16
Osteochondral Allograft Transplantation
Experience with fresh osteochondral allografting in the management of chondral and osteochondral injuries extends over many decades. In fact, the first documented use of fresh allografts was by Erich Lexer1 in 1908. In more modern times, the pioneering work by Dr. Alan Gross and Marvin Meyers in the 1970s has led to greater understanding of the indications, outcomes, and basic science of fresh osteochondral allograft transplantation in the treatment of chondral and osteochondral injuries.2,3 Traditionally, the complexities of allograft surgery, including the recovery, procurement, testing, and storage of fresh tissue, as well as the surgical technique, have limited the use of allografts to specialized centers that have devoted time and effort to overcoming many of the obstacles and difficulties in working with fresh allografts. More recently, however, allografts have become increasingly popular in the orthopaedic community, and as a result the number of allograft procedures is increasing, limited mainly by the availability of fresh allograft tissue. Historically, allografts have been used in the treatment of large chondral or osteochondral defects, particularly in the knee,2–9 but also in the ankle10,11 and hip.12 In the algorithm of cartilage restoration, allografts often are indicated as a salvage treatment in posttraumatic situations after periarticular fracture or in lesions where there is a significant osseous component, such as osteochondritis dissecans (OCD)or avascular necrosis. Fresh allografts may also be employed after failure of other biologic resurfacing techniques such as microfracture, osteochondral autograft transplantation system (OATS), or autologous chondrocyte implantation. Very occasionally, allografting is considered as an alternative to arthroplasty in the young, active individual. In the athlete, allografts are most useful in those individuals with major trauma, or with large focal or multiple defects, who have failed to respond to more conservative measures.
Current techniques of cartilage repair and restoration generally involve two fundamental strategies. The first is to induce cells to form tissue that can function as an articular surface. Examples of this include abrasion, microfracture, periosteal grafts, autologous chondrocyte implantation, and many tissue-engineering techniques that are not yet clinically available. The second strategy of biologic resurfacing involves the replacement of diseased or absent tissue with architecturally mature articular cartilage. Surgical techniques that fall into this realm include osteochondral autografting, osteochondral allografting, and perhaps in the future, tissue-engineered articular cartilage constructs.
The rationale for fresh allografting thus relies on the consideration that it is a tissue or organ transplantation procedure, utilizing mature hyaline cartilage with underlying bone that functions as a vehicle for attachment, and in some cases to fill an associated osseous defect. The fresh osteochondral graft, therefore, is a composite of living chondrocytes supporting the hyaline matrix and nonliving bone. The chondrocytes survive transplantation and support the hyaline matrix over time, whereas the allograft bone is replaced by host bone, over time, by the process of creeping substitution.13,14
Cartilage is an ideal tissue for transplantation. It is avascular, aneural, and by virtue of chondrocytes being embedded within the hyaline matrix, relatively immuno-privileged.15 Further, cartilage is amenable to storage for a period of time,16,17 and the cartilage can be fashioned to fit the recipient’s anatomy.
Experience with fresh allografts at our institution began in 1983 with an institutional review board—approved protocol. The success of this program was directly related to a close association with a tissue bank and the development of clinical protocols and research programs. Nearly 500 procedures have been performed during this period, and currently about 50 per year are performed, depending on tissue availability. The vast majority of these procedures involve the knee; however, the ankle is another joint that has been successfully treated with allografting.10,11 Allografting of the hip has been performed, but on a limited basis.12 Finally, it should be noted that there is no theoretical contraindication to performing allografts on any joint; however, the indications and thus the clinical experience is relatively limited in these situations.
Indications
Clinical experience with fresh osteochondral allografting has demonstrated its utility in a wide spectrum of pathology, including osteochondritis dissecans, large or traumatic or degenerative chondral lesions, osteonecrosis, posttraumatic reconstruction after intraarticular fracture, and increasingly, in the salvage of failed cartilage restorative procedures, such as microfracture, osteochondral autografting, or autologous chondrocyte implantation. Additionally, allografts can be utilized in carefully selected cases of patellofemoral disease, such as chondrosis or secondary arthrosis, and in unicompartmental arthrosis of the knee joint. In the ankle, allografts are indicated for the treatment of large OCD lesions and osteonecrosis of the talus, and in the treatment of traumatic arthritis, as an alternative to arthrodesis or arthroplasty.
In our experience, fresh allografts can be utilized in treating defects of any size. In most cartilage restoration algorithms, however, fresh allografts are reserved for lesions larger than 2 cm2 that have associated osseous defect or abnormality. In practical terms, the most common indication in the athlete is in the treatment of OCD, or as a salvage after failure of another cartilage repair technique (Table 16–1).
