Overview of Orthobiologics for Bone Repair
Mirtijn van Griensven, MD, PhD
Elizabeth Rosado Balmayor, PhD
Dr. van Griensven or an immediate family member serves as a board member, owner, officer, or committee member of DGOU and TERMIS. Dr. Balmayor or an immediate family member serves as a board member, owner, officer, or committee member of DGOU, EORS, and TERMIS.
INTRODUCTION
An overview of physiologic bone composition regarding cells and matrix as well as the anatomic features is provided. This natural information is the basis for describing the possible therapeutic options comprising biomaterials, cells, or a combination thereof. These orthobiologic approaches are discussed in detail, along with the importance of the different components for certain pathologies. Furthermore, the individual entities and the combinations have different legislative implications, such as the law on advanced therapy medicinal products.
BRIEF BIOLOGY OF BONE
The bone is a very specialized organ; its main function is to support the human body. Because of its composition, it is a hard material allowing the skeleton to protect the inner organs. In addition to the structural support, the bone contains bone marrow that is important for the production of blood and also contains other progenitor cells, including mesenchymal stem cells (MSCs). The bone plays an important role in calcium homeostasis as well as in the secretion and sequestration of growth factors and cytokines.1
Composition: Matrix and Cells
The bone is characterized as a connective tissue. The main component is mineralized extracellular matrix. In the extracellular matrix, different cell types are embedded. The extracellular matrix consists of an organic and an inorganic part. The organic extracellular matrix part together with the different bone cells comprises the organic mass. The organic bone mass consists of 90% of collagen type I and the other 10% of small proteins such as osteonectin and osteocalcin.2 The inorganic bone mass, which is approximately 70% of the bone dry mass, consists of 99% of the alkaline mineral hydroxyapatite.
The bone consists of three major cell types: osteoblasts, osteoclasts, and osteocytes. Furthermore, osteoprogenitor cells being part of the MSCs are also present. Osteocytes are terminally differentiated cells in the bone. Osteoblasts, however, are able to build up new bone. In contrast, osteoclasts dissolve bone mass. The osteoblasts are derived from the MSCs, whereas osteoclasts are derived from hematopoietic stem cells.
Function: Structural and Biologic
The bone tissue has different functions that are structural and biologic. Structurally, bones provide protection to the inner organs from outside forces. Furthermore, bones allow humans to stand upright and maintain their posture. These functions depend on the strength of the bone and are related to its hardness. This is determined by the mineralized extracellular matrix. This results in the biologic function for calcium homeostasis. Bone tissue is a depot for calcium and is involved in the intricate homeostatic circle of calcium depending on resorption in the intestine, excretion in the kidney, and storage in the bone tissue. This homeostasis is closely regulated by hormones such as calcitonin, calcitriol, and parathyroid hormone (PTH). The amount of calcium also partially depends on estrogens, as can be seen that during menopause when estrogen levels are decreased, a decreased calcium content in bones is observed. Because certain bones, as outlined in the next section, contain bone marrow, bone also has an important function in the production of blood cells. Moreover, bone marrow hosts not only hematopoietic stem cells for blood production but also MSCs for the regeneration of other tissues.
HIERARCHY OF STRUCTURE, INCLUDING MORPHOLOGY AND HISTOLOGY
Macroanatomy
The human skeleton consists of 206 bones. The skeleton can be divided into an axial skeleton and an appendicular skeleton. The axial skeleton consists of cranial bones, spine, ribs, and sternum. The upper and lower extremities, the shoulder, and the pelvis belong to the appendicular skeleton. In total, five different bone types are present in the body: long, short, flat, irregular, and sesamoid bones. The long bones are characterized by a longer length than diameter. In the middle of the long bone, a hollow space mostly filled with bone marrow is available. This hollow space is absent in short bones, and the ratio between length and diameter is close to 1 and these bones are mostly cubic. Flat bones are extensive but flat as the
name indicates. These are, for instance, the cranium, pelvis, scapula, and ribs. Bones that cannot be characterized by the previously described forms are called irregular bones such as vertebra and bones of the face. Sesamoid bones are the smallest bones in the body, and they have a special role where tendon is entering other type of bones. The sesamoid bones serve as a type of force reductor.
name indicates. These are, for instance, the cranium, pelvis, scapula, and ribs. Bones that cannot be characterized by the previously described forms are called irregular bones such as vertebra and bones of the face. Sesamoid bones are the smallest bones in the body, and they have a special role where tendon is entering other type of bones. The sesamoid bones serve as a type of force reductor.
