Drug class
Mechanism of action
Effect on bone
Medication concerns
Anticoagulants
Heparin
Both standard and low MW heparin increase bone resorption by reducing osteoprogenetin and favoring RANKL-induced osteoclast differentiation, as well as inhibiting osteoblast differentiation. The result is decreased bone formation
Significant decrease
A newer oral anticoagulant, fondaparinux, has rapid onset; reversibility is not a concern
Low MW heparin
Moderate decrease
Warfarin
Decreases the γ-carboxylation of and calcium-binding properties of osteocalcin, which is involved in bone formation
Mild-moderate decrease
HMG-CoA reductase inhibitors
Atorvastatin, lovastatin, rosuvastatin, simvastatin
Decreases mevalonate, which indirectly increases osteoblast differentiations
Suggested BMD increases and fractures decrease
N/A
Diuretics
Thiazides (hydrochlorothiazide, chlorthalidone)
Decreases renal Ca excretion
Promote bone building
Thiazides and K+ sparing preferred over loop diuretics if clinically acceptable for patient
Potassium sparing agents (spironolactone, Aldactone)
Decreases renal Ca excretion, Increases K stores, Decreases aldosterone-mediated bone loss
Increases bone building
Loop diuretics
Increases renal Ca excretion
Increases bone resorption
Beta blockers
Nebivolol
B-Adrenergic receptor inhibition
Likely a positive effect on bone
Evidence insufficient to warrant any specific agent over another
Induces NO release which may be protective in bone preservation and prevention of osteoporosis
Atenolol
No specific action on bone cells delineated
Majority of larger studies indicate a positive effect on bone
Metoprolol
Nitrates
NO stimulation of osteoblastic activation
Likely a positive effect on bone
N/A
Calcium channel blockers
Studies suggest decreases osteoclast function
Decreases risk of fractures
N/A
ACE inhibitors
Angiotensin action on osteoblasts or calcium metabolism
Appear to increase fractures; neutral effect on bone
Consider other antihypertensive if clinically acceptable for patient
Angiotensin receptor blockers
Possible action at the angiotensin receptor
May inhibit bone resorption
N/A
Epidemiology of Bone Loss in Persons with Cardiac Disease
The risk factors and prevalence of bone loss in persons with advanced heart disease are difficult to estimate, since risks are closely dependent on the extent of disease, age of the individual patient or groups of patients, medications, comorbidities, and lifestyle. A similar statement could apply to risk of fractures, since syncopal events and hypotension as well as new medications or changes in doses of existing medications can substantially increase the risk of falls. Table 2 summarizes the findings of a cross section of studies in the last 15 years, looking at a variety of populations.
Table 2
Presence of osteoporosis in patients with cardiac conditions
Population studied/source | N control subjects | N experimental subjects | Outcome in control subjects | Osteopenia or osteoporosis in experimental subjects | Special notes |
---|---|---|---|---|---|
Aortic valve calcification (AVC) subjects (Aksoy et al. [12]) | 65 (AVC–) | 49 (AVC+) | 60 % with osteopenia or osteoporosis by BMD in L spine L1–4 | 82 % osteopenia or osteoporosis, by BMD in L spine L1–4 | Presence of hypertension and age in AVC+ subjects |
Postmenopausal sample of population-based females being screened for osteoporosis, with/without CAD (Barengolts et al. [17]) | 11 with normal BMD | 20 with osteopenia and 14 with osteoporosis by DXA | Coronary calcium score in all arteries 41.9 ± 83.1, in LAD 30.2 ± 69.1 | Coronary calcium score in all arteries for osteopenia 115.1 ± 182, in LAD 68,9 ± 115 and for osteoporosis was 221.7 ± 54.3 for all arteries and 166.6 ± 243.6 for LAD | Osteoporosis based on BMD at L spine, total hip FN, or Ward’s triangle. Overall prevalence OP 31 %, calcium deposits 76 % |
Men and women with NYHA Class III or IV CHF (Shane et al. [7]) | Study sample with unknown bone disease but known CHF | N 101 total with 79 men and 22 women, | N/A | Osteopenia in 43 % at L spine, 47 % at total hip, and 42 % at FN. Osteoporosis in 7 % L spine, 6 % total hip, and 19 % FN | Osteopenia and osteoporosis present in approximately 50 % sample in either spine hip or both locations |
Men with and without CHF screened for presence of osteoporosis (Terrovitis et al. [10]) | 13 male patients without CHF | 60 male patients with CHF | Absence of osteopenia or osteoporosis on average in control group | T-score BMD in femur for Class I/II-1.1 and for Class II/IV patients was −1.9 | Both classes had significant differences in BMD from controls |
In patients with advanced degrees of heart failure who are awaiting a transplant, evidence suggests a correlation between trabecular bone loss and time of evolution of the disease prior to transplant [40]. Garcia-Delgado et al. identified an increase in markers of bone resorption as well as decreased bone mass at lumbar and hip sites among patients before cardiac transplantation. Waiting time for the transplant was the most important predictive factor in bone loss at the lumbar spine, and as the next chapter will discuss, patients with recent organ transplants experience an even greater degrees of bone loss.
Treatment of Bone Loss in Cardiac Conditions
Given that osteoporosis occurs in 23 % of patients with heart failure pretransplant [41] and that medications given posttransplant (corticosteroids, immunosuppressants) contribute to further bone loss, preventative efforts and early treatment should be initiated prior to reaching the stage of organ transplantation [42].
For patients with heart failure on cardiac medications, particularly antihypertensive agents, selection of a non-loop diuretic such as spironolactone or one of the thiazides is favored over agents such as furosemide. In terms of effect on bone, data on ACE inhibitors is mixed, but if other equally suitable options for treatment of hypertension exist, then such agents should be considered. As with many other conditions, optimizing calcium and vitamin D stores is advisable in high-risk patients. Shane et al. [7] found that in two groups of heart failure patients, those with more severe conditions, requiring ongoing hospitalization for intravenous inotropic agents or a management of left ventricular assistive device (LVAD), had significantly lower stores of serum vitamin D25-OH and notably reduced levels of the active form of vitamin D 1,25-(OH)2. In contrast, the comparison group of heart failure outpatients had modestly deficient levels of vitamin D titers. Oral supplementation and, if possible, brief outdoor exposure to sunlight are advised if patients can tolerate these interventions.