Patient Selection
Patient selection is critical to the success of the fresh allografting procedure. Careful evaluation of the patient’s goals and activity level are vital. Patients should have realistic expectations about the outcome and their ability to return to high-level activity. Patient age is important, because allografting, just like other biologic resurfacing techniques, is less successful in patients over 40 than in those in their 20s. This relates to the important issue of the biologic status of the joint, and the concept of the continuum of cartilage disease. One can easily recognize that a focal lesion in a 21-year-old with an otherwise healthy knee is a far different situation from a similar-sized lesion in a 45-year-old with secondary degenerative changes and multiple previous surgeries. As such, it is crucial in the preoperative assessment to perform a careful evaluation of the biologic and mechanical status of the involved limb. This includes attention to the status of ligaments, meniscus, and other compartments of the knee joint, as well as a careful assessment of the mechanical alignment. Instability and mechanical malalignment should always be corrected prior to, or simultaneously with, the allografting procedure. Finally, the lesion size, depth, and location should be evaluated, usually arthroscopically. Most often, patients have had previous surgical intervention, and the status of the knee is fairly well defined.
Knee |
---|
1. Chondral lesions |
Traumatic |
Degenerative |
2. Osteochondritis dissecans |
3. Salvage of previous cartilage procedure |
4. Posttraumatic reconstruction |
Tibial plateau fracture |
Femoral condyle fracture |
5. Osteonecrosis |
6. Patellofemoral chondrosis or arthrosis |
7. Unicompartmental arthrosis (selected cases) |
Ankle |
1. Osteochondritis dissecans |
2. Osteonecrosis |
3. Posttraumatic arthrosis |
Hip |
1. Osteonecrosis |
2. Osteochondral fracture |
Imaging studies include the following radiographs: standing anteroposterior (AP), lateral, 45-degree posterior-anterior views, and standing alignment films, as well as patellofemoral views, when indicated. These provide a comprehensive evaluation of the osseous structures, and can characterize the pattern of joint disease. Additionally, advanced imaging studies such as magnetic resonance imaging (MRI) are useful, particularly where there are questions about ligament and meniscus status, or when the disease involves subchondral bone, such as is seen in OCD or osteonecrosis. Finally, and perhaps more importantly with allografting than with any other cartilage-restorative procedure, a careful discussion and informed consent process should be undertaken by the surgeon. This is due to the fact that allograft donor material will be used, and both the surgeon and the patient should understand the unique risks inherent in this procedure, including, but not limited to, viral and bacterial disease transmission.
Surgical Technique
Femoral Condyle
Preoperative planning includes careful informed consent, and matching of donor and recipient. Currently, no attempt is made at matching tissue type or blood group. Thus, size is the important parameter in matching donor with recipient. Preoperatively, a standing AP knee x-ray is obtained with a radiographic marker. A corrected size measurement, typically of the tibial plateau width or the femoral condyle width, is made, and this measurement is provided to the tissue bank to obtain a matching donor.18 Measurements obtained with computed tomography (CT) scans or MRIs may be acceptable, but we have no experience with their accuracy in matching osteochondral grafts, although this technique is used for meniscal allografts.
Once a match is obtained and the tissue becomes available, the surgical technique depends on the site to be grafted. Most commonly, grafting involves the femoral condyle. Arthroscopic evaluation is performed only when indicated, if there is a question about other pathology, or if meniscal or ligament treatment is necessary.
The knee is prepped and draped in standard fashion. Care is taken to place a bolster on the table, to allow the knee to stand free in about 70 to 100 degrees of flexion, to access the femoral condyle easily. A midline incision measuring 6 to 7 cm is made, and a miniarthrotomy is performed, either medially or laterally, depending on the site of the lesion. This incision is extended carefully to the mid- or superior patellar retinaculum, and distally into the fat pad, taking care not to injure the meniscus. In some cases where the lesions are very posterior, typically in the lateral femoral condyle, the meniscus must be taken down to gain access. This can be done by incising the meniscus within a few millimeters of the anterior attachment, leaving enough tissue to perform suture repair at the conclusion of surgery.
Retractors are placed to expose the femoral condyle (Fig. 16–1). Careful placement of a retractor in the notch allows mobilization of the patella, but it should be noted that high degrees of flexion decrease the ability to mobilize the patella. This is particularly true in the setting of OCD of the medial femoral condyle, where the lesion extends into the notch, making mobilization of the patella crucial to accessing the lesion. Fortunately, in this setting, the lesions are very anterior, and lower degrees of knee flexion allow easy access with mobility of the patella. Trochlear lesions can be accessed by extending the knee and retracting the patella. Rarely is it necessary to perform a full arthrotomy with patellar eversion unless grafting is to be done on both condyles.
FIGURE 16–1 Miniarthrotomy with exposure of femoral condyle lesion.
Once the site of the lesion is inspected, a size measurement is obtained. This can be done either with a ruler or by utilizing commercially available instruments (Fig. 16–2) (Arthrex, Naples, FL). The availability of instruments to allow preparation of dowel-type allografts from 15 to 35 mm has improved the precision and facilitated the performance of femoral condyle allografts; however, the surgeon should be ready to prepare the allograft freehand, utilizing curettes, osteotomes, and high-speed burrs, in situations where dowel grafts are not appropriate.
FIGURE 16–2 Typical instruments used for preparation of dowel-type allografts up to 35 mm in diameter.