Long Bones
Long bones as stated previously have a medullary canal. This is in the middle two-thirds of the length of the long bone, which is called the diaphysis. The wall of the diaphysis is the cortex and consists of compact bone. On both ends of the diaphysis, the epiphysis is present. The transition from diaphysis to epiphysis is the metaphysis, which is the area of the long bone where growth occurs before having reached the final length. The epiphysis has an irregular structure and is part of the joints. Therefore, the epiphysis is covered with cartilage. The inside of the epiphysis is spongy bone and here bone marrow is also present. The entire long bone is surrounded by periosteum. The periosteum consists of two layers and is connected to the cortical bone by very strong collagen fibers that are called Sharpey fibers. The periosteum is important because it comprises bone progenitor cells, blood vessels, and nerves. When the periosteum is not present, fracture healing is more difficult and often delayed. In the metaphysis during the growth phase, hypertrophic cartilage is present. The cartilage columns proliferate and lead to length growth of the long bones. The cartilage cells are then mineralizing and change into osteoblasts. This is the so-called enchondral ossification. Examples of long bones are femur, tibia, humerus, radius, and ulna.
Flat Bones
Flat bones are broad plates consisting of two layers of compact bone and in between a variable thickness layer of cancellous bone. In this spongy part, bone marrow is present and is one of the most prominent spaces where erythrocytes are formed. Flat bones have periosteum on both sides. Flat bones typically have a protective function; for instance, they surround the brain, lungs, and heart. Furthermore, they are insertion points for muscles. Flat bones can have different shapes such as a concave shape for the skull, a triangular shape for the scapulae, and a long flat shape for the sternum for instance. Flat bones typically develop via mesenchymal ossification, meaning directly ossified from stem cells. The osteoblasts that are present in the flat bones produce the calcium phosphate for mineralizing the flat bones. After having reached the final shape, many osteoblasts retract their extensions and thereby small channels, known as canaliculi, remain empty. Nutrients and fluid are transported via the canaliculi to the resting osteoblasts that are then called osteocytes because they are much less active, resting cells. In addition to the bone-building cells, there are also osteoclasts present that are important for bone remodeling and for calcium homeostasis.
Cancellous Bone
Cancellous bone is a porous bone and is also called spongy bone or even trabecular bone. Trabecular bone originates from the presence of the trabeculae in the cancellous bone. These trabeculae are bridges of bone on the inside of the bone (mainly in the epiphysis of the long bones and in between the compact bone plates of the flat bones). These trabeculae are aligned according to the load they carry. They are also closer together in areas where the bone encounters more force such as the femoral neck. In between the trabeculae, open spaces are present and are mostly filled with red bone marrow. Because of its structure, cancellous bone has less weight compared with the same volume of compact bone. It also provides structural support and flexibility along the lines of stress. Approximately 20% of the human skeleton is formed by cancellous bone. Because cancellous bone is important for diverting the stress and taking up load, it has a high level of metabolic activity and can be easily remodeled by osteoblasts and osteoclasts. The smallest unit in cancellous bone is called the spongy osteon. Here, bone cells form a unit together with vessels and nerves.
Compact Bone
Eighty percent of the skeleton consists of compact bone. Compact bone is also built up by bone lamellae that are, however, much denser and closer together than in cancellous bone. Thus, the structure per se is similar to cancellous bone, but the density is higher. In compact bone, these lamellae are mostly ordered in a parallel manner. This ordering is also in the direction of the load. Compact bone is found in the cortex of long bones and is also called cortical bone. It forms the outer part of both the long bones and the flat bones. Because of its compact nature, this bone is very strong, is not very flexible, and provides a high degree of protection. The smallest bone structures in compact bones are also called osteons, and they are called haversian channels. These are concentric circles of lamellae with blood vessels in the middle and bone cells concentrically arranged around them. The canaliculi are perpendicular to the circles. These haversian channels comprise approximately 45% of compact bone. These channels are also important in sensing load or movement stresses. Haversian channels are perpendicularly connected by Volkmann channels. Immature compact bone is often referred to as woven bone. Compact bone mainly consists of a very solid hydroxyapatite matrix.
