(1)
Department of Orthopedic Surgery Academic Medical Centre, University of Amsterdam, Amsterdam, The Netherlands
Abstract
A patient with OCD typically experiences deep ankle pain on or after activity. This chapter describes the cause of this pain. For diagnosis, additional diagnostics are often needed. The preferred diagnostic strategy is mentioned. For treatment, the most important treatment options are described in detail. It concerns debridement and bone marrow stimulation for primary lesions of <15 mm. Retrograde drilling is for large cystic lesions. Fixation is for large osteochondral defects (> 15 mm in size). Osteochondral transplants, calcaneal osteotomy, and application of a HemiCAP are considered for treatment of secondary osteochondral defects.
A patient with OCD typically experiences deep ankle pain on or after activity.
The pain is caused by repetitive high fluid pressure during walking, which results in stimulation of the highly innervated subchondral bone underneath the cartilage defect.
For preoperative planning, a CT scan is preferred.
The choice of the treatment for osteochondral lesions depends on the duration of complaints, the size of the defect, and if it is a primary or secondary osteochondral defect.
Treatment by means of excision, debridement, and bone marrow stimulation is the first choice of treatments and results in 86 % good/excellent results.
Based on the therapy the following guidelines are proposed:
1.
Asymptomatic lesion or low symptomatic lesion: conservative treatment
2.
Symptomatic lesion up to 15 mm: debridement and drilling/microfracturing/bone marrow stimulation
3.
Symptomatic lesions larger than 15 mm: fixation
4.
Cystic lesion in tibial roof or large talar cystic lesion: retrograde drilling and bone transplantation
5.
Failed primary treatment: osteochondral transplant, chondrocytegraft, HemiCAP, or calcaneal osteotomy
8.1 Introduction
The incidence of (osteo)chondral defects of the talar dome in patients with acute lateral ankle ligament ruptures is 5–7 % (Bosien et al. 1955; Van Dijk 1994; Lippert et al. 1989). These osteochondral defects lead to prolonged swelling and synovitis, diminished range of motion, and pain on weight bearing. A plain radiograph can disclose a lesion but is often negative. Most traumatic cartilage lesions have a good prognosis and patients will become asymptomatic eventually (Fig. 8.1). Traumatic osteochondral lesions have a poor healing capacity. This does not mean that all these lesions eventually will remain symptomatic. Low or non-symptomatic lesions do exist (Fig. 8.1).
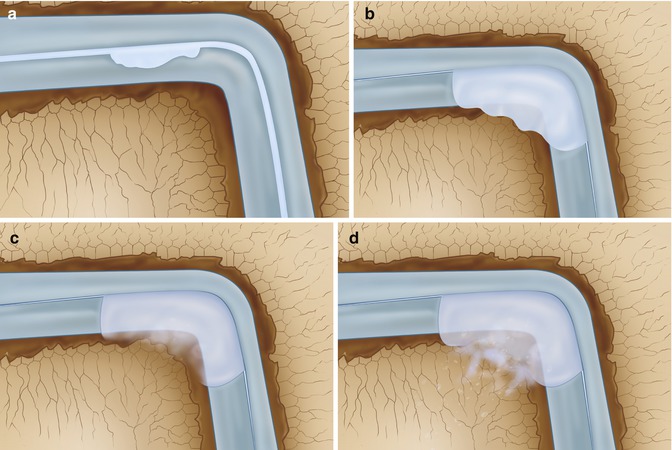
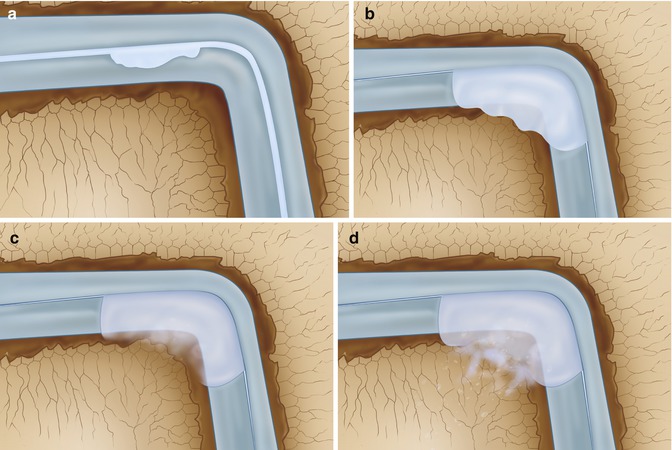
Fig. 8.1
Most traumatic cartilage lesions have a good prognosis, and patients will eventually become asymptomatic. (a) Superficial cartilage damage in which the subchondral bone plate has remained intact. The loose cartilage fragment might cause transient synovitis. Since the subchondral bone is intact, there is no bone pain. The prognosis is good. (b) Osteochondral defect extending into the subarticular spongiosa. The fragment has lost its position and might act as a locking loose body. The donor site defect however will have a spontaneous healing tendency due to filling with a fibrin clot followed by mesenchymal stem cells that will cover the defect with fibrous cartilage (c). On weight bearing the water content of this cartilage will be squeezed into the subarticular spongiosa, but since there is a large/wide defect in the subchondral bone plate, this will not result in a localized high pressure in the subarticular spongiosa. (d) Bone edema will be present for quite some time, but eventually the subchondral bone plate will heal and most patients will become asymptomatic
The nontraumatic etiology concerns idiopathic osteochondral defects. Ischemia, necrosis, and possibly genetics play a role. Osteochondral defects in identical twins and in siblings have been described (Woods and Harris 1995; Anderson and Lyne 1984; Erban and Kolberg 1981). In 10–25 % of patients, the occurrence of the defect is bilateral (Berndt and Harty 1959; Canale and Belding 1980). Most of these lesions are asymptomatic. They can become symptomatic after a traumatic event. In lateral lesions, trauma is described in 98 % of cases; in medial lesions, this is 70 % (Flick and Gould 1985).
Lateral osteochondral lesions are usually located in the anterior third of the talar dome. Medial lesions are mostly located in the posterior half. A lateral lesion has a typical shallow and wafer shape, indicating a shear mechanism of injury. Medial lesions are most often deep and cup shaped, indicating a mechanism of torsional impaction. Lateral lesions are more often displaced than medial lesions.