Once the site is exposed, a guide pin is placed through the center of the lesion, and the size measurement is obtained. The cartilage is then scored, utilizing a special cannulated cartilage cutter, and a router is used to prepare a circular defect. Great care must be taken not to extend this too deep into the subchondral bone. Typically, the depth within the subchondral bone is 3 to 5 mm, making the entire composite depth 5 to 8 mm, including the healthy surrounding cartilage (Fig. 16–3). Occasionally, the preparation needs to be performed deeper, to remove diseased bone. Rarely does this require a depth of greater than 10 to 12 mm.
The next step is to measure the depth of the prepared recipient site, utilizing a depth gauge. These measurements are performed in four quadrants, and transferred to a map, which will be used in preparing the donor material.
On the back table, the fresh graft is removed from its packaging and inspected. (Ideally, this should be done prior to surgery, to ensure the appropriate size and quality of the donor material, prior to incision.) The graft is then placed into a graft holder, and the corresponding position of the lesion on the recipient is marked. Landmarks such as the notch, sulcus terminalis, or other sites can be used to confirm the appropriate position. This becomes critical as graft size increases, to create a graft that has a similar contour to the recipient knee. Once this is performed, a mark is made on a defined position on the graft, to determine rotation after the graft is removed. A cutting guide is placed over the site of the graft, taking care to align this perpendicular to the graft surface and at the same angle that was used to prepare the recipient socket, and a tube saw is utilized to core out the appropriate sized graft. The core is then removed and inspected. The depth measurements made in the recipient are then transferred to the graft core and marked. The allograft is placed in a holder, and an oscillating saw is used to resect portions of the osseous component of the graft until the appropriate thickness in all four quadrants is obtained (Fig. 16–4). A rasp can be used for final contouring. At this point, it is critical to wash the graft thoroughly, utilizing high-pressure lavage, to remove as much marrow element as possible. A final check of measurements should be done to confirm the appropriate thickness of the graft in relationship to the recipient socket. Next, the graft is placed into the site and gently tamped in place (Fig. 16–5). In situations where there is difficulty fitting the graft, dilators are available to dilate the graft; also, a rasp may be used to gently round the corners of the graft, to aid in its fitting.
FIGURE 16–3 Preparation of the recipient defect.
FIGURE 16–4 The allograft is harvested and trimmed to appropriate thickness.
FIGURE 16–5 Final implantation of the allograft prior to placement of polydiaxanone (PDS) pins.
Once the graft is seated, a decision is made regarding whether adjunctive fixation is necessary. We routinely use absorbable pins (Orthosorb, Johnson & Johnson, Raynham, MA), because of concern about micromotion of the graft in the postoperative period. We have seen no deleterious effect from placing 1.3-mm pins through the graft surface; in fact, MRI studies have shown the dissolution of these pins and healing within 3 to 6 months.19 After the graft is seated, the range of motion should be carefully checked, to ensure that the graft is not catching or unstable. Once this is performed, the knee is irrigated, to remove any debris, followed by routine closure.
Patellofemoral Joint Surgical Technique
The allografting procedure can be performed in the femoral trochlea with a technique quite similar to that for the femoral condyle. In this setting, a slightly larger arthrotomy is necessary, and most lesions are amenable to dowel-type grafting. Great care should be taken in this region, because the complex anatomy can lead to technical errors in preparation and creation of the grafts. On the patellar surface, hemipatellar grafts can be utilized for small lesions. Often we find it necessary to resurface the entire patella, and this is performed in a fashion similar to placing a prosthetic patellar component (Fig. 16–6). The entire patellar articular surface is removed, leaving a residual osseous component of 12 to 16 mm. The patellar graft is resected freehand, taking care to include at least 2 mm of subchondral bone with the lateral facet, and placed in the appropriate position on the resected surface, creating a type of sandwich. Tracking then is checked, and fixation of the graft is performed with small-fragment screws from the anterior surface of the patella, in a lag fashion.
FIGURE 16–6 Patellar allograft.
Tibial Allografts
The majority of tibial allografts involve resurfacing the entire medial or lateral tibial plateau. These are performed in cases of posttraumatic injuries, such as those seen in tibial plateau fractures. Occasionally, focal lesions of the tibia are resurfaced, which present a challenge in accessing the lesions. Anterior detachment of the meniscus is necessary in these cases, and in our experience it has been unusual to require take-down of the collateral ligaments. Flexion and rotation often allows access to the anterior half of the tibial plateau surface where the dowel instrumentation can be utilized. Central lesions can be grafted utilizing a freehand technique, which is technically very challenging. When 50% or more of the tibial surface is involved (the most common scenario), resurfacing the entire plateau is recommended. In cases where the entire tibial plateau is resurfaced, the use of fluoroscopy is necessary. Reciprocating and oscillating saws are used to resect the tibial surface. This can be done either freehand or utilizing Kirschner wires (K-wires) to guide appropriate slope and alignment. Alternatively, a unicompartmental arthroplasty jig can be employed. A minimal resection (2–6 mm) is desired, understanding that typically there is already loss of plateau height from previous trauma. If the recipient meniscus is to be preserved, it is detached anteriorly and protected, and the vertical resection on the plateau is carefully made on the up-slope of the tibial spine, protecting both the posterior attachment of the meniscus and the anterior cruciate ligament. Once the tibial surface is resected, the leg is brought into extension and the measurement of the extension space is performed with a caliper. This gap is typically 10 to 15 mm. Measurements of the anteroposterior and mediolateral dimensions of the prepared defects are also made, and the tibial allograft is carefully marked. Reciprocating and oscillating saws are used to carefully remove the allograft hemiplateau from the tibial graft. Remeasuring and recutting is routine; and trial fittings are performed as the surgeon checks visually and fluoroscopically for restoration of plateau height, slope, graft position, coverage, and stability. When satisfactory fit and stability are obtained, the graft is lavaged. Fixation is obtained with small interfragmentary screws placed in the midcoronal region and anteriorly (Fig. 16–7). The meniscus is repaired with nonabsorbable suture. In situations where meniscus allografting is performed in conjunction with the osteochondral allograft, it is important to complete the recipient meniscectomy. Plateau resection is performed closer to the cruciate insertion, and the plateau graft is prepared to include the insertions of the meniscus. All residual soft tissue is removed from the composite allograft, and meniscal suturing is performed after fixation of the tibial allograft.