Haversian Channels and Blood Supply
As stated previously, blood vessels are at the center of haversian osteons. Bone is indeed a richly vascularized
organ. This is important for the exchange of oxygen and carbon dioxide as well as nutrients and waste material. Furthermore, the blood cells that are produced in the bone marrow are transported from the bone marrow and inner parts of the bones via this vascular system. Approximately 10% to 20% of the cardiac output is reverted to the bones.3,4 Many blood vessels are present in the area with red bone marrow.5 Long bones receive blood via external vascular systems. In general, intraosseous and extraosseous blood supply by the blood vessels can be distinguished. In long bones, a systemic artery is present parallel to the longitudinal axis of the long bone. From this longitudinal artery, an artery is derived entering the diaphysis. Myoperiosteal vessels build transversal anastomoses around the diaphysis and the epiphysis. From those, additional blood vessels are derived that nurture the epiphysis and metaphysis.6 Because of this network of blood vessels, a typical structure occurs in the bone tissue. Affluent blood vessels are perpendicular to the central channel from the osteons and are also perpendicular to the Volkmann channels. Therefore, a connection between the Volkmann channels and the periosteal vasculature exists. The nutrient arteries enter the cortex via the nutrient canals to supply the diaphysis with blood. These are the strongest vessels supplying the bone tissue and clearly visible in macerated bone. The vessels are mostly paired and branch further into the medullary cavity, going up and down and further branching into. a fine capillary net. Those vessels are responsible for approximately half of the circulation in the long bones.7 Per long bone, two or more of such diaphyseal arteries are present.8
organ. This is important for the exchange of oxygen and carbon dioxide as well as nutrients and waste material. Furthermore, the blood cells that are produced in the bone marrow are transported from the bone marrow and inner parts of the bones via this vascular system. Approximately 10% to 20% of the cardiac output is reverted to the bones.3,4 Many blood vessels are present in the area with red bone marrow.5 Long bones receive blood via external vascular systems. In general, intraosseous and extraosseous blood supply by the blood vessels can be distinguished. In long bones, a systemic artery is present parallel to the longitudinal axis of the long bone. From this longitudinal artery, an artery is derived entering the diaphysis. Myoperiosteal vessels build transversal anastomoses around the diaphysis and the epiphysis. From those, additional blood vessels are derived that nurture the epiphysis and metaphysis.6 Because of this network of blood vessels, a typical structure occurs in the bone tissue. Affluent blood vessels are perpendicular to the central channel from the osteons and are also perpendicular to the Volkmann channels. Therefore, a connection between the Volkmann channels and the periosteal vasculature exists. The nutrient arteries enter the cortex via the nutrient canals to supply the diaphysis with blood. These are the strongest vessels supplying the bone tissue and clearly visible in macerated bone. The vessels are mostly paired and branch further into the medullary cavity, going up and down and further branching into. a fine capillary net. Those vessels are responsible for approximately half of the circulation in the long bones.7 Per long bone, two or more of such diaphyseal arteries are present.8
As stated previously, the periosteum contains many blood vessels as well. Because the periosteum is surrounding the entire bone, the compact bone is mainly nurtured via perfusion because of periosteal vessels. However, as already mentioned, the periosteal vessels have a connection with haversian and Volkmann channels.9 It is also still not clear how much both supply routes fulfill the metabolic needs of the bones.