Repetitive loading of the damaged articular cartilage surface can lead to local cellular degeneration or death by the disruption of collagen fibril ultrastructure and thickening of the subchondral bone (Frenkel and Di Cesare 1999). Animal experiments have shown that oscillating fluid pressure can lead to osteolysis (Van der Vis et al. 1998). Fluid pressure-induced bone resorption is a powerful bone resorptive stimulus. In situations with net bone loss, there is an ongoing bone formation adjacent to bone resorption (Kadoya et al. 1996). This bone resorption due to hydrostatic pressure leads to subchondral cysts surrounded by a newly formed calcified zone (Madry et al. 2010; Pape et al. 2010).
8.2 Cause of the Pain
Several mechanisms have been proposed as a cause of osteochondral defect pain. These include elevated joint pressure, elevated intraosseous (venous) pressure, synovial pain, or bone pain. Synovial pain would imply tenderness on palpation of the inflamed and thickened synovium. The synovium in the ankle joint is accessible for palpation both on the anteromedial and anterolateral joint line. Patients with a symptomatic osteochondral defect usually show surprisingly little abnormality. These patients typically have no synovial swelling, a normal range of motion, and most of all absence of recognizable tenderness on palpation of the synovium. The synovium therefore is not the cause of the pain in these patients (Van Dijk et al. 2010a). In order for joint fluid to cause a relevant rise in articular pressure, the joint must be completely filled. This is not the case in the typical patient with an osteochondral defect. In some of these patients, there is some effusion, but this is not sufficient to give a relevant rise in intra-articular pressure.
The relationship between painful osteoarthritis and a high resting intraosseous venous pressure was studied by Arnoldi, Lemperg, and Linderholm (1971, 1975). They suggested that the high intraosseous venous pressure was the result from blockage flow in the periarticular veins. Osteotomy or cortical fenestration resulted in a remarkable reduction in intraosseous pressure.
It has been determined that nerve fibers are widely distributed in bone tissue such as periosteum and bone marrow (Fig. 8.2). The nerves in the bone marrow show apparent regional distribution with different densities. They are often associated with blood vessels and show a beaded appearance (Irie et al. 2002; Mach et al. 2002).
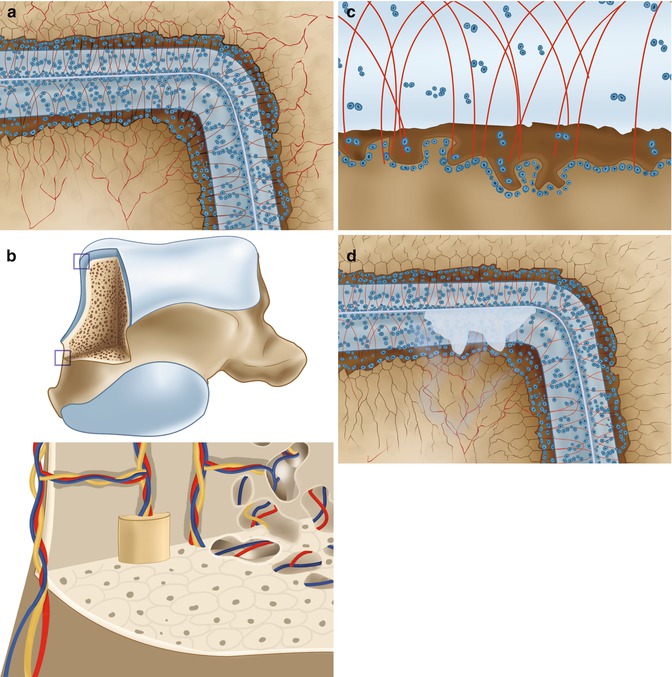
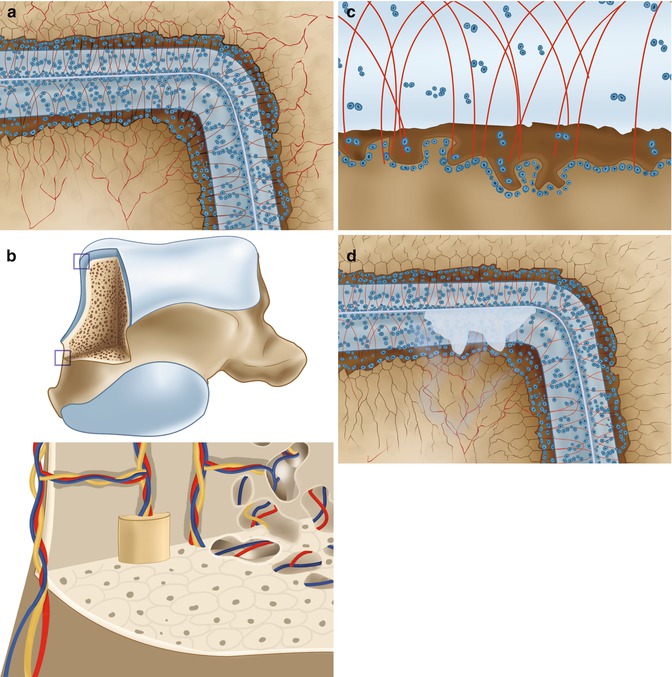
Fig. 8.2
(a) It has been determined that nerve fibers (represented in red) are widely distributed in the subarticular spongiosa. The nerve endings are often accompanied by blood vessels (b). (c) Schematic drawing of cross section of the cartilage showing the composition of cartilage (collagen fibers in orange, chondrocytes in yellow, and the blue color) is water with proteoglycans. Underneath the subchondral bone plate, we find a subarticular spongiosa. (d) Schematic drawing of a cartilage defect extending through the subchondral bone plate. The empty lacunae with dead chondrocytes are shown around the defect. In this situation there is a connection between the joint space and the subarticular spongiosa
Local fluid pressure is a powerful stimulus for the nerve endings in the bone marrow. Measurements of fluid pressure in osteolytic cysts have shown a fluid pressure reaching 200 mmHg (Anthony et al. 1990). Experimentally, a constant fluid pressure of 200 mmHg for 2 weeks has shown to lead to massive bone resorption exactly under the pressurized area (Van der Vis et al. 1998) (Fig. 8.3). Cartilage is a viscoelastic material. The affinity of its matrix for water is the principal factor in determining the stiffness of the cartilage (Madry et al. 2010; Pape et al. 2010). Interstitial water is expressed from the cartilage matrix as it is compressed. The average thickness of the talar cartilage in humans has been determined to be 1.3 mm (1.0–1.6 mm) (Shepherd and Seedhom 1999). Thickness of the articular cartilage is related to the congruency of joint. The ankle joint is the most congruent joint in the lower extremity and has the thinnest cartilage (Fig. 8.4). The thickness of the cartilage from the knee joint was determined by the same authors to be 2.1 mm (1.7–2.6), whereas the average thickness of the hip joint cartilage was determined to be 1.7 (1.3–2.0).