FIGURE 16–7 Tibial allograft.
Postoperative Care
For femoral condyle grafts, the postoperative management is straightforward. Patients typically are allowed full range of motion, except in cases where meniscus has been repaired. A continuous passive motion (CPM) machine is used during the brief hospitalization; physical therapy, including quadriceps-setting exercises and crutch training, is performed. Patients undergo a period of 6 to 12 weeks of protected weight bearing, and begin closed-chain exercises at 4 weeks. Unlimited weight bearing is generally allowed at between 2 and 4 months, depending on the size of the graft and the rehabilitation of the knee. Radiographs are obtained at 4 to 6 weeks, 3 months, and 6 months to assess healing of the allograft. Full activity is allowed at 6 months, if functional rehabilitation is complete.
Postoperative management of patellofemoral allografts includes restricted knee flexion (typically 0–45 degrees for the first 4–6 weeks) utilizing a range-of-motion brace. Unrestricted weight bearing is generally allowed in full extension.
Postoperative management of tibial allografts is similar to that for femoral allografts, with attention to meniscal protection and often a longer period (3–4 months) of restricted weight bearing. In addition, range-of-motion braces routinely are utilized, followed by prescription of an unloader brace to be used for at least 1 year. Patients are generally cautioned about excessive impact loading of the allograft, particularly in the first year.
No immunosuppression is utilized with fresh osteochondral allografts, and the use of antiinflammatories is carefully considered, due to concerns with inhibition of osseous healing, which is critical to the success of the allograft.
Complications
Early complications unique to the allografting procedure are few. There does not appear to be any increased risk of surgical site infection with the use of allografts as compared with other procedures. The use of a miniarthrotomy in the knee likely decreases the risk of postoperative stiffness. Occasionally, one sees a persistent effusion, which is typically a sign of overuse, but which may also indicate a possible graft-mediated synovitis.
Delayed union or nonunion of the fresh allograft is the most common early finding. This is evidenced by persistent discomfort or visible graft—host interface on serial radiographic evaluation. Delayed union or nonunion is more common in larger grafts, such as those used in the tibial plateau, or in the setting of compromised bone, such as in the treatment of osteonecrosis. In this setting, patience is essential, and complete healing or recovery may take an extended period. Decreasing activities, instituting weight-bearing precautions or the use of braces, and possibly using external bone stimulators may be helpful in the early management of a delayed healing. In such cases, careful evaluation of serial radiographs can provide insight into the healing process; MRI scans are rarely helpful, particularly prior to 6 months postoperatively, as they typically show extensive signal abnormality that is difficult to interpret. It should be noted that with adequate attention to postoperative weight-bearing restrictions and adequate graft fixation, delayed union or nonunion requiring repeat surgical intervention within the first year is extremely uncommon.
The natural history of the graft that fails to osseointegrate is unpredictable. Clinical symptoms may be minimal, or there may be progressive clinical deterioration and radiographic evidence of fragmentation, fracture, or collapse. Typical symptoms of this type of graft failure include increase of pain or sudden onset, often associated with minor trauma. Effusion, crepitus, or focal, localized pain is commonly seen. Careful evaluation of serial radiographs typically will demonstrate collapse, subsidence, fracture, or fragmentation. CT or MRI also can be utilized to confirm graft failure.