The blood flow inside the bone occurs under unique circumstances. It is a closed cavity system, in which the pressure needs to stay constant. This occurs by changing the flow rate of the blood or via angiogenesis and/or vasculogenesis.10 In adult bone, the blood flow occurs centrifugal, meaning from the medullary cavity in the direction of the periosteum. This is due to the high blood pressure in the nutrient arteries and the lower pressure in the periosteal system.11,12,13 The venous blood flow is local in the epiphysis and metaphysis via a sinusoidal capillary system.8 The sinusoids drain into a central venous channel. Collecting veins flow to an emissary vein, and in the diaphysis, this vein joins the nutrient artery through the bone cortex.14 The venous system of the periosteum has connections with this collecting vein as well as with intramuscular and interfascicular veins. The venous blood flow is centripetal, meaning from the outside to the inside and mostly parallel to the arterial vessels of the bones.15
Enchondral and Mesenchymal Regeneration
Bone defects can regenerate in two different ways. This is also according to normal embryonic development. When bone regeneration occurs directly via the activity of MSCs, mesenchymal regeneration is present. Homing of stem cells to the bone defect occurs, and they differentiate into bone cells that induce mineralization of the matrix and bone tissue is formed. The MSCs can also produce messenger molecules that attract other cells that are also important for bone regeneration.
Indirect regeneration occurs when the defect starts to show chondrocytes. These chondrocytes are mostly hypertrophic as in the metaphysis; they proliferate and fill up the defect. On the guidance of other growth factors, the hypertrophic chondrocytes start to mineralize and differentiate in osteoblasts (enchondral ossification). During enchondral regeneration in the cartilage phase, the defect is softer and more flexible than normal bone.
CELL BIOLOGY AND LINEAGE DESCRIPTION
Osteoblasts
Osteoblasts are the bone-building cells derived from MSCs. Osteoprogenitor cells develop as a result of differentiation of MSCs, and they are essential for maintaining the osteoblastic cell population.16 The Wnt/β-catenin signal pathway plays an important role in the differentiation from osteoprogenitor cells to osteoblasts.17 Osteoprogenitor cells have a spindlelike morphology, whereas osteoblasts have a more quadratic morphology. Differentiated osteoblasts are approximately 20-µm mononuclear cells and are the only cell population in the human body capable of producing unmineralized bone matrix.16
The production of this new bone matrix is only possible when several osteoblasts work together. This can be seen in the histology where the osteoblasts are arranged similar to a pearl necklace on the surface of new bone. These structures are also called osteoids. While building new bone, osteoblasts produce unmineralized bone matrix, which mainly consists of cross-linked collagen and some highly specialized proteins such as osteocalcin and osteopontin. Osteoblasts highly express alkaline phosphatase. This enzyme is important to prepare the secreted proteins for the later mineralization.18
The organic matrix that has been produced by the osteoblasts is subsequently mineralized by the deposition of hydroxyapatite. This starts to occur especially in the grooves of the collagen. Because of the mineralization of the bone matrix, a connective tissue arises, which is typical for the structural strength of the bone. During mineralization of the bone matrix, singular osteoblasts are closed up by the minerals. Then the osteoblasts are differentiated further into osteocytes that cannot produce
any bone matrix anymore. In addition, osteoblasts can differentiate into so-called bone lining cells after the bone matrix has been materialized and they are localized at the surface of the bone. The activity of osteoblasts is regulated by different hormones and growth factors.16 They also play an important role by the activation of osteoclast progenitor cells.
any bone matrix anymore. In addition, osteoblasts can differentiate into so-called bone lining cells after the bone matrix has been materialized and they are localized at the surface of the bone. The activity of osteoblasts is regulated by different hormones and growth factors.16 They also play an important role by the activation of osteoclast progenitor cells.
Osteoclasts
Osteoclasts are derived from mononuclear progenitor cells in the bone marrow. They are approximately 50 to 100 µm in size and are localized at the surface of the bone.19 Osteoclasts are polyploid, and they are the only known cell type to be able to resorb bone. This polyploidy is based on the development of the osteoclasts because they are derived from a fusion of several mononuclear osteoclast progenitor cells. Osteoclasts are able to resorb mineralized bone matrix because of the presence of highly active ion channels (H+-ATPase) in their plasma membranes. With the help of these channels, the osteoclasts can pump protons in the extracellular space and locally massively decrease the pH. The protons stay local because when the osteoclasts have close contact with the bone matrix, the actin skeleton reorganizes and a sealing zone with the contact border occurs. The interaction between osteoclasts and the surface of the bone is mainly organized via integrins. The resorption spaces are called Howship lacunae. The highly acidic environment dissolves the bone mineral.