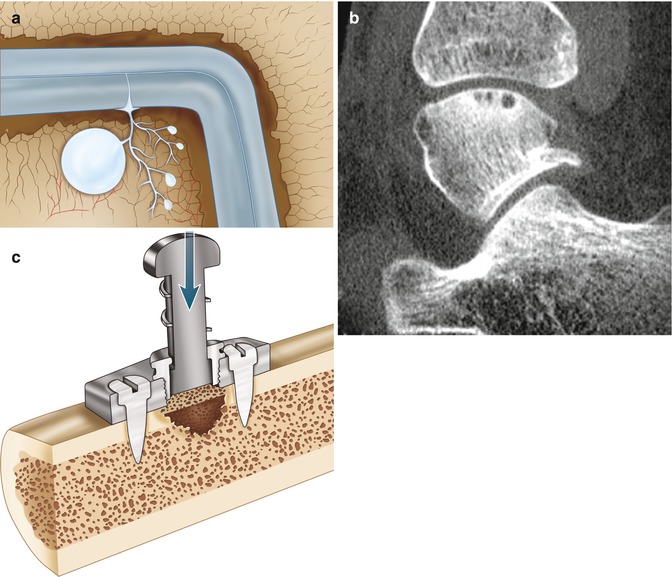
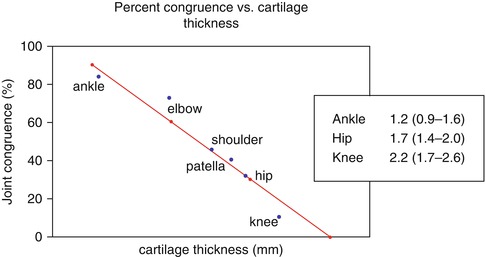
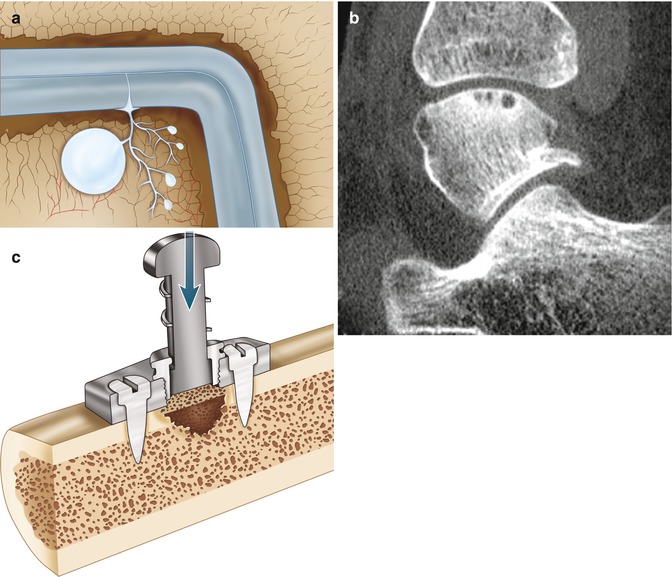
Fig. 8.3
The recurrent oscillating local pressure will induce bone resorption which can lead to cyst formation in the subarticular spongiosa (a). On CT scan multiple cysts can often be seen directly under the subchondral bone plate (b). (c) Research performed by Van der Vis et al. (1998). In their experiment intermittent fluid pressure of 150 mmHg was given to the bone interface of six rabbits (using a fluid chamber). Local osteolysis occurred in all animals (Aspenberg and Van der Vis 1998; Astrand et al. 2003)
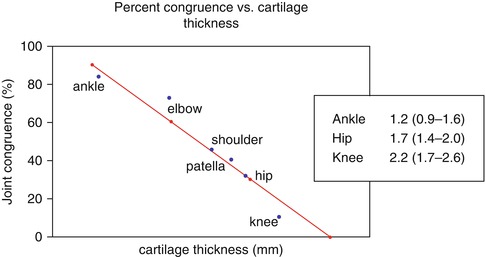
Fig. 8.4
Diagram showing the linear relationship between cartilage thickness and joint congruency. The ankle joint has the thinnest cartilage and the highest congruency, while the knee has the thickest cartilage and the lowest congruency (19). The average thickness of the cartilage of ankle, hip, and knee has been determined in humans (18)
Cartilage consists of cells (chondrocytes), collagen (arcade structure), and water (Fig. 8.2). The water content of human articular cartilage has been determined to be 79 % (Simon 1971). The deformation of the talar articular cartilage under dynamic load (0.1 s) has been determined to reduce 20 % of its thickness. Under static load (30 min), the thickness of the talar cartilage will reduce to 45 % of its original thickness (Simon 1971). There is a difference between a congruent joint and a behavior of the cartilage in a joint with less congruency (Fig. 8.5). When the subchondral bone plate is damaged, water from the cartilage can penetrate in the richly innervated subcortical spongiosa.