Late complications associated with the allografting procedure also usually involve the osseous portion of the graft. The requisite event for a successful fresh allograft procedure is healing of the host—graft bony interface and integration of the host bone into the osseous portion of the allograft. This process of so-called creeping substitution is well described in the paradigm of bone—graft healing. Revascularization of the allograft bone by the host may take many years, and may not be complete.20 The amount of bone within the allograft may be important in this process, and it is likely that thinner grafts will have more complete revascularization than thicker grafts. Retrieval studies13,14,20 of failed fresh osteochondral allografts have provided tremendous insight into the allograft healing process, and have led to the understanding that fresh osteochondral allografts rarely fail due to the cartilage portion of the graft; rather, most failures originate within the osseous portion of the graft, or from progression of the host joint disease process, that is, osteoarthrosis. It is likely that late allograft failure, which has been seen at between 2 and 17 years, is the result of graft subsidence collapse or fragmentation due to fatigue failure, very much like that seen with bulk allografts placed under repetitive loading situations. This clinical finding underscores the need to pay close attention to joint alignment and stability in the initial treatment of the patient. Clinically, the patient presents with new pain or mechanical symptoms, of either insidious or acute onset. Radiographs show cysts or sclerosis, or perhaps subchondral collapse, typically in the center of the graft, which may be most distant from the revascularization process, or in an area that has been under higher load due to activity of the patient or malalignment. Again, careful review of serial radiographs is important. MRI also may be useful at this point, and generally is obtained to confirm suspected allograft pathology and to rule out other sources of pain or sites of pathology in the knee joint (Fig. 16–8). It is important to note that the allografted joint may suffer from the same pathology that is present in any other joint, such as meniscus or ligamentous injury. It should also be noted that radiographic and magnetic resonance abnormalities are commonly noted, even in well-functioning allografts,19 and great care must be taken in interpreting and correlating the imaging studies with clinical findings. Finally, it is not yet clear whether allografting alters the natural history of an injured joint. Therefore, over time, progression of cartilage disease and frank osteoarthrosis is possible and likely, particularly in the older population. Further intervention for a previously allografted knee may not be related to the allograft.
FIGURE 16–8 Failed allograft. Note collapse of subchondral bone and irregularity of articular surface.
Treatment options for failed allografts include observation, if the patient is minimally symptomatic and the joint is thought to be at low risk for further progression of disease. Arthroscopic evaluation and debridement also may be utilized. In many cases, revision allografting is performed, and generally has led to a success rate equivalent to primary allografting. This appears to be one of the particular advantages to fresh osteochondral allografting, in that fresh allografting does not preclude a revision allograft as a salvage procedure for failure of the initial allograft. In cases of more extensive joint disease, particularly in older individuals, conversion to prosthetic arthroplasty is necessary.
Case Example
A 17-year-old high school baseball player sustained a noncontact injury to his left knee while running the bases. He presented with mild medial knee pain and mechanical symptoms. Clinical evaluation, including plain radiographs and MRI, revealed a large osteochondritis lesion of the medial femoral condyle, with fragment displacement and loose bodies (Fig. 16–9A). He was unable to fully extend the affected knee. Ligament and meniscus exam were unremarkable, and there was no axial malalignment of the limb. His past history was remarkable for occasional knee discomfort for many years that did not interfere with activity and was diagnosed as “growing pains.”
The patient underwent arthroscopic debridement and removal of loose bodies. The lesion measured 2.8 by 2.2 cm and 8 mm deep (Fig. 16–9B). Because of persistent symptoms, size and depth of the lesion, and his desire to return to sports, he underwent fresh osteochondral allografting. A 3.0 cm by 9 mm dowel graft was harvested, fit into the prepared defect, and fixed with three absorbable pins (Fig. 16–9C).
The patient had an uneventful recovery. After 3 months of protected weight bearing, radiographs demonstrated osseous healing. He gradually returned to sports and recreational activities, resuming competitive baseball by 8 months after surgery.
FIGURE 16–9 (A) Anteroposterior (AP) radiograph of osteochondritis dissecans of the medial femoral condyle in a 17-year-old baseball player. (B) Osteochondral defect prior to allografting. (C) Medial femoral condyle with implanted allograft. Three PDS pins have been placed.
Results
The clinical outcome of fresh osteochondral allografting depends on the age and diagnosis of the patient, the size of the lesion, and the number of lesions grafted (Table 16–2).
We have reported a series of 69 knees in 66 individuals with OCD of the femoral condyle treated with fresh osteochondral allografts.21 All allografts were implanted within 5 days of procurement. Patients were prospectively evaluated using an 18-point modified D’Aubigne and Postel scale, and subjective assessment was performed with a patient questionnaire. In this group, there were 49 males and 17 females, with a mean age of 28 years (range 15–54). Forty lesions involved the medial femoral condyle, and 29 the lateral femoral condyle. An average of 1.6 surgeries had been performed on the knee prior to the allograft procedure. Allograft size was highly variable, with a range from 1 to 13 cm2. The average allograft size was 7.4 cm2. Two knees were lost to follow-up. Mean follow-up in the remaining 67 knees was 5.2 years (range 1–14). Overall, 50/69 (72%) knees were rated good or excellent, scoring 15 or above on the 18-point scale; 11/69 (16%) were rated fair; and 2/69 (3%) were rated poor. The average clinical score improved from 13.0 preoperatively to 15.8 postoperatively (p <. 01). Six patients had reoperations on the allograft: one was converted to total knee arthroplasty, and five underwent revision allografting at 1, 2, 5, 7, and 8 years after the initial allograft. Thirty-six of 66 patients completed questionnaires: 95% reported satisfaction with their treatment; 86% felt they were significantly improved. Subjective knee function improved from a mean of 3.2 to 7.8 on a 10-point scale. Garrett6 also reported on treating OCD with fresh allografts. At 2- to 9-year follow-up, 16/17 were reported successful.