More severely involved heart failure patients would have reduced exercise tolerance and limited community mobility, further contributing to osteoporosis. For patients with advanced CHF, including those with LVADs, lower extremity strengthening, weight-bearing exercises such as walking, and aerobic conditioning are all acceptable forms of exercise after VAD implantation [43]. Moreover, they lessen the severity of proximal muscles and osteoporosis which often occur in the transplant population.
Pulmonary Conditions and Osteoporosis
Advanced pulmonary disease most commonly occurs in the form of chronic obstructive pulmonary disease (COPD) including emphysema and chronic bronchitis and cystic fibrosis (CF). While other forms of restrictive pulmonary disease lead to organ failure, this section will focus primarily on the effects of COPD and cystic fibrosis on osteoporosis. Given their high prevalence in the elderly population coupled with the frequency of osteoporosis in the same age group, it is not surprising that more than 60 % of COPD patients in a recent study had osteopenia and 29 % met the criteria for osteoporosis [44]. For CF, a prevalence study of 103 patients aged 16–53 indicated that 10 % had osteoporosis and another 36 % had osteopenia [45]. Because the last three decades have seen CF life expectancies rise to a median age of nearly 40 years, those treating patients with the disease are just now seeing how bone health is affected by years of compromised pulmonary function, multiple infections, and malabsorption of nutrients [46].
Epidemiology of Osteoporosis and COPD
The prevalence of osteoporosis in COPD generally varies between 21 % and 59 % depending upon the diagnostic methods used, the age and gender of the sample, and the duration and severity of COPD in a study group [47, 48]. The few studies that demonstrated prevalence under 20 % used either quantitative ultrasound in part for the diagnosis, which is not the standard of care, or included subjects with obstructive airflow disease who had not met the definition of “chronic” in terms of disease duration. Although COPD is the third leading cause of death in the United States [49], it is not considered a risk factor for osteoporosis by the American College of Physicians, nor is smoking, despite its close association with COPD [50]. In contrast, the FRAX tool for prediction of fractures includes COPD but not smoking. Selected pulmonary parameters, inactivity, steroids, and other medications are among the risk factors for osteoporosis in those with COPD.
In their 2011 review of a number of studies involving samples of 40 to over 100 subjects, Lehouck et al. [47] found that osteoporosis occurred to a greater degree in subjects with lower forced expiatory volume in 1 second (FEV1) scores and in those with advanced disease who are awaiting organ transplant [51]. In a study by Silva et al. [52] in which 42 % of subjects with COPD were osteoporotic and another 42 % were osteopenic, a significant correlation was found between femoral neck T-score and body mass index (BMI), with a significant inverse relationship between femoral neck T-score and BODE, a combined value including body mass index, air flow obstruction, dyspnea, and exercise capacity. In addition, correlations were found between a DXA T-score and FEV1, forced vital capacity (FVC), and percent of predicted diffusing capacity of the lung for carbon monoxide. The severity and rate of progression of COPD would have a marked influence on maintaining BMD.
By contrast, a 2011 study by Graat-Verboom et al. [53] examining 255 outpatients with stable COPD revealed an astonishing 51 % had osteoporosis defined by a combination of spinal x-rays and DXA. A summary of recent studies on prevalence rates is given in Table 3.
Table 3
Prevalence of osteoporosis and vertebral compression fracture in COPD
Condition Author/source | Patient group | Subjects | BMD measurements | Prevalence of osteoporosis, % | RX diagnosis of VCF | Prevalence of VCF, % |
---|---|---|---|---|---|---|
Osteoporosis | ||||||
Graat-Verboom et al. [54] | COPD patients (GOLD I–IV) referred for PR | 554 | Whole-body BMD (DXA) | 21 | … | … |
Ferguson et al. [55] | COPD patients (GOLD II–IV) | 658 | BMD LS and hip (DXA) | 24 | … | … |
Sin et al. [56] | COPD patients (GOLD I–IV) | 5215 | BMD total femur (DXA) | 4–33 | … | … |
Førli et al. [51] | COPD patients awaiting LTX | 40 | BMD LS and FM (DXA) | 59 | … | … |
Iqbal et al. [57] | Patients with chronic lung disease | 130 | BMD LS and hip (DXA) | 36 | … | … |
Sabit et al. [58] | COPD patients (GOLD I–IV) | 75 | BMD LS and hip (DXA) | 24 | … | … |
Bolton et al. [59] | Respiratory outpatients referred for PR | 81 | BMD total body, LS, and hip (DXA) | 32 | … | … |
Compression fractures | ||||||
Jørgensen et al. [60] | Ambulatory COPD outpatients (GOLD III–IV) | 62 | … | … | Thoracic and lumbar spine radiographs | 24 |
Nuti et al. [61] | Ambulatory COPD outpatients (GOLD I–IV) | 2981 | … | … | Lateral chest radiograph | 41 |
Papaioannou et al. [62] | COPD patients (GOLD not reported) | 127 | … | … | Lateral chest radiograph | 27 |
McEvoy et al. [63] | Male COPD patients (GOLD not reported) | 312 | … | … | Lateral lumbar and thoracic radiograph | 49–63 |
Graat-Verboom et al. [53] | Ambulatory COPD outpatients (GOLD I–IV) | 255 | BMD LS, and hip (DXA) | 51.4 | Lumbar, lateral chest, and thoracic radiograph | 36.5 |
The Global Initiative for Chronic Obstructive Lung Disease (GOLD) has defined four levels of COPD severity, ranked I–IV, the end stage [64]. GOLD rankings take into account extrapulmonary manifestations of COPD, one of which is osteoporosis.