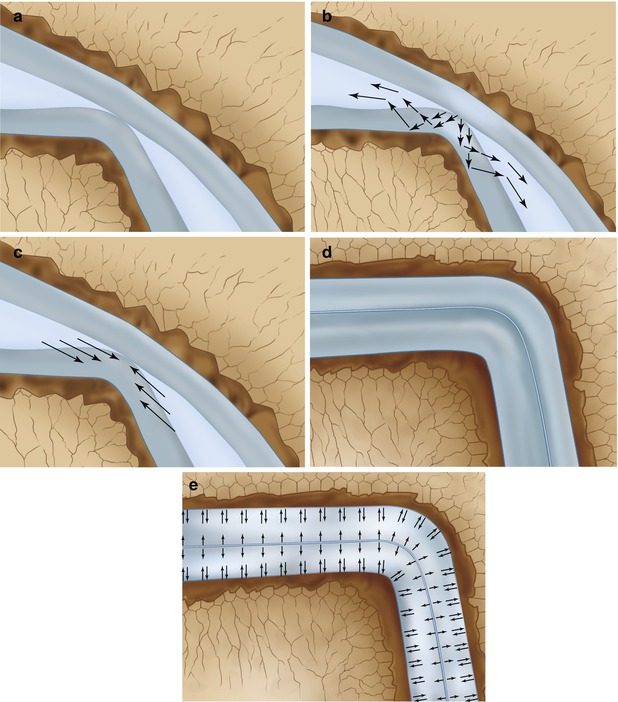
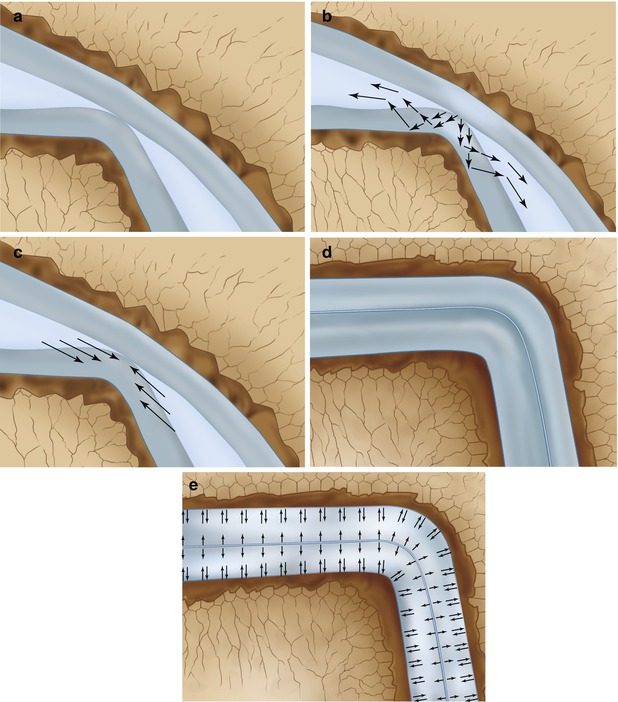
Fig. 8.5
Systematic drawing of deformation of articular cartilage under dynamic load and static load in an incongruent joint (a–c) and a congruent joint (d, e). (a) Systematic drawing of incongruent joint surfaces without loading. (b) Under loading water will flow within the cartilage and some of the water is compressed out of the cartilage into the joint space. (c) When the pressure is released, the water returns to the cartilage restoring its original volume. (d) Schematic drawing of situation in a congruent joint. (e) Under compression the water will remain in the cartilage and cannot move into the joint space. In a congruent joint, under dynamic load deformation will only be 20 %
Edge loading of the damaged talar cartilage by the distal tibia will result in a local fluid pressure which is directed towards the subchondral bone plate (Fig. 8.6). In case of damage of the subchondral bone plate, the fluid can enter the underlying bone (Fig. 8.6) (Gomoll et al. 2010). This recurrent oscillating local pressure will induce bone resorption which can lead to cyst formation (Figs. 8.3, 8.4, 8.5, 8.6, and 8.7).
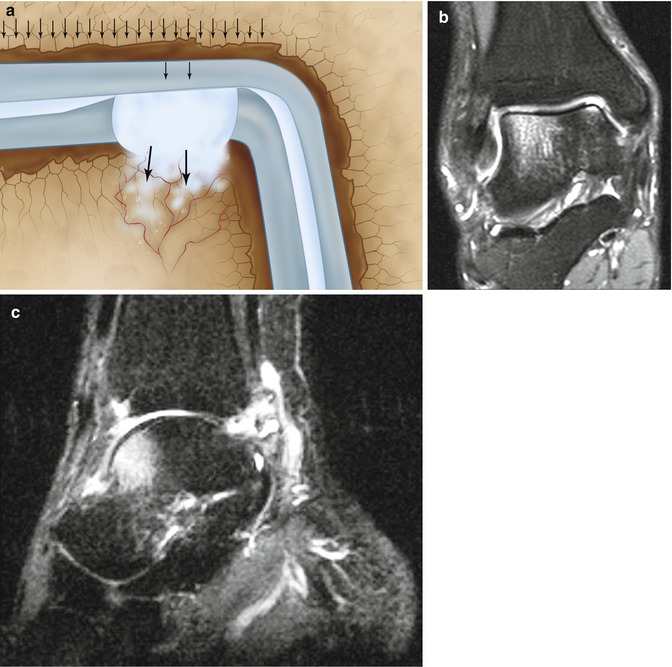
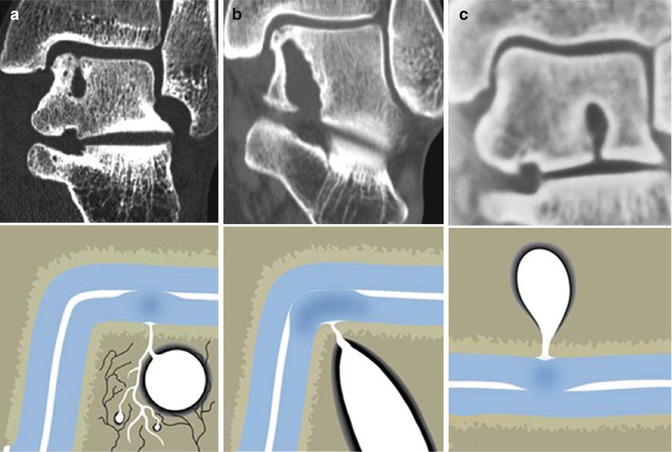
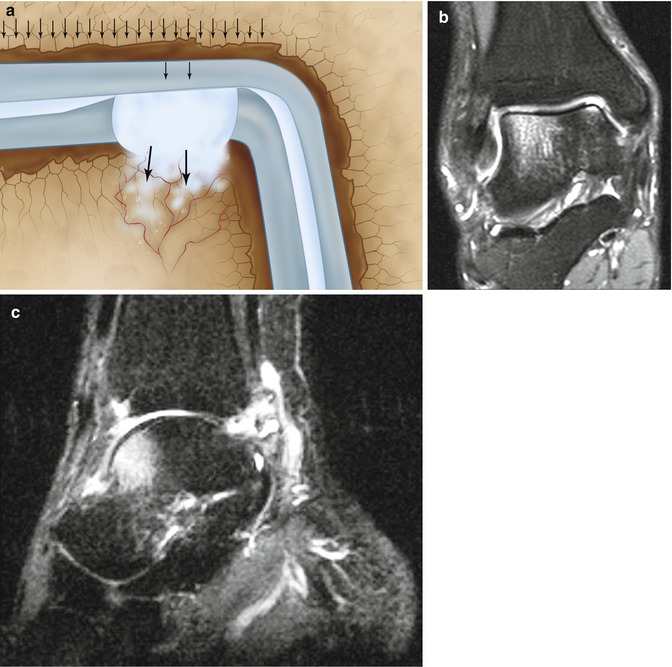
Fig. 8.6
Edge loading of the damaged talar cartilage by the distal tibia will result in a local fluid pressure which is directed towards the subchondral bone plate. In case of damage of the subchondral bone plate, the fluid can enter the underlying bone (a). MRI (b, c) shows the bone edema adjacent to the joint. The bone edema is probably caused by the water which is squeezed out of the cartilage by loading (walking) and enters the subarticular spongiosa through the damaged subchondral bone plate. The smaller the diameter of the defect in the subchondral bone plate, the higher the water pressure!