In addition to the secretion of protons, osteoclasts also produce hydrolytic enzymes and procollagenases. Those enzymes digest the organic bone matrix. Osteoclasts are activated by two main soluble proteins, receptor activator of nuclear factor kappa B ligand and macrophage colony-stimulating factor.20
Osteocytes
Osteocytes are the main cell type in the mineralized bone matrix. Osteocytes are connected to each other through protrusions of the plasma membrane. Via these protrusions, the osteocytes communicate both with each other and with the surrounding microenvironment. Osteocytes are the mechanosensory cells in the bone. Depending on the mechanical environment, they regulate when and where resorption or bone construction will take place.
Osteoprogenitor Cells Including MSCs
As stated previously, osteoprogenitor cells are derived from MSCs. MSCs can be found in almost all tissues of the body. In bone tissue, MSCs are found in the bone marrow. Adult bone marrow MSCs can differentiate into various cell types and form new tissue including bone. Because they are located in the bone niche, it is claimed that this is the most optimal source for the regeneration of bone. There are approximately 30 MSCs/mL of bone marrow. They belong to the nucleated cells present in the bone marrow, which are approximately 25 × 103/µL. MSCs are characterized by the markers CD73, CD90, and CD105. They lack the markers CD45 and CD34.21
MSCs can be isolated from the bone marrow and used for therapeutic purposes as explained later. Depending on the environment including the mechanical stresses and the humoral factors present, MSCs differentiate in a certain cell type among other osteoblasts. Interestingly, it could be shown that MSCs from older patients can be earlier and more pronounced and be differentiated to osteoblasts in vitro than the ones obtained from younger patients. However, over time, MSCs from older patients lose activity and become senescent.
In addition to the regenerative capacity of MSCs, they also play an important role in immunomodulation. MSCs are able to modulate the activity of immune cells. They have the ability to suppress T-cell activity both in a cellular and humoral-dependent way.22
EXTRACELLULAR MATRIX COMPOSITION AND HOMEOSTASIS
Collagen Composition and Calcium Deposition
As stated previously, approximately 90% of the organic bone matrix consists of collagen.16 The main collagen type in the bone is collagen type I. Collagen consists of a triple helix of different alpha helices. In bone, mainly two alpha-1 helices and one alpha-2 helix are combined. Collagen is produced as a pre-procollagen, and one of the main features is the presence of hydroxylated proline and lysine. This hydroxylation is vitamin C dependent. Selected hydroxylysines can then be glycosylated. A procollagen triple helix is formed and subsequently secreted to the extracellular space where the propeptides are cleaved. There they are self-assembled into a fibril of approximately 10 to 300 nm in diameter. These collagen fibrils are then aggregated to form a collagen fiber with a diameter of approximately 0.5 to 3 µm. Collagen type I is the so-called fibrillar collagen.23
The amino acid sequence of collagen shows typical triplets of glycine-proline-amino acid or glycine-amino acid-hydroxyproline. The glycine in the collagen is important for the stabilization of the collagen fibers so that the single fibril can be brought closer together because it allows for intermolecular cross-linking. Hydroxyproline is important for the thermal stability of the triple helices. This may be partially based on hydrogen bonds.
The presence of these amino acids also enables the formation of curves in the fibrils. This leads to the presence of minor and major grooves. Together with the charge of the specific amino acids, these are the exact localizations where calcium and phosphate can be deposited. The collagen fibril diameter is probably also important for the calcium-to-phosphate ratio. This is important for the bone strength and is also related to pathologic circumstances such as osteoporosis and osteogenesis imperfecta.
Calcium Homeostasis
As mentioned previously, one of the main inorganic components of the bone is hydroxyapatite. This is a combination of calcium and phosphate. Approximately 99% of the calcium in the human body is stored in bone. The rest of the calcium can be found intracellularly and in the serum. There, it is highly important to be regulated in a very strict range. Therefore, calcium homeostasis is important. The term calcium homeostasis is mainly indicating the hormonal regulation of the serum calcium levels.