Among the most consistent risk factors for osteoporosis in the COPD population is the prolonged use of oral corticosteroids or glucocorticoids (GCs). An extensive review by van Staa et al. [65] demonstrates that the total cumulative dose of corticosteroids is inversely related to BMD. Although this study was not specifically focused on COPD patients, it does illustrate the substantial risk that COPD patients face in terms of maintaining bone health with cumulative years of GCs use.
The risk of developing osteoporosis with inhaled corticosteroids (ICS), as opposed to oral or intravenous corticosteroids (ICS), appears to be low based on a meta-analysis by Drummond et al. [66] who observed no significant difference in BMD among those COPD subjects using and not using ICS. Similarly, the TORCH trial (Towards a Revolution in COPD Health) [55], involving 658 patients with COPD, found minimal decreases in BMD (1.7–3.2 %) with use of ICS therapy in the first three years. However, the risk for fractures with ICS is increased, according to a number of investigations described in the following section.
Epidemiology of Fractures in COPD
As some investigations on COPD patients demonstrate, BMD assessed by DXA measures only bone density, not bone microarchitecture and bone quality. However, the use of DXA as a measure of BMD to determine fracture risk may not be ideal in some settings. Quantitative CT (QCT), an alternative method of assessment, was employed in a large study (n = 3317) dealing with the effect of smoking on both osteoporosis and vertebral fracture risks in patients, with and without COPD [66]. In a smaller companion sample of 111 subjects taken from this larger group, Jaramillo et al. [67] applied both QCT and standard DXA tests, finding that QCT identified more subjects as osteopenic or osteoporotic than did DXA and that these subjects had a greater number of fractures. In this sample, 6 % of the QCT-classified osteopenic subjects and 37 % of those classified as osteoporotic had vertebral fractures. More broadly, the trial concluded that COPD was independently associated with not only low volumetric bone density, after adjusting for race, BMI, smoking, steroid use, and age, but also with an increased prevalence of vertebral fractures, with the highest number of fractures between T6-12, a region that is perhaps better evaluated by quantitative CT than DXA.
In a further analysis of ICS in COPD, Loke et al. [68] evaluated 16 randomized controlled trials (RCTs) and 14 observational studies relating to the use of two different ICS, fluticasone or budesonide, for at least 90 weeks. Findings demonstrated an increased odds ratio (OR) for fractures of 1.27 for all studies and 1.19 for the four trials of greatest duration (three years). The preceding meta-analysis evaluated risks of vertebral and nonvertebral fractures, with evidence demonstrating ICS were associated with both types of fractures. However, no comparison of likelihood of one type of fracture versus another was possible, since the studies used different combinations of ICS or different solo agents.
Clinicians often balance the benefits of steroids for prevention and management of COPD exacerbations against the deleterious effects on other organ systems including bone health. Yet even very low daily doses can have deleterious effects: 2.5 mg/day presents a modest increased risk of fractures, while amounts greater than 7.5 mg/day confer a fivefold increased fracture risk [65, 69]. However, the risk of fractures does dissipate with discontinuation of steroids.
Etiology and Pathogenesis
Many different elements—physiologic, environmental, and pharmacological—contribute to osteoporosis in those with COPD and emphysema. Chronic hypoxemia, chronic inflammation, hypogonadism, dietary deficiencies in vitamin D and calcium, chronic use of corticosteroids, and other disease-modifying agents reduced physical activity and contributed to a sedentary lifestyle [70]. Figure 1 illustrates the risk factors for osteoporosis and functional consequences [47].


Fig. 1
Risk factors for osteoporosis in COPD and functional consequences (Source: Lehouck et al. [47]. Used with permission)
Liang and Feng [44] have demonstrated a relationship between systemic inflammation of low to moderate grades and low BMD in relatively stable COPD patients. It is known that the inflammatory cytokines, IL-6 and TNF alpha, induce expression of RANKL and RANKL-mediated bone resorption. Chronically elevated stores of these cytokines were found to be associated significantly with low BMD (p = 0.023 and 0.010, respectively) as was the level of C-reactive protein (CRP), falling just short of statistical significance (p = 0.062). These findings held true after adjustment for age, gender, use of inhaled corticosteroids, and severity of airway obstruction. States of chronic inflammation also suppress the WNT/β-catenin signaling pathway that stimulates osteoblasts, thereby contributing to the development of osteoporosis [71].
As in other conditions of inflammation including rheumatic disorders, the use of glucocorticoids (aka corticosteroids) increases expression of RANKL and decreases osteoprotegerin (OPG) [72]. In addition to reducing apoptosis of osteoclasts which resorb bone, enhanced bone resorption occurs, with subsequent inhibition of production and maturation of osteoblasts. These combined effects result in a net loss of bone with ongoing resorption and prolonged inhibition of bone formation. Initial use of GCs adversely alters trabecular bone but prolonged inflammation can also affect cortical bone.
Low vitamin D also compromises optimal absorption of calcium from the gut and influences immature osteoblastic cells to stimulate RANKL which, in turn, stimulates osteoclasts to promote bone resorption. In addition to exerting a direct influence on cells involved in bone metabolism, adequate serum levels vitamin D help to maintain muscle strength, thereby reducing the risk of falls [73–75]. An estimated 20–30 % of patients with COPD have reduced limb muscle strength relative to healthy controls [76].
Finally a mechanical abnormality related to altered lung structure and involving air trapping and parenchymal destruction may account for a substantial portion of bone loss, based on evidence that lung volume reduction surgery in advanced emphysematous patients significantly improves BMD in patients one year after undergoing this procedure [77].
Nonpharmacologic Treatment of COPD and Osteoporosis
Optimizing Function Prior to Formal Exercise
As stated above, evidence suggests lung volume reduction surgery has a positive effect on BMD as opposed to other surgical interventions which confer no direct, osteoporotic benefit with COPD. Optimizing pulmonary function prior to pulmonary rehabilitation can be valuable. Blocking or decreasing exacerbations of COPD with inhaled anticholinergics, such as ipratropium or tiotropium as well as short acting beta-2 agonists such as albuterol, helps with dyspneic symptoms and can indirectly improve pulmonary endurance for participation in aerobic exercise including weight-bearing activities. If any component of airway hyperactivity and bronchospasm exists, mast-cell stabilizers such as cromolyn sodium can alleviate airway inflammation which limits exercise tolerance [78].