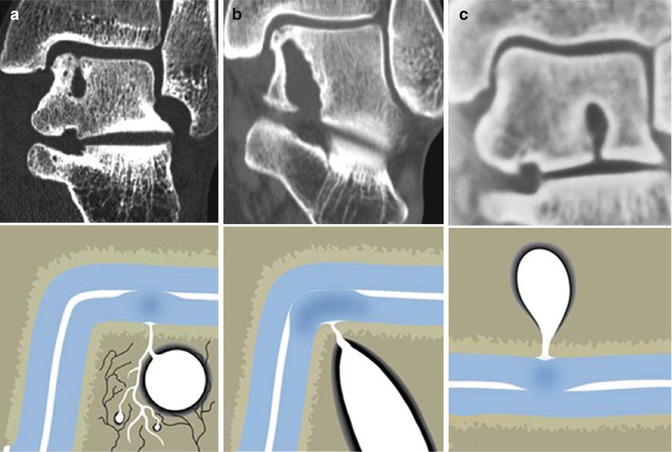
Fig. 8.7
Three examples of local cartilage damage with involvement of the subchondral bone plate which has resulted in a large subchondral cyst. (a) Subchondral cyst on the medial talar dome in a patient with a supination trauma 8 years before. (b) Large cystic lesion extending from the ankle joint to the subtalar joint after compression trauma (car accident) 12 years before. The patient developed deep ankle pain. (c) Patient who jumped from a ship into a life boat 5 m below 15 years before. Patient developed deep ankle pain over the years. CT scan demonstrates a cystic lesion with opening towards the subtalar joint
In conclusion, the pain does not arise from the cartilage lesion but is most probably caused by repetitive high fluid pressure during walking, which results in stimulation of the highly innervated subchondral bone underneath the cartilage defect (Fig. 8.8). Understanding the natural history of osteochondral defects can lead to the development of strategies for preventing progressive joint damage (Van Dijk et al. 2010b).
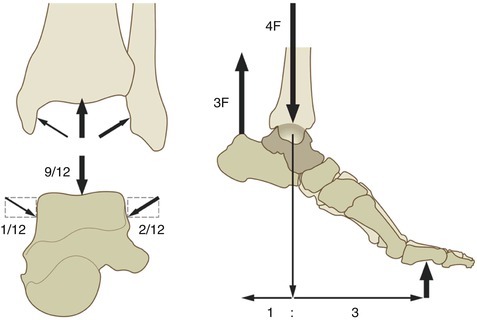
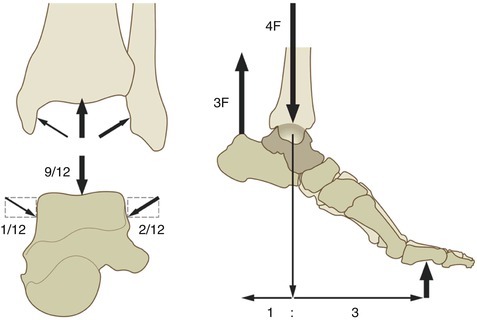
Fig. 8.8
The load on the ankle joint during normal walking can exceed five to six times the body weight
8.3 Clinical Presentation of a Chronic Lesion
These patients typically experience deep ankle pain during or after activity. On examination these patients can show surprisingly little abnormality. These ankles can have a normal range of motion with the absence of swelling and absence of recognizable pain on palpation. Some patients have a diminished range of motion.
8.4 Additional Diagnostics
Routine radiographs of the ankle consist of an AP and lateral X-ray of both ankles. The radiographs may show an area of detached bone, surrounded by radiolucency. Initially the damage may be too small to be visualized on a routine X-ray. By repeating the X-rays in a later stage, the abnormality sometimes becomes apparent.
A heel-rise view with the ankle in a plantar-flexed position may reveal a posteromedial or posterolateral defect (Verhagen 2004) (Fig. 2.12). For further diagnostic evaluation, CT scan and MRI have demonstrated to have similar accuracy (Verhagen et al. 2005). For preoperative planning, a CT scan is preferred however. The multislice helical CT protocol involves actual 0.5-mm acquisition with 0.3-mm reconstruction. Multiplane coronal and sagittal reconstruction should be 2 mm. Most lesions can be treated by means of anterior arthroscopy (Figs. 8.9 and 8.10).
