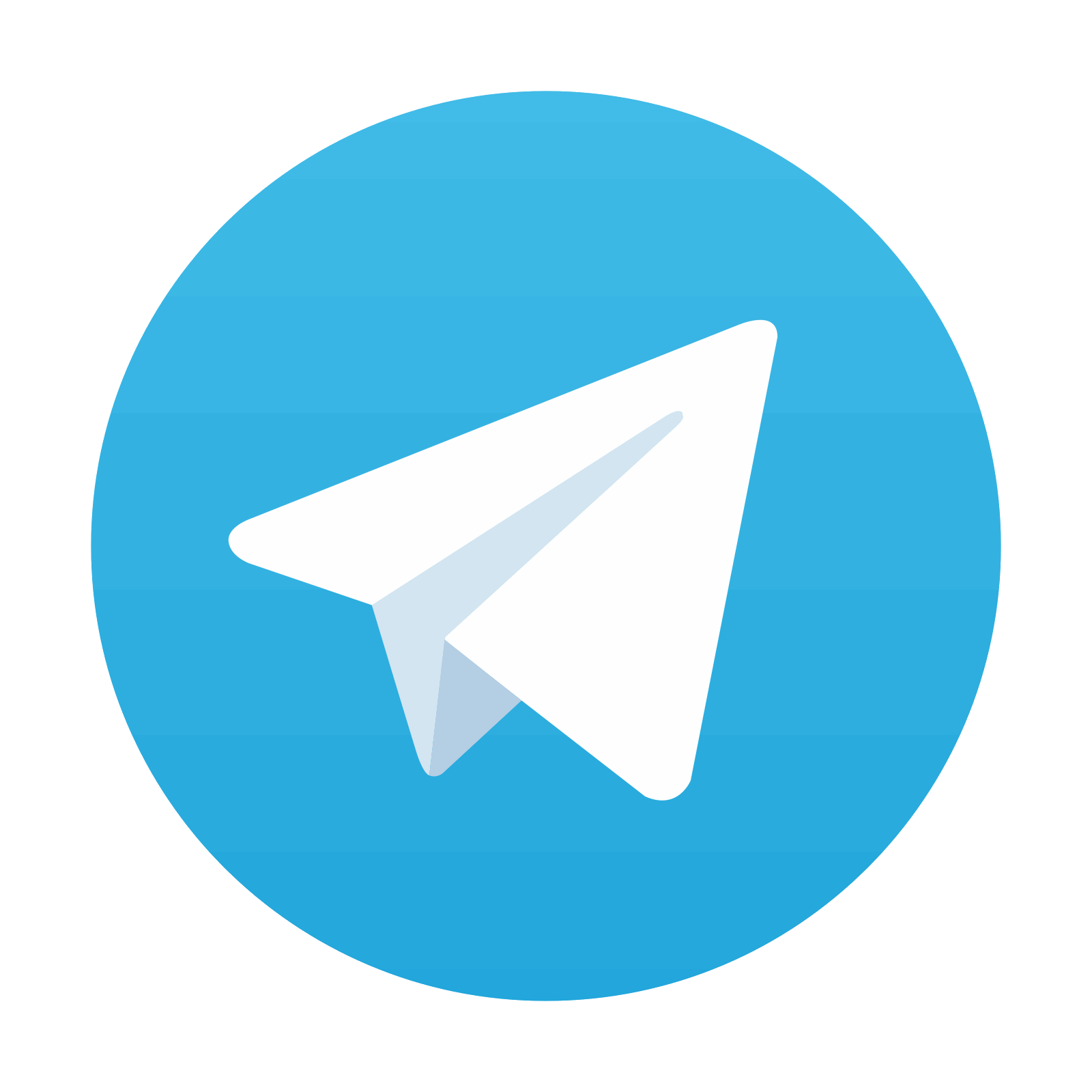
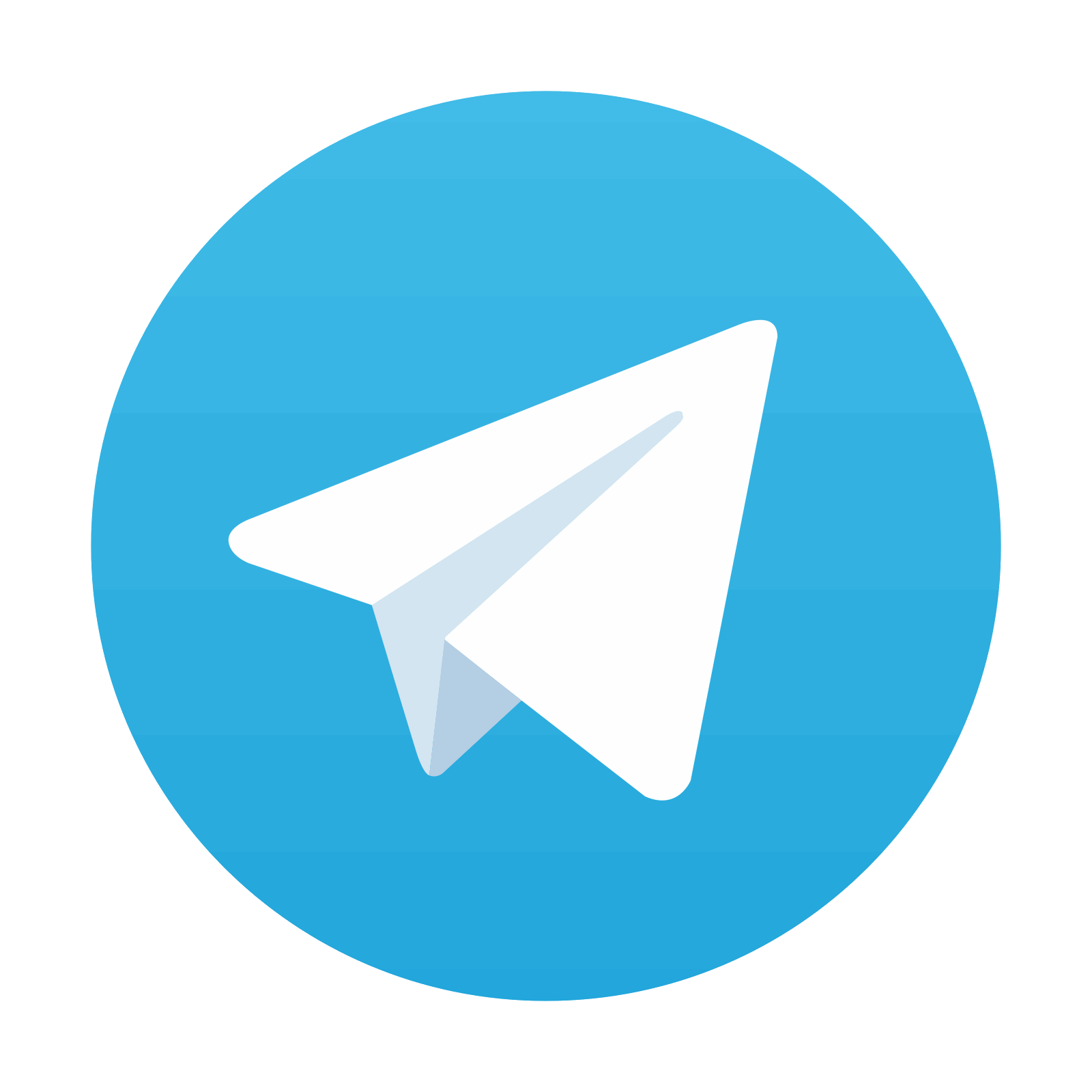
Stay updated, free articles. Join our Telegram channel

Full access? Get Clinical Tree
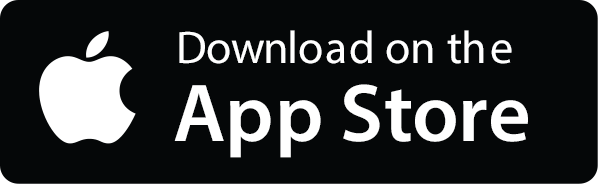
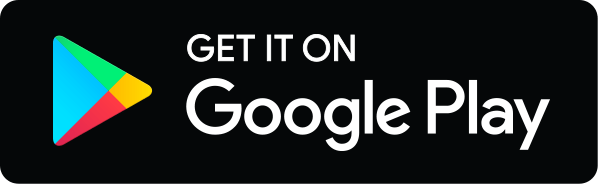