Supplemental oxygen can improve endurance during physical therapy and aerobic activities by reducing shortness of breath. Perceived exertional activity during aerobic conditioning improves cognitive function, which is critical in settings where fall risk exists and attention to safety is imperative. Unless there is documented reduction in oxygen saturations with exercise, supplemental oxygen is unlikely to ameliorate function. Improving breathing with diaphragmatic and segmental breathing techniques can increase tidal volume and maximize oxygen uptake with reduced effort (Figs. 2 and 3) [79]. Pursed lip breathing prevents air trapping and small airway collapse, improving gas exchange, while it also reduces dyspnea and the work of breathing [80]. The combination of diaphragmatic and pursed lip breathing leads to higher arterial blood gas numbers, signifying improved oxygen supply to muscles and key organs. In this manner, exercise endurance is enhanced, and overall sense of fatigue is lessened.



Fig. 2
Illustration of diaphragmatic breathing. Diaphragmatic breathing is appropriate for COPD patients who demonstrate predominantly upper thoracic expansion but limited abdominal movement or with inward movement of the lower rib cage during inspiration. This technique may be difficult to learn and is most easily performed, at least initially, in the semi-Fowler or side-lying position. Once mastered, the therapist should progress to the sitting position, followed by standing, and finally walking and stair climbing (Source: http://www.slideshare.net/sharminsusiwala22/a-detailed-description-on-breathing-exercises)

Fig. 3
Illustration of segmental breathing. Segmental breathing involves localized expansion exercises used to direct airflow to specific regions. This technique is often used in hypoventilation states as with painful conditions and muscle guarding in which patients self-limit their thoracic expansion. This type of scenario may occur following vertebral compression fractures; spine surgery for traumatic or insufficiency fractures (which have less pain than traumatic fractures); kyphoplasty; post thoracotomy, trauma to the chest wall, post-chest radiation fibrosis, pneumonia, and postmastectomy scars (Sources: For illustration and text above, see http://www.slideshare.net/sharminsusiwala22/a-detailed-description-on-breathing-exercises. See also Garritan [80])
Optimizing Nutrition: Vitamin D and Calcium
Because most patients with COPD tend to be older and many have comorbidities, standard supplementation with oral vitamin D3 on the order of 800 IU daily is advised for all those with normal to near normal serum vitamin D25OH levels [47]. Many will have difficulty simply maintaining therapeutic levels of vitamin D without a daily dose of a supplement. At the same time, several researchers advise the administration of high potency vitamin D to any COPD patient with levels <10 ng/ml, because inadequate levels of the active form of vitamin D 1,25 (OH)2 D can adversely affect inflammation, thus exacerbating other comorbidities found in COPD [81]. Ensuring a diet adequate in calcium intake is also essential. For those patients who are lactose intolerant or who consume few products rich in calcium, oral supplementation is recommended.
Physical and Occupational Therapy in COPD
Formalized therapy in those with COPD and osteoporosis must be tempered by continual recognition of the need to improve ventilation and reduce the work of breathing [82]. Ideally, patients should start with 5 minutes sessions but work up to 15 minutes of resistive training pulmonary exercises daily. Focusing on activities of daily living, they generally begin with reconditioning exercises which encompass upper extremity range of motion and gentle resistance exercises with arm elevation as well as pool or bicycle routines [83]. Although none of these workouts involve weight-bearing through the spine or lower extremities, less intense exercise may initially be required to build adequate core strength before the more challenging activities of walking or stair climbing can be undertaken safely. Alternating walking with unsupported upper extremity exercise produces a safe combination of weight-bearing and non-weight-bearing exercise in keeping with the standard pulmonary rehabilitation protocol.
An individually prescribed exercise program, incorporating devices such as inspiratory orifices, should include a warm-up, followed by a time of gradually increasing physical exercise, and then a cooldown. Ideally, a pulmonary rehabilitation therapist working in conjunction with a patient’s pulmonologist or internist should be involved in the design and initial intensity of a given patient’s program. To maximize blood flow to lungs and avoid splanchnic diversion of oxyhemoglobin, exercise should not be initiated for at least 90–120 minutes after eating. In addition, nutritional selection, especially prior to therapy sessions, is important, since high carbohydrate meals produce the most carbon dioxide for the amount of oxygen used, whereas metabolism of fat produces the least carbon dioxide expenditure [84].
All programs should include a daily 12 minutes walk, with the distance recorded in order to monitor progress and estimate future exercise tolerance. Rest breaks are essential in the early and, depending on the severity of COPD, the later phases of pulmonary rehabilitation. Overexertion and pulmonary fatigue can compromise gas exchange and lead to hypercapnia. Arterial blood gases may need to be monitored. Ideally heart rate should not increase more than 20 % from baseline in initial phases of pulmonary rehabilitation reconditioning, and no more than 30 % in patients who are well advanced in the therapy program. Warning signals to discontinue or suspend therapy temporarily include heart rate >125, oxygen saturations below 91 %, or greater than 13 on the Borg perceived exertion scale (Table 4) [85]. If oxygen saturations do not improve despite supplemental oxygen or if any EKG changes involving premature ventricular contractions (PVCs) appear, therapy for the day should be suspended, and physicians should alter the plan of care to lessen intensity.