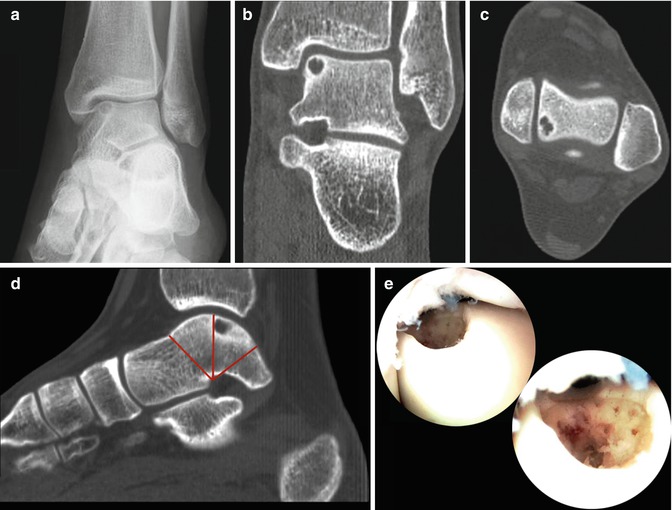
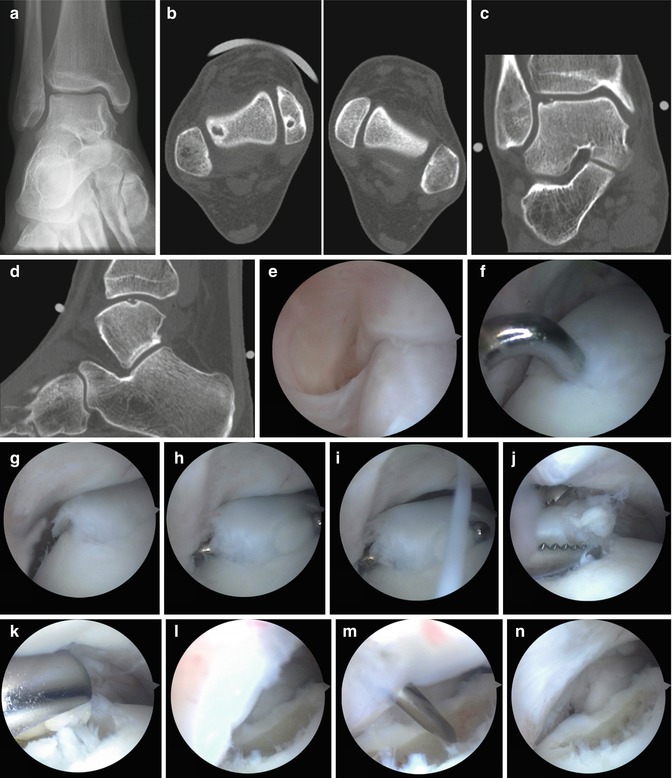
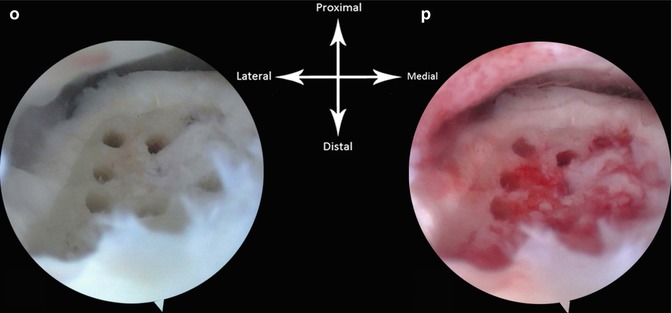
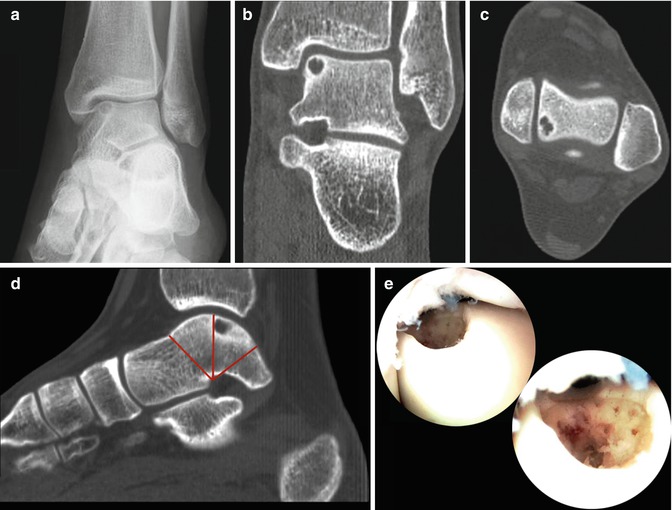
Fig. 8.9
Preoperative planning for a talar OCD is performed on a sagittal CT reconstruction (d). (a) Osteochondral defect on the medial talar dome in the AP standing heel-rise view. (b) CT scan in the frontal plane demonstrating the subchondral cyst in the medial talus. (c) Axial CT view showing the posterior location of the medial defect. The cartilage of the talus is divided in two equal segments (red lines). The defect is located in the anterior part of the posterior segment. This is the location where 90 % of these medial defects are located. If they are situated in this location, they can be brought into the anterior working area (e) by hyperplantarflexion of the ankle. Prerequisite is unrestricted plantar flexion
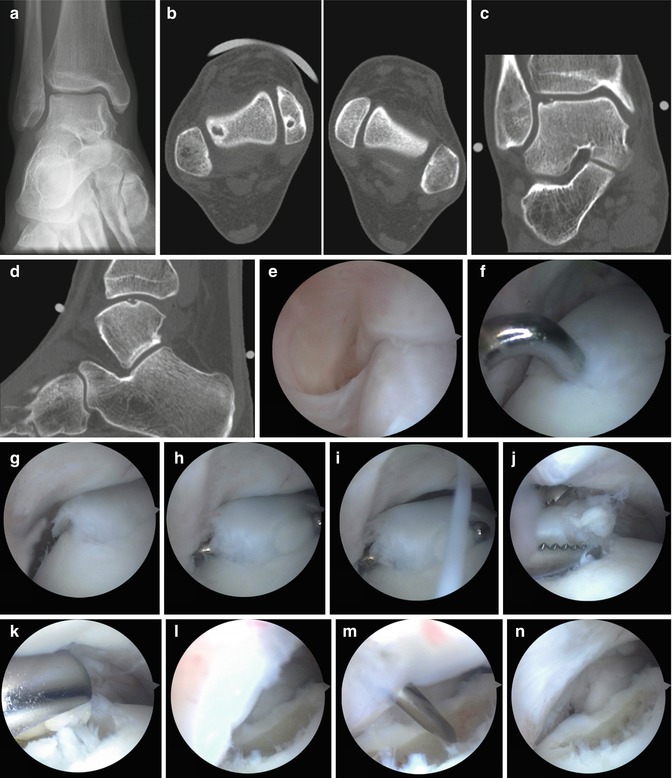
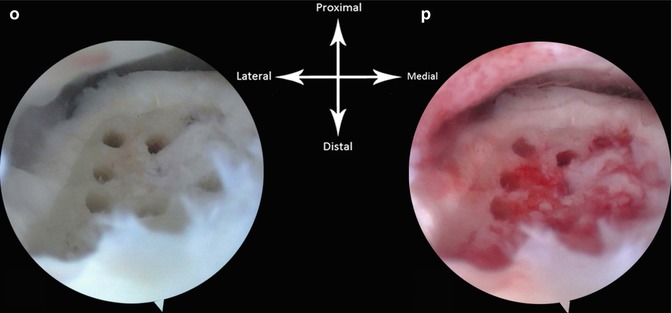
Fig. 8.10
Example of arthroscopic treatment of a lateral talar OCD in a right ankle. (a) AP X-ray demonstrating the defect in the lateral talus. (b, c, and d) Axial view reconstruction of the frontal (c) and sagittal (d) plane demonstrating the lateral defect. (e) Arthroscopic view. Arthroscope is in the anteromedial portal. The ankle is in dorsiflexion. The defect cannot be seen. (f) The ankle is now in plantar flexion and we have applied a soft tissue distractor. The probe is introduced through the anterolateral portal. The anterior part of the defect is palpated as shown. (g) The defect can be seen in the anterolateral corner in plantar flexion without joint distraction. (h, i) The loose cartilage is removed with a probe. (j) The detached cartilage is removed using a grasper. (k) The debridement is further executed with a 5.5-mm aggressive shaver (bonecutter). (l) Situation after debridement with the shaver. (m) Microfracture probe is introduced and multiple openings are made in the subchondral bone. (n) Situation after microfracturing. The scope is still in the anteromedial portal and the defect can just been seen. (o) Direct view on the defect: the scope is now in the anterolateral portal. We therefore now have a better view on the defect. (p) Situation after release of the tourniquet
8.5 Operative Treatment Options
There are various published surgical techniques for treatment of symptomatic osteochondral lesions. Generally, these are based on one of the following three principles:
1.
Debridement and bone marrow stimulation (microfracture, abrasion arthroplasty, drilling)
2.
Securing a lesion to the talar dome (retrograde drilling, fragment fixation)
3.
Stimulating the development of hyaline cartilage (osteochondral autografts, allografts, autologous chondrocyte implantation)
In 2000, we published the result of a literature overview on the treatment of talar OCD (Tol et al. 2000). In 2010 we published an update of this overview (Zengerink et al. 2010). We concluded that the first and best treatment option is debridement and bone marrow stimulation (Table 8.1).
Table 8.1
Literature overview published in 2010 on treatment for talar OCD (Zengerink et al. (2010))
Treatment strategy | No. | Pts. | Success | % |
---|---|---|---|---|
Nonoperative treatment—rest | 3 | 86 | 39 | 45 |