There are three important hormones that play a role: PTH (from the parathyroid gland), calcitriol (1,25-dihydroxyvitamin D), and calcitonin from the thyroid gland. In case the serum calcium levels are diminished, the thyroid gland starts to secrete PTH. PTH induces the production of calcitriol. In bone tissue, PTH induces bone resorption because of the activation of osteoclasts and the inhibition of osteoblasts. The secreted calcitriol stimulates calcium absorption in the gut and kidneys. In the bone, it further activates osteoclasts. Nevertheless, calcitriol also stimulates the mineralization of the osteoid. Calcitonin induces opposite reactions and thus more calcium deposition in bone tissue.
It is clear from the aforementioned information that the calcium balance is highly important for the amount of calcium in bone tissue. When people get older and especially in postmenopausal women due to a shortage of estrogen, this balance is disturbed and the calcium deposition in the bone is gradually reduced. This leads to a reduction in bone mass and bone strength. This is of course also dependent on the nutritional state.
OVERVIEW OF THERAPEUTIC OPTIONS
As previously described, it is clear that bone is an intricate tissue composed of several components. In pathologic situations in the orthopaedic and trauma field, bone can be lost, bone defects can occur, and then it needs to be regenerated. The components as described previously are taken into account, meaning that for treatment, the matrix can be considered in the form of biomaterials. In addition, cells can be used, and finally stimulatory molecules, the so-called morphogens, can be included in therapeutic approaches. Thereby, these components can be used separately or in combination (Figure 1).
Biomaterials
Biomaterials are materials used for biologic applications and can be of different origin, namely, natural, inorganic, and synthetic. They can be applied in different forms such as gels, powder, blocks, and using biofabrication techniques.
Natural Materials
Autologous bone grafts are still the gold standard for bone replacement therapy. The osteogenic properties are associated with the osteogenic progenitor cells and osteoblasts found in the graft. However, graft removal techniques and graft preparation can affect osteogenic properties. This is problematic mainly because of the risk of osteonecrosis. Therefore, it is necessary to carefully remove the grafts and use the correct implantation techniques. Furthermore, the osteoconductive properties are associated with the occurrence of growth factors in the graft. Some growth factors are known to exist in fresh autologous grafts.24 Notably, bone morphogenetic protein (BMP)-2 and BMP-4, fibroblast growth factor, vascular endothelial growth factor, platelet-derived growth factor, and insulinlike growth factor I can be found. In addition, the osteoconductive properties depend on the three-dimensional structure of the graft. These determine the speed of bone integration.25 For example, spongiosa grafts are usually incorporated much faster than cortical grafts because of their porous structure.26
Another form of the autologous bone graft is the so-called reamer-irrigator-aspirator (RIA). This system is derived from the normal reamer system for reaming of
long bones to insert an intramedullary nail. Because this procedure could lead to pulmonary embolism, an additional suction was installed. The suction material contains bone marrow and bone dust from the cortex. By inserting a sieve system with a container, both the bone marrow and bone dust can be collected. That means a mixture of osteoprogenitor cells, growth factors, and bone extracellular matrix is obtained. Removing it from the container, a type of putty material is obtained with a volume of approximately 25 to 90 cm3. When it was implanted in an ovine bone healing model, accelerated regeneration was observed. Therefore, this RIA material is an excellent material to fill bone defects because of osteotomy or during the second stage of the Masquelet procedure.
long bones to insert an intramedullary nail. Because this procedure could lead to pulmonary embolism, an additional suction was installed. The suction material contains bone marrow and bone dust from the cortex. By inserting a sieve system with a container, both the bone marrow and bone dust can be collected. That means a mixture of osteoprogenitor cells, growth factors, and bone extracellular matrix is obtained. Removing it from the container, a type of putty material is obtained with a volume of approximately 25 to 90 cm3. When it was implanted in an ovine bone healing model, accelerated regeneration was observed. Therefore, this RIA material is an excellent material to fill bone defects because of osteotomy or during the second stage of the Masquelet procedure.