Table 4
Borg perceived exertional scale
How you might describe your exertion | Borg rating of your exertion | Examples (for most adults <65 years old) |
---|---|---|
None | 6 | Reading a book, watching television |
Very, very light | 7–8 | Tying shoes |
Very light | 9–10 | Chores like folding clothes that seem to take little effort |
Fairly light | 11–12 | Walking through the grocery store or other activities that require some effort but not enough to speed up your breathing |
Somewhat hard | 13–14 | Brisk walking or other activities that require moderate effort and speed your heart rate and breathing but don’t make you out of breath |
Hard | 15–16 | Bicycling, swimming, or other activities that take vigorous effort and get the heart pounding and make breathing very fast |
Very hard | 17–18 | The highest level of activity you can sustain |
Very, very hard | 19–20 | A finishing kick in a race or other burst of activity that you can’t maintain for long |
Pharmacologic Intervention
Antiresorptive Agents
In terms of medications that directly address osteoporosis, bisphosphonates have the longest follow-up evidence, demonstrating their ability to protect against postmenopausal and glucocorticoid-induced osteoporosis. The monoclonal antibody, denosumab, is effective in these conditions as well but has not been specifically studied in COPD. In a major clinical trial examining the effect of alendronate (a once weekly oral bisphosphonate) on BMD, Smith et al. [86] used a daily 10 mg oral dose of the drug with 600 mg oral calcium supplementation for 12 months in subjects with established osteoporosis (T-score, –2.5 by DXA). Compared to controls who received a placebo with 600 mg daily elemental calcium, subjects given alendronate with calcium demonstrated significant improvement in BMD of the lumbar spine segments L2-4 but no improvement in the femoral neck or total hip.
Given the risks of osteoporosis in persons with COPD, the American College of Rheumatology has recommended that patients whose DXA scans are in the osteopenic range (T-score below –1) and who regularly use glucocorticoids be offered treatment in the form of antiresorptive agents, even in the absence of initial fractures. These recommendations published in 2001 predate the release of PTH and so do not address how its use might fit into the guidelines, now over a decade old [87]. Newer recommendations in 2010 place an emphasis on dosing of glucocorticoids and selection of bisphosphonate and in some cases teriparatide, although the latter agent receives a lower level of support than bisphosphonates, mainly based on the evidence published up to that time (2010, when evidence was just building regarding this medication) [88]. Imaging via DXA is incorporated in other tools of determination such as the FRAX score, which is used in the revised model. The guidelines suggest a number of limitations with reliance on FRAX, since it uses only the BMD at the hip rather than the spine, where many glucocorticoid-induced fractures are seen.
Additional guidance on approaches to treatment in high-risk patients such as those with COPD, and the timing of monitoring clinical response to treatment is needed in light of recent pharmacologic advances, radiographic imaging, and biological markers of response to interventions.
Cystic Fibrosis
Epidemiology of Cystic Fibrosis, Osteoporosis, and Fracture
Cystic fibrosis (CF) is an autosomal recessive disorder that affects approximately 30,000 people in the United States and a somewhat higher number throughout the rest of the world [89]. The most common lethal genetic disease in the Caucasian population, it affects the protein known as cystic fibrosis transmembrane conductance regulator (CFTR) which controls the movement of sodium, chloride, and water in and out of cells, predominantly in the lungs but also in the pancreas, digestive system, and liver. With too little or abnormal CFTR, the mucus secreted in these areas becomes thickened, causing obstructions, infection, and loss of function [90].
Generally diagnosed by age two, half of patients with CF recorded in 2014 were, for the first time, over the age of 18, with a survival rate of 39.3 years compared with 29.2 years in 1986. Many patients live well into their 40s and 50s [91]. Advancements in newborn screening programs, leading-edge therapeutic options, and improved management have all contributed to rising life expectancy; however, that lengthened life span has led to an increased occurrence of age-related comorbidities, including CF-associated osteoporosis [92].
As Gore et al. have observed [93], decreased BMD and higher fracture rates occur at a young age, 30 years earlier than in a non-CF population; they increase with age, the severity of the disease, and the use of corticosteroids. Beginning with the 1979 Mischler study [94], subsequent trials have corroborated the finding that CF patients have a demonstrated low BMD. Whereas Mischler used direct photon absorptiometry to find that 44 % of 27 patients (ages 5–24) had significant bone deficiency, Conway et al. [95] employed densitometry and multiple indices of disease severity to determine that, in a sample of 114 adolescents and adults, 79 % of 53 men and 56 % of 61 women had osteopenia or osteoporosis at one or more sites. They further documented a clear relationship between low BMD and disease severity as well as between reduced BMD and corticosteroid use. In terms of bone mineralization, 26 % of 66 patients singled out for this complication had pronounced anterior vertebral collapse, resulting in rib and thoracic compression fractures that restrict both coughing efficiency and the ability to undertake chest physiotherapy.
Further research confirms the marked prevalence of osteoporosis and fractures in patients with CF. In a study involving 50 clinically stable CF adults with 53 controls, Aris et al. [96] demonstrated that the CF cohort experienced accelerated bone breakdown without a compensatory increase in bone formation, suggesting that such factors as inflammatory cytokines and PTH increase osteoclast activity and play a role in CF bone disease [96]. In comparison with age-matched controls, patients with both CF and osteoporosis have a 100-fold increased risk of vertebral fractures and a tenfold increased risk of rib fractures [97]. In a 2010 meta-analysis of young adults with CF (mean age = 18.5–32 years), the prevalence of vertebral fractures varied between 5.0 % and 31.0 %, and the prevalence of nonvertebral fractures was as high as 20–40 %, but it could not be determined whether these fractures were truly osteoporotic [98]. As Goalski and Aris [99] have observed, no association between BMD and fractures has been specifically proved in CF studies. However, the link is so strong outside of CF that it dictates treatment.
Finally, a recent study of areal bone density comparing a cohort of CF adults (ages 18–50) who were evaluated in 1995–1999 (historic) with a comparable cohort examined in 2011–2013 (current) has produced unanticipated, disturbing results [100]. Despite advances in care management and heightened life expectancy, areal BMD was no better in the current cohort than it was 15 years ago. However, the present-day cohort did evidence improvements in pulmonary function, vitamin D deficiency, and secondary hyperparathyroidism. The underlying factors contributing to this static state include difficulty achieving nutritional goals, greater intake of systematic and inhaled glucocorticoids, delayed puberty, and CFTR dysfunction.