Nonoperative treatment—cast | 3 | 69 | 40 | 58 |
Excision | 4 | 59 | 32 | 54 |
Excision and curettage | 13 | 259 | 201 | 78 |
Excision, curettage, and BMS | 18 | 386 | 325 | 84 |
Excision, curettage, BMS, and autogenous bone graft | 5 | 93 | 64 | 69 |
Antegrade (transmalleolar) drilling | 1 | 22 | 7 | 32 |
Osteochondral transplantation/OATS | 9 | 243 | 214 | 88 |
Autologous chondrocyte implant/ACI | 4 | 59 | 45 | 76 |
Retrograde drilling | 3 | 42 | 37 | 88 |
Fixation | 1 | 27 | 24 | 89 |
Total | 64 | 1,345 | 1,028 | 76 |
The choice of treatment for osteochondral lesions depends on the duration of complaints, the size of the defect, and if we deal with a primary or secondary osteochondral defect. None of the current grading systems are sufficient to direct the choice of treatment (Fig. 8.11).
Asymptomatic or low-symptomatic lesions are treated conservatively with rest, ice, temporarily reduced weight bearing, and in case of giving way, an orthosis.
For mechanically unstable lesions, treatment options are fixation, debridement with bone marrow stimulation, retrograde drilling, osteochondral transfer, or autologous chondrocyte transplantation.
Fixation is preferred in (semi)acute situations in which the fragment is 15 mm or larger. In adolescents refixation of an osteochondral defect must always be considered even in fragments that are smaller than 15 mm. Large talar cystic lesions can be treated by retrograde drilling and filling the defect with a bone graft. In case of failed primary treatment, an osteochondral transplant can be considered. The place of cultured chondrocyte transplant (MACI) and (massive) allografts still needs to be determined. Treatment by means of excision, debridement, and bone marrow stimulation is the first choice of treatment and results in 86 % good/excellent results. Concerning osteochondral transplants, an ISAKOS expert panel stated that the technique looks simple, but this is not the case (Van Dijk 2005).
The technique of osteochondral transplant should be reserved for secondary cases. Often a malleolar osteotomy is needed in order to adequately gain access to the lesion (see Sect. 8.11).
The type of surgical treatment will influence the exposure. The majority of primary lesions can be treated by arthroscopy. Many posteromedial lesions do not have to be treated by malleolar osteotomy, but can be treated arthroscopically by bringing the foot in hyperplantarflexion, although skill and experience are required (Schuman et al. 2002).
Canale has recommended medial malleolar osteotomy when dealing with posterior medial lesions. In an effort to avoid the osteotomy, Flick and Gould have suggested the use of an anteromedial approach combined with “grooving” of the anteromedial distal tibia. Thompson and Loomer have recommended a combined anteromedial and posteromedial approach (Flick and Gould 1985; Thompson and Loomer 1984). Recently Jakob has described a technique to treat lateral defects by the osteotomy of the lateral malleolus (Jakob et al. 1997). Another option is to use an aiming device and drill through the medial malleolus (Fig. 6.7). In our experience, 90–95 % of all lesions can be treated by means of anterior arthroscopy. In 5 % we approach the lesions with a two-portal hindfoot approach.