FIGURE 16–10 (A,B) Anteroposterior and lateral radiograph 7 years after fresh allograft of lateral femoral condyle in 47-year-old man. Note integration of allograft and evidence of osteoarthrosis. Patient is a recreational triathlete.
Chu et al4 reported on 55 consecutive knees undergoing osteochondral allografting. This group included patients with diagnoses such as traumatic chondral injury, avascular necrosis, OCD, and patellofemoral disease. The mean age of this group was 35.6 years, with follow-up averaging 75 months (range 11–147 months). Of the 55 knees, 43 were unipolar replacements and 12 were bipolar resurfacing replacements. On an 18-point scale, 42/55 (76%) of these knees were rated good to excellent, and 3/55 were rated fair, for an overall success rate of 82%. It is important to note that 84% of the knees that underwent unipolar femoral grafts were rated good to excellent, and only 50% of the knees with bipolar grafts achieved good or excellent status. No realignment osteotomies were reported in this series. Many of the patients who underwent unipolar replacement were allowed to return to recreational and competitive sports (Fig. 16–10).
Ghazavi et al8 reported on 126 knees in 123 patients with posttraumatic osteochondral lesions of the tibia and femur at an average follow-up of 7.5 years. Eighty-five percent of patients were rated as successful; the remaining 18 patients had failed. Factors related to failure included age over 50 years, bipolar defects, malalignment, and workers’ compensation cases. Aubin et al9 later reported on the long-term results of 60 fresh femoral grafts implanted for posttraumatic lesions. These patients were a subgroup of the cohort reported by Ghazavi et al. Kaplan-Meier survivorship analysis showed 85% graft survival at 10 years and 74% survival at 15 years. Sixty-eight percent of patients underwent simultaneous realignment osteotomy, and 17% had concomitant meniscal transplantation.
Fresh Allograft Tissue
The fundamental principle of fresh osteochondral allografting is that fresh allografts, rather than frozen allografts, contain a high percentage of viable chondrocytes, and these chondrocytes will maintain the intact hyaline cartilage matrix. It has been shown in retrieval studies that viable chondrocytes are present for up to 17 years after implantation.14 In contrast, frozen allografts demonstrate little to no chondrocyte viability,13,22 and as a result matrix degeneration invariably ensues within a few years after implantation of frozen allografts.13 This experience supports the fundamental basis of utilizing fresh allografts, in spite of the increased difficulty in tissue recovery and processing. It is thought that the fresh graft provides the maximum chondrocyte viability, leading to the best long-term graft function. Historically, in allograft centers, transplantation has occurred within 7 days of tissue recovery; however, current fresh tissue banking protocols require a period of 14 days for completion of tissue testing. The issue, therefore, becomes one of balance between the safety of fresh tissue and deterioration of tissue during storage.
Storage studies have demonstrated that chondrocyte viability decreases with storage time, and particularly after 14 days of storage in a modified culture medium at 4°C. Between days 14 and 21, chondrocyte viability decreases from percents in the upper 90s to 70%. Additionally, viable cell density decreases dramatically. It has also been shown that sulfate uptake, a measure of metabolic activity of the tissue, declines significantly after only 7 days of storage.16,17
The issue of prolonged storage of fresh allografts is evolving. At the present time, there are no clinically relevant studies investigating the outcome of allografting utilizing grafts stored for longer than 7 days. It is therefore unclear whether there are any clinical consequences to prolonged storage. Clearly, further investigation is needed in this area. The storage of osteochondral allografts is an important component of the tissue banking aspects that are so unique to fresh osteochondral allografts. Few tissue banks have developed protocols to screen, recover, test, and process fresh allograft material. These processes are difficult and complex; however, they yield tissue that strikes a balance between safety and graft quality.
Allograft tissue donors are rigorously screened and tested, utilizing American Association of Tissue Banks guidelines.23 Nonetheless, it is important to recognize that fresh osteochondral allografts cannot be sterilized; more properly, they are harvested aseptically and tested for the presence of bacteria and viral disease. Historically, allografts, including fresh allografts, have been very safe; at our institution we have had no documented cases of disease transmission from allograft to recipient in over 20 years and 500 procedures. Disease transmission and infections from both fresh and frozen allografts have been reported.24 It is therefore critically important for surgeons to have a careful understanding of the process and the tissue bank with which they work, and communicate the appropriate risk to patients undergoing the procedure.