Pathogenesis of CF
As the occurrence of CF-related bone disease continues to rise with increased life expectancy, the number of age-related risk factors increases concomitantly, including those directly related to osteoporosis as well as those unique to CF, specifically the role of the CF transmembrane conductance regulator. The interaction of these factors leads to an uncoupling of the dynamic status of bone turnover, resulting in decreased bone formation and increased bone resorption [101].
Factors contributing to osteoporosis in CF originate in childhood. In comparison with healthy subjects, those with CF during childhood and adolescence fail to achieve adequate bone mass because of the interaction of delayed puberty, chronic infections, and hormonal imbalance [93]; in addition, the BMD in an age group of 4.9–17.8 years was found to decline at a rapid rate of approximately 1 SD every 6–8 years [102]. A high proportion of CF patients—85–90 %—evidence endocrine pancreatic insufficiency leading to digestive problems and malabsorption of vitamins A, E, K, and D. Both vitamin K and vitamin D levels, so critical to bone formation, are significantly reduced in cystic fibrosis. Whereas vitamin K deficiency is linked to low levels of carboxylated osteocalcin, vitamin D deficiency impairs the ability to achieve peak bone mass and limits calcium absorption by increasing bone turnover through PTH stimulation [103].
In severe CF, acute pulmonary exacerbations are associated with higher levels of cytokines (e.g., tumor necrosis factor-alpha (TNF-α), interleukin (IL)-1, and IL-6) that stimulate osteoclastic bone resorption and cause bone loss [93]. Corticosteroids employed to improve respiratory function in CF are known to adversely affect bone, with oral corticosteroids decreasing BMD in the lumbar spine and femoral neck and inhaled corticosteroids resulting in low total body BMD [95, 104]. High-dose corticosteroid therapy is particularly important following lung transplantation, but it contributes to a rapid decline in BMD associated with increased fracture risk. In contrast to an earlier prevailing belief that osteoporosis with fractures contraindicates lung transplants, most transplant centers now regard osteoporosis as a “remediable comorbidity,” with only uncontrolled pain related to fractures considered to be a contraindication [105, 106].
Specific to osteoporosis in CF, dysfunction of the CFTR has recently been implicated as another factor leading to bone disease. In trials with knockout mice, the existence of an abnormal CFTR protein called delta F509 was linked with osteopenia, reduced cortical bone, and thinning of the trabecular bone [107], while a study of CF adults revealed that delta 509 mutation is an independent risk factor for osteoporosis [108, 109]. In addition, Shead et al. [110] have demonstrated the presence of CFTR in human osteoblasts, osteocytes, and osteoclasts. Implications of these findings require further research.
Diagnosis and Treatment
In accordance with guidelines established by the Cystic Fibrosis Foundation [111], screening for CF-related bone disease should begin in childhood in order to determine the extent of bone density loss and to implement preventative and treatment measures. A baseline BMD determined by standard dual-energy x-ray absorptiometry (DEXA) of the lumbar spine and hip is recommended for all patients at age 18; children ages eight and over should be tested if they evidence risk factors such as previous history of fractures, delayed FEV1 <50 % predicted, and glucocorticoids 5 mg/day or more for a minimum of 90 days/year.
Vitamin Supplementation
Vitamin D and K supplementation as well as supplemental doses of calcium at 1300–1500 mg daily is a first step in both prevention and treatment. To maintain a target level of serum vitamin D25(OH) of 30 mg/mL and optimize calcium metabolism in CF patients, updated guidelines for vitamin D [112] stipulate giving 400–500 IU/day for children 12 months and younger, 800–1000 IU/day for ages 1–10, and 800–2000 IU/day for those 11 years and older. An age-specific intake of 0.3–0.5 mg/day of vitamin K is recommended, based on recent research demonstrating its positive impact on the posttranslational activation of osteocalcin which promotes bone formation and mineralization [113].
Physical Therapy
In CF, physical activity must be tailored to the needs and capabilities of the individual patient. Observational studies of CF children and adults have shown a positive relationship between regular aerobic weight-bearing exercise and BMD, as well as a complementary role in chest physiotherapy. However, controlled intervention trials examining the effect of exercise on bone mass accrual are lacking. Consequently existing evidence is limited to indications that patients with mild to moderate CF are physically able to participate in regular exercise that benefit muscle strength as well as bone and lean mass—if they have sufficient dietary energy intake and motivation [114].
Pharmacologic Intervention
Bisphosphonates, including oral alendronate and risedronate or intravenous pamidronate and zoledronic acid, have been shown to be effective in CF. These agents are recommended in patients with a T/Z scores less than −2.0 and in those with T/Z scores between −1.0 and −2.0 who have a history of fractures, are awaiting a lung transplant or are losing BMD at the rate of >3–5.0 annually [111]. In a Cochrane review of seven small trials, bisphosphonates consistently increased BMD but did not demonstrate fracture reduction or survival benefits [115]. A recent trial involving CF patients aged 5–30 found that BMD increased by 16.3 % in patients receiving alendronate for one year as opposed to 3.1 % for patients on placebo; moreover the patients on alendronate achieved a normal-for-age BMD Z-score after one year. Adverse effects included nonsevere gastrointestinal, muscle, and bone pain [116]. Given their efficacy in cystic fibrosis and their greater degree of tolerance, oral bisphosphonates remain the first-line therapy for the disease.
Denosumab, teriparatide, and growth hormone are among the promising therapies for osteoporosis in cystic fibrosis, but further tests are needed to determine their potential in the specific context of CF. As an antiresorptive agent in bone disease, denosumab improves bone density in lumbar spine and hip and, unlike bisphosphonates, increases cortical bone density [101]. Although its long-term effects are yet to be assessed, its short-term benefits, coupled with relatively few side effects and twice-yearly dosing, strengthen the likelihood that it will become an effective intervention in CF-related bone disease. The parathyroid hormone, teriparatide, known to increase osteoblast formation and bone growth, may prove useful in CF patients experiencing severe osteoporosis or previous fractures [117]. A study of children and young adults up to age 25 has demonstrated that recombinant growth hormone improves intermediate outcomes in height, weight, and lean tissue mass as well as improvements in exercise tolerance; however, long-term RCMs are needed before it can be administered on a routine basis [118].