8.6 Classification and Staging
A number of classifications for osteochondral defects have been proposed. The first classification was from Berndt and Harty.
Classification of Berndt and Harty (1959).(Berndt and Harty 1959):
Stage I: A small compression fracture
Stage II: Incomplete avulsion of a fragment
Stage III: Complete avulsion of a fragment without displacement
Stage IV: Displaced fragment
Ferkel and Sgaglione described the CT-based classification. Andersson described an MRI-based classification and Cheng and Ferkel described an arthroscopy-based classification (Ferkel and Sgaglione 1993; Andersson et al. 1989; Cheng and Ferkel 1998).
Since the first step in treatment of symptomatic lesions is debridement and bone marrow stimulation, the ISAKOS consensus group feels only a limited need for these classification systems. Based on the therapy, the following guidelines are proposed:
1.
Asymptomatic lesion or low-symptomatic lesion: conservative treatment
2.
Symptomatic lesion up to 15 mm: debridement and drilling/microfracturing/bone marrow stimulation
3.
Symptomatic lesions larger than 15 mm: fixation
4.
Cystic lesion in tibial roof or large talar cystic lesion: retrograde drilling and bone transplantation
5.
Failed primary treatment: osteochondral transplant, HemiCAP, or calcaneal osteotomy.
For 4 and 5, debridement and bone marrow stimulation must also be considered (Reilingh et al. 2010, 2012; Van Bergen et al. 2011a, 2013; Knupp et al. 2011).
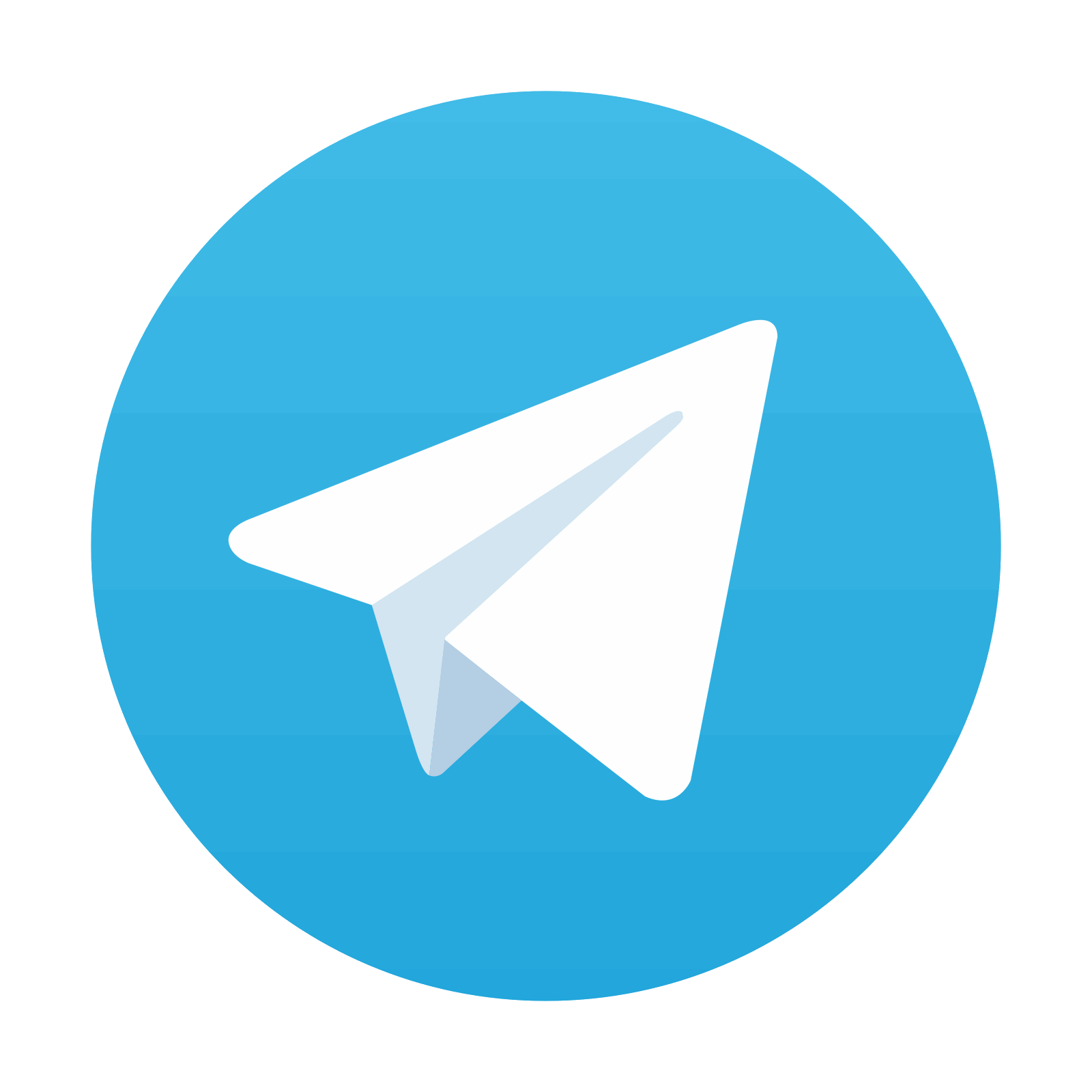
Stay updated, free articles. Join our Telegram channel

Full access? Get Clinical Tree
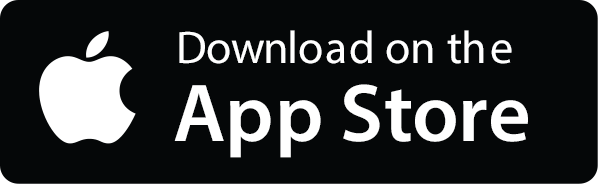
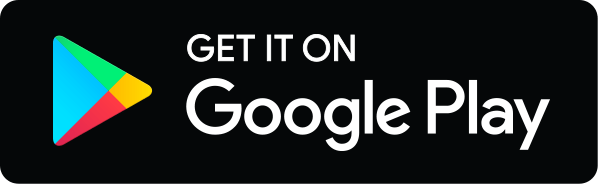