Immunologic Response to Fresh Allografts
The long-term function of fresh allografts depends on the osseous integration of the allograft to the host, the maintenance of the matrix by viable chondrocytes, and the relatively limited immune response and lack of frank rejection of the graft by the recipient. Several basic scientific studies have been performed investigating the immune response to fresh and frozen matched and mismatched osteochondral allografts. Stevenson25 demonstrated, in a canine study, that fresh canine leukocyte antigen—mismatched grafts generated the most vigorous immune response. Human studies include the histologic evaluation of retrievals of both functioning and failed allografts.20 These studies typically show osseous integration of the graft to the host with variable replacement and revascularization of the osseous portion of the graft, up to 90% chondrocyte viability, and no evidence of cell-mediated rejection such as lymphocyte infiltration. Conversely, in our series, we have measured anti—human leukocyte antigen (HLA) cytotoxic antibodies in the serum of patients. Approximately 50% of patients undergoing fresh osteochondral allografts generate these antibodies, presumably against the osseous or chondral component of the donor material. Although the clinical consequences of this response are not yet clear, we have demonstrated a correlation with the presence of these antibodies and inferior appearance on postoperative MRI.19 Additionally, over 90% of failed allografts in our series are antibody-positive. These findings suggest the need for further study on the immunologic response to fresh grafts. It is entirely possible that in the future we will have a better understanding of the immune behavior of fresh allografts, and perhaps perform some type of tissue matching or pharmacologic immune modulation.
Conclusion
Fresh osteochondral allografts are extremely useful for a wide spectrum of cartilage pathology, from the focal chondral lesion to extensive articular disease. Most commonly, in the athlete, allografts are utilized in the treatment of focal osteochondral lesions, such as those seen in osteochondritis dissecans, and as a salvage procedure when other cartilage restorative procedures have failed. Additionally, reconstruction of posttraumatic defects, such as those seen in periarticular fractures, is another common indication for fresh allografts in young, active individuals.
The allografting procedure takes advantage of the unique characteristics of both osseous and chondral tissue components. Uniquely, allografts can restore both osseous and chondral defects with a single graft material. Long-term clinical studies have demonstrated success rates of between 75% and 90% in focal femoral condyle lesions. Nonetheless, the allograft procedure presents unique clinical issues that require careful understanding prior to its undertaking. It is anticipated that in the future, advances in tissue banking, basic scientific investigations into the behavior of fresh allografts, and further study of clinical results will improve the outcome and more clearly define the indications for fresh osteochondral allografts.
REFERENCES
1. Lexer E. Joint transplantations and arthroplasty. Surg Gynecol Obstet 1925;40:782–809
2. Meyers MH, Akeson WA, Convery FR. Resurfacing of the knee with fresh osteochondral allograft. J Bone Joint Surg Am 1989;71A:704–713
3. Gross AE, McKee NH, Pritzker KP. Reconstruction of skeletal deficits of the knee: a comprehensive osteochondral transplant program. Clin Orthop 1983;174:96–106