The promise of increased survival rates and advanced treatments for CF and its related diseases is enhanced by the existence of more than 120 care centers and 55 affiliated programs, including 96 for adult treatment, at teaching and community hospitals across the country. Initiated in 1960 and accredited by the CF Foundation, this network of multidisciplinary facilities sets and maintains standards for care, research, and education; provides data on health outcomes to the CF Patient Registry; designs CF clinical trials; and identifies the most promising therapies; thereby serving as a model of effective healthcare for other chronic diseases [119, 120].
Renal Osteodystrophy and Disorders of Mineral Metabolism
Abnormalities of bone in the spectrum of chronic kidney disease–mineral and bone disorders (CKD–MBD, also called renal osteodystrophy) can result in a number of serious consequences for long-term bone health including the formation of heterotopic bone, excessive vascular calcification, and abnormalities of bone formation. Irregularities in the metabolism of both calcium and phosphate occur, even in the early stages of renal disease, and moderately impaired glomerular filtration rates (GFR) are evidenced by elevated levels of fibroblast growth factor 23 (FGF23) and hyperparathyroidism. This section will explain the pathophysiology of renal disease and its effect on bones and offer approaches to treatment [121]. Before focusing on the skeletal pathology component of CKD, it is necessary to describe the broader context of metabolic bone changes and related organ pathology involved in CKD.
Pathophysiology of CKD–MBD
In the course of renal disease, a number of circulating inhibitors and promoters work in negative ways to decrease bone formation and bone mineralization (Fig. 4). Fibroblast growth factor FGF23 is increased along with sclerostin, both of which promote bone resorption and bone remodeling, although FGF23 does so only indirectly through mechanisms involving PTH and vitamin D. Produced by osteocytes and osteoblasts, FGF23 exerts a direct action on renal tubules and parathyroid glands. As kidney disease progresses, the number of working nephrons in the kidney continually declines. Hence, more phosphate is excreted by each of the remaining nephrons, a mechanism that is only possible through increased activity of FGF23 and parathyroid hormone (PTH) [122]. The constant elevated production of PTH resulting from hyperplasia of the parathyroid glands results in increased RANKL production. The consequences of increased RANKL in this setting are significant: high turnover renal osteodystrophy, desensitization of the PTH receptor, and excessive bone resorption [121].


Fig. 4
The metabolism of vitamin D and location of actions of vitamin D analogs. Vitamin D analogs doxercalciferol and paricalcitol act similarly to 1α-25OH (calcitriol) but may have less effect on the intestine and bone compared with calcitriol (See page 303)
Additional effects of actions of FGF23 include inhibition of the enzyme 25-hydroxyvitamin D 1-α hydroxylase that produces calcitriol, resulting in decreased intestinal calcium absorption and ultimately hypocalcemia. This cascade of events also reduces the number of active vitamin D receptors on cells in the parathyroid contributing to more definitive adverse changes in CKD–MBD of those with advanced renal disease [123]. High serum phosphorous, low serum calcium, and low calcitriol levels collectively contribute to persisting and worsening hyperparathyroidism. By controlling the levels of calcium and phosphorous early in the course of CKD, practitioners can help to prevent one of the most severe complications of renal osteodystrophy, i.e., hyperparathyroidism [124].
There are three different types of metabolic disorders in CKD: high bone turnover states, low bone turnover states, and mixed disorders. If hyperparathyroidism is present, a high bone turnover state develops which is characterized by irregular collagen production known as woven bone. In comparison to mineralized bone, woven bone lacks strength because of haphazard orientation of the type I collagen fibers that form osteoid. Furthermore, due to enhanced resorption as compared with formation, bone mass is decreased. In contrast, if the prevailing PTH level is insufficient to overcome PTH resistance characteristic of severe CKD, the result is a low bone turnover state, a condition classified as either adynamic bone or osteomalacia. Adynamic bone is characterized by complete lack of osteoid production and new bone formation, whereas osteomalacia results from normal osteoid production but impaired ability to mineralize the osteoid. In CKD, osteomalacia most frequently occurs from aluminum toxicity. In mixed uremic osteodystrophy, both a mineralization defect and high bone turnover state exist [121]. All three disorders are influenced by the extent of vitamin D metabolic irregularities, any immunosuppressive treatment adversely affecting bone maintenance, dietary restrictions, and associated health problems including diabetes. Table 5 compares the various types of bone turnover pathology seen in CKD [121].
Table 5
Pathology and diagnosis of bone turnover in CKD
Predominant hyperparathyroidism | Low bone turnover | Mixed uremic osteodystrophy | Undefined |
---|---|---|---|
Intact PTH >500 pg/ml | Adynamic bone disorder (AMD) 1. Intact PTH <100 pg/ml 2. Normal alkaline phosphatase or bone-specific alkaline phosphatase | PTH >300 pg/ml | PTH >100 pg/ml <500 pg/ml |
Elevated alkaline phosphatase or bone-specific alkaline phosphatase | Osteomalacia 1. Intact PTH <100 pg/ml 2. Normal alkaline phosphatase or bone-specific alkaline phosphatase 3. Low osteocalcin 4. Elevated aluminum | Elevated aluminum |
The intact PTH level varies depending on the severity of CKD. Elevated levels of intact PTH are often desired in later stages of CKD (3–5D) in order to have optimal bone health due to the known skeletal resistance to PTH with uremia. Therapy should be aimed at improving calcium, phosphorous, and vitamin D levels, as well as focusing on PTH levels [124]. If intact PTH falls outside the desired range of 2–9 times, the upper limit of normal in a patient with CKD stage 5D, management plans must be further individualized in conjunction with close coordination with the patient’s nephrologist. Nearly all of the laboratory studies and medical treatments desired at this stage of evaluation can be performed in an inpatient rehabilitation setting. Only in cases of severe CKD (stages 3–5D), which are consistently unresponsive to medical interventions including both dialysis adjustments and pharmacologic agents, would a parathyroidectomy need to be considered.