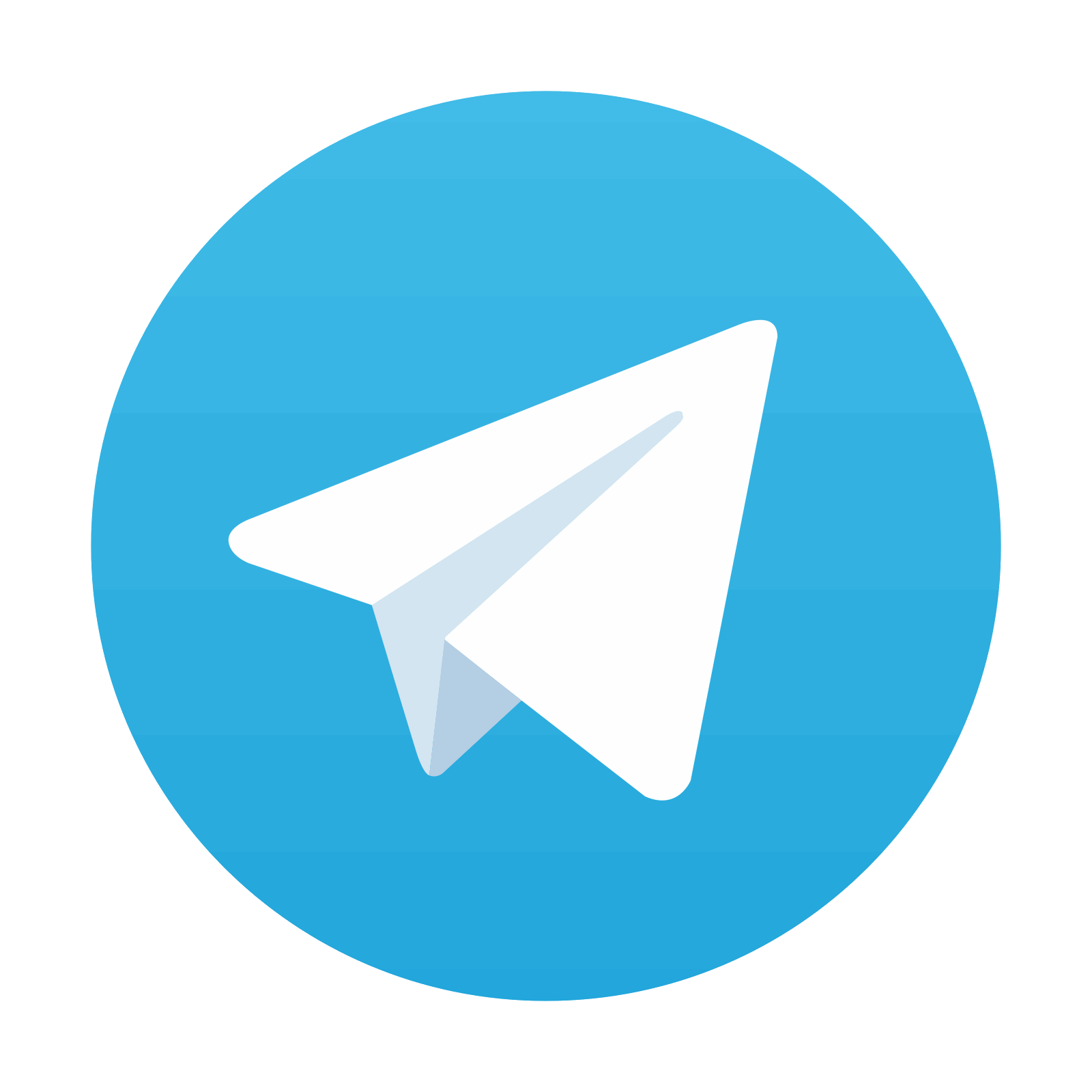
Stay updated, free articles. Join our Telegram channel

Full access? Get Clinical Tree
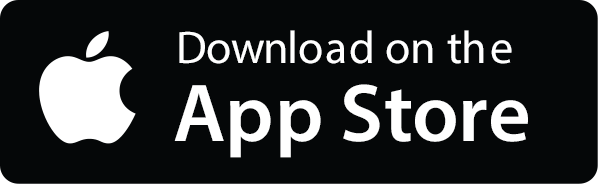
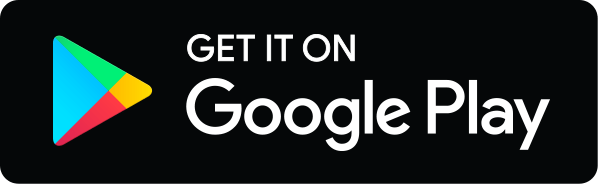