4. Chu CR, Convery FR, Akeson WA, et al. Articular cartilage transplantation: clinical results in the knee. Clin Orthop 1999;36: 159–168
5. Bugbee WD,Jamali A, Rabbani R. Fresh osteochondral allografting in the treatment of tibiofemoral arthrosis. Proceedings of the 69th meeting of the American Academy of Orthopaedic Surgeons (AAOS), Dallas, TX, February 2002
6. Garrett JC. Fresh osteochondral allografts for treatment of articular defects in osteochondritis dissecans of the lateral femoral condyle in adults. Clin Orthop 1994;303:33–37
7. Bugbee WD. Fresh osteochondral allografts. Semin Arthroplasty 2000;11:1–7
8. Ghazavi MT, Pritzker RP, Davis AM, et al. Fresh osteochondral allografts for post-traumatic osteochondral defects of the knee. J Bone Joint Surg Br 1997;79B:1008–1013
9. Aubin PP, Cheah HK, Davis AM, Gross AE. Long term follow-up of fresh femoral osteochondral allografts for post-traumatic knee defects. Clin Orthop Relat Res 2001;391(suppl):318–327
10. Gross AE, Agnidis A, Hutchison CR. Osteochondral defects of the talus treated with fresh osteochondral allograft transplantation. Foot Ankle Int 2001;22:385–391
11. Kim CW, Tontz WL, Jamali A, Convery FR, Brage ME, Bugbee WD. Treatment of post-traumatic ankle arthrosis with bipolar tibiotalar osteochondral shell allografts. Foot Ankle Int 2002;23:1091–1102
12. Meyers MH. Resurfacing of the femoral head with fresh osteochondral allografts: long-term results. Clin Orthop 1985;197:111–114
13. Enneking WF, Campanacci DA. Retrieved human allografts: a clinicopathological study. J Bone Joint Surg Am 2001;83:971–986
14. Czitrom AA, Keating S, Gross AE. The viability of articular cartilage in fresh osteochondral allografts after clinical transplantation. J Bone Joint Surg Am 1990;72:574–581
15. Langer F, Gross AE. Immunogenicity of allograft articular cartilage. J Bone Joint Surg Am 1974;56A:297–304
16. Ball S, Amiel D, Williams SK, et al. The effects of storage on fresh human osteochondral allografts. Clin Orthop Relat Res 2004;418:246–252
17. Williams SK, Amiel D, Ball ST, et al. Prolonged storage effects on the articular cartilage of fresh human osteochondral allografts. J Bone Joint Surg Am 2003;85-A:2111–2120
18. Convery FR, Akeson WH, Meyers MH. The operative technique of fresh osteochondral allografting of the knee. Oper Tech Orthop 1997;47:340–344
19. Sirlin CB, Brossman J, Boutin RD, et al. Shell osteochondral allografts of the knee: comparison of MR imaging findings and immunologic responses. Radiology 2001;219:35–43
20. Oakeshott RD, Farine I, Pritzker KP, et al. A clinical and histologic analysis of failed fresh osteochondral allografts. Clin Orthop 1988;233:283–294
21. Bugbee WD, Emmerson BC, Jamali A. Fresh osteochondral allografting in the treatment of osteochondritis dissecans of the femoral condyle. Paper 054. Presented at the 70th annual meeting of the American Academy of Orthopaedic Surgeons (AAOS, New Orleans, LA), February 2003
22. Ohlendorf C, Tomford WM, Mankin HJ. Chondrocyte survival in cryopreserved osteochondral articular cartilage. J Orthop Res 1996;14:413–416
23. American Association of Tissue Banks. Standards for tissue banking. Arlington, VA: American Association of Tissue Banks, 1987
24. Centers for Disease Control. Update. Allograft-associated biochemical infections—United States, 2002. Morb Mortal Wkly Rep 2002;51: 207–210
25. Stevenson S. The immune response to osteochondral allografts in dogs. J Bone Joint Surg Am 1987;69:573–582
< div class='tao-gold-member'>
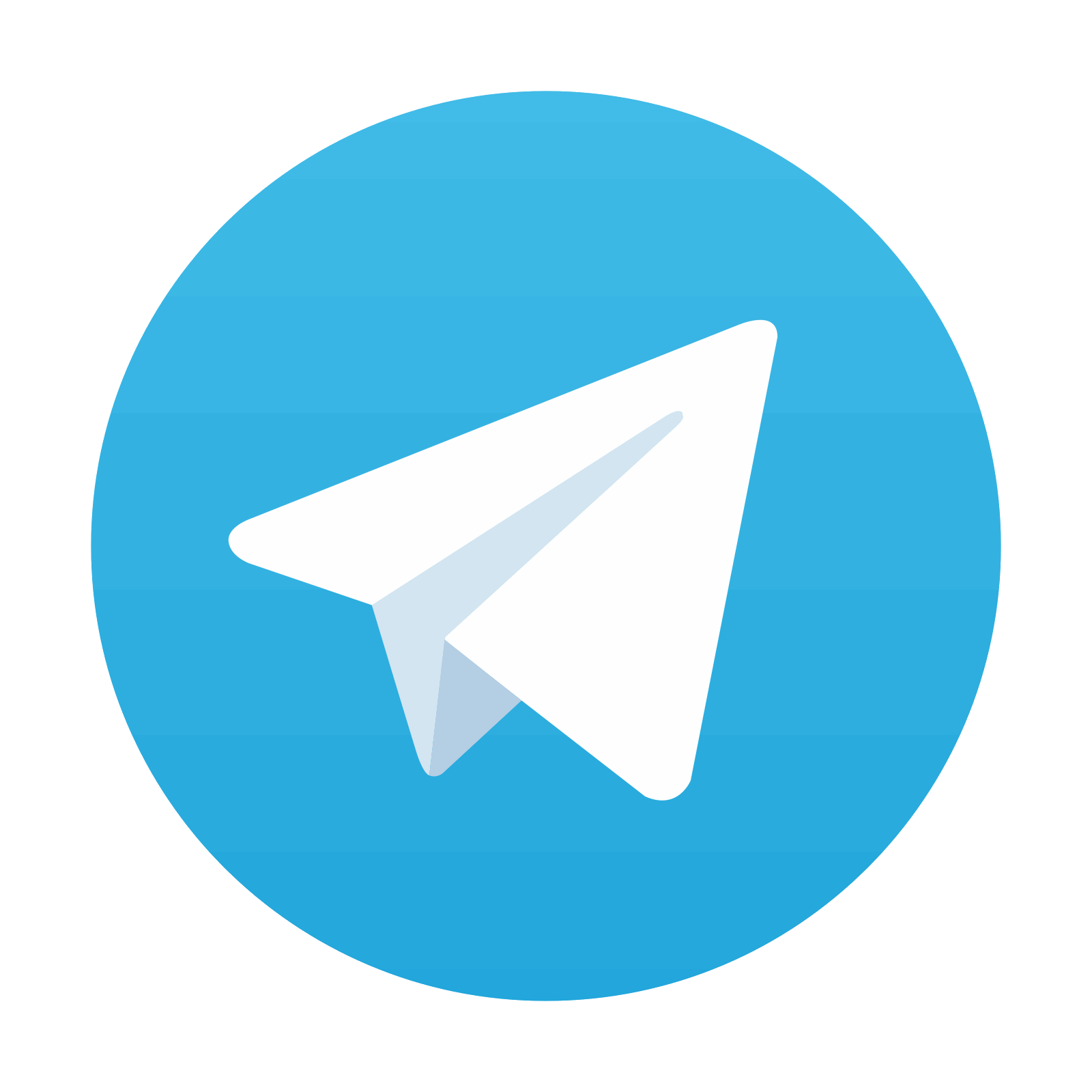