Diagnostic Evaluation
Serum calcium (or ionized calcium if albumin levels are low), phosphorous, intact PTH, and bone-specific alkaline phosphatase should be measured at baseline when GFR falls below 60 ml/min. Serum vitamin D 25OH should be monitored at least twice annually, especially at the end of winter when levels reach their nadir. Due to diurnal, postprandial, and dialysis (pre and post) variability, it is helpful to pick a consistent time for patients to be analyzed and compare those values over months. In addition, titers used by laboratories differ, particularly for vitamin D and alkaline phosphatase. Although P1NP is an alternative marker of bone formation to bone-specific alkaline phosphatase, it is not widely available, takes longer to process and obtain results (often sent out of a clinic to a specialized lab), and is between three and four times the cost of bone-specific alkaline phosphatase [125]. In reality, the most important measures used for day-to-day management of CKD–MBS remain calcium, phosphorous, vitamin D, and PTH.
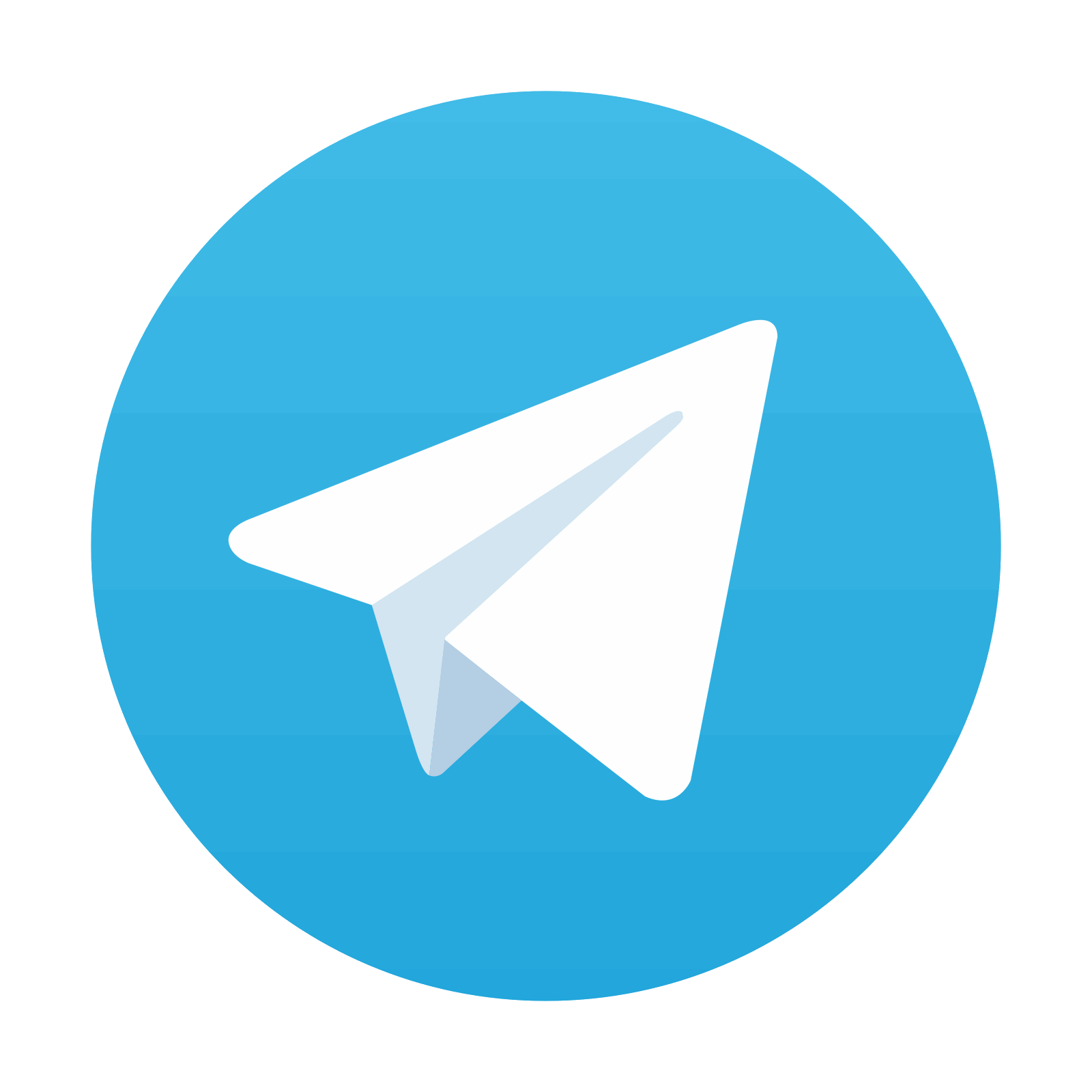
Stay updated, free articles. Join our Telegram channel

Full access? Get Clinical Tree
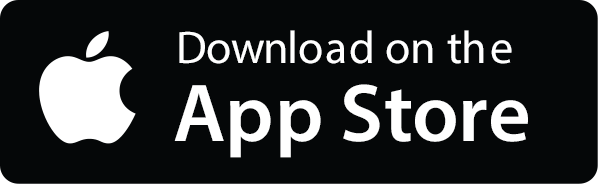
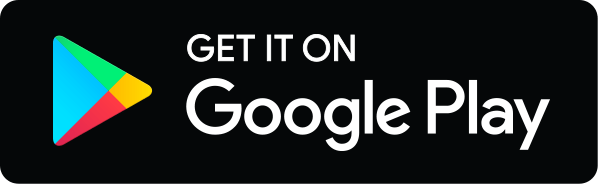