Oral Rehydration Therapy
Christopher Duggan
Julius G. K. Goepp
Mathuram Santosham
Acute gastroenteritis continues to be a leading cause of child mortality in the world. In developing nations, an estimated 1.5 to 2.5 million children younger than 5 years die annually from diarrhea and its complications. Although the total number of deaths from diarrhea is still unacceptably high, deaths due to diarrhea have been reduced dramatically in the past two decades. For instance, in 1982, an estimated 5 million deaths per year occurred, and in 1992, the estimated annual deaths fell to 3 million. More recent estimates are lower still.
Among children in the United States, acute gastroenteritis still accounts for approximately 300 deaths per year, 160,000 to 200,000 hospitalizations, and 1.5 million physician visits, all accounting for approximately $1 billion in direct medical costs. Although most diarrheal episodes among U.S. children are mild, the resulting physicians’ visits produce substantial health care costs.
Over the past 30 years, considerable progress has been made in establishing the etiologic agents of acute gastroenteritis and the pathophysiology of diarrheal dehydration. In particular, an improved understanding of fluid and electrolyte transport in the gastrointestinal tract has led directly to the development of physiologically appropriate solutions for oral fluid therapy. Such therapy, in combination with appropriate nutritional management of the child with gastroenteritis, has come to be known as oral rehydration therapy (ORT).
Early attempts at treating dehydration resulting from diarrhea were described in the medical literature in the 1830s during epidemics of Vibrio cholerae infections. These attempts at parenteral fluid therapy were largely unsuccessful because of inadequate aseptic technique and poor equipment and because the physiologic effects of diarrhea and volume depletion in humans were poorly understood. Even those patients who improved with this therapy worsened when therapy was discontinued, and more than 75% still died. By 1926, awareness of treating acidosis in dehydration led Powers to administer solutions of glucose, saline, and bicarbonate, along with blood; fasting also was recommended. He reported a reduction in the mortality rate among hospitalized patients to 33%. During the ensuing 2 decades, the use of saline solutions with dextrose, accompanied by enforced fasting, was ineffective at further reducing the hospital mortality rate below 30%. In 1946, Darrow and Harrison, following careful balance studies of salt and water losses, added potassium to rehydration fluids and reported a mortality rate as low as 6%, still in fasting children.
Oral electrolyte solutions were pioneered in the 1940s by Harrison. The first commercially available solution (Lytren, Mead, Johnson Co.) was developed in the 1950s. Simultaneous reports of hypernatremic dehydration and increased rates of mortality led to a widespread distrust of ORT among pediatricians in the United States. In fact, the epidemic of hypernatremia was attributable to the inappropriate management of diarrhea and to the packaging of Lytren itself, not to intrinsic ORT problems: (a) Boiled skimmed milk was recommended frequently for use in diarrhea, and its high osmolality contributed to high serum sodium values; (b) Lytren was sold in bulk to be mixed at home, and parents often mixed the solution
improperly; and (c) Lytren contained 8% glucose, which made it excessively osmotic (see following discussion of the osmolarity of oral rehydration solutions). A valuable lesson learned from the 1950s experience is that ORT use must be appropriately taught to caregivers and that proper feeding practices should be followed during diarrhea, not that ORT itself is a dangerous treatment.
improperly; and (c) Lytren contained 8% glucose, which made it excessively osmotic (see following discussion of the osmolarity of oral rehydration solutions). A valuable lesson learned from the 1950s experience is that ORT use must be appropriately taught to caregivers and that proper feeding practices should be followed during diarrhea, not that ORT itself is a dangerous treatment.
In the 1950s and 1960s, laboratory models of fluid and electrolyte transport in mammalian intestines, combined with improved measurements of stool electrolyte losses in cholera, led to the development of the first oral solutions truly tailored to the needs of patients with gastroenteritis, specifically cholera. Subsequently, numerous studies have documented the effectiveness of ORT among children and adults with dehydration from gastroenteritis caused by a variety of infectious agents.
The critical role of nutrition during diarrhea episodes has only been relatively recently appreciated. Although Park in 1924 and Chung in 1948 were among the first to challenge the notion that feedings should be withheld during diarrhea, their views were not accepted until studies in the 1980s demonstrated improved outcomes with continued feeding during diarrhea episodes.
Management of gastroenteritis and dehydration has moved to the forefront of pediatric medicine over several decades. Practice guidelines emphasizing ORT as a cornerstone of therapy were released by the Centers for Disease Control and Prevention (CDC) in 1992 and 2003. The American Academy of Pediatrics (AAP) Practice Parameter for the Management of Acute Gastroenteritis in Young Children identifies ORT as the preferred treatment of children with mild to moderate dehydration caused by diarrhea. Paradoxically, ORT is used least in the industrialized countries where much of the original basic scientific research was done. Like any therapy, ORT relies on appropriate teaching and implementation for its ultimate effectiveness. In the balance of this chapter, we describe the pathophysiology of gastroenteritis, the mechanisms of action of ORT, and practical aspects of ORT delivery, particularly in an industrialized world setting.
PATHOPHYSIOLOGY AND ETIOLOGY OF ACUTE DEHYDRATING GASTROENTERITIS
Although the terms dehydration and rehydration strictly refer to loss and replacement of water alone, they are used commonly to reflect overall fluid and electrolyte status. More accurately, a patient with diarrhea usually has sustained volume depletion, reflecting losses not only of water but of sodium and other electrolytes as well. Initial losses of sodium during gastroenteritis are from the extracellular fluid compartment (ECF). With progressive loss of circulating volume and total body potassium depletion, sodium is also shifted from the ECF into the intracellular fluid compartment. Thus, regardless of the measured serum sodium or potassium concentration, total body sodium and potassium content is invariably diminished during dehydration from gastroenteritis.
Pathophysiologic Considerations
Intestinal Absorption
A grasp of basic intestinal physiology is vital to understanding the concepts underlying fluid therapy of the volume-depleted patient. Here, we consider absorption of various substances from the bowel and their physiologic roles in homeostasis.
Fluids and electrolytes are secreted at a tremendous rate in the healthy bowel (approximately 9 L daily in adults). Therefore, powerful mechanisms for reabsorption must be present; otherwise, rapid volume depletion would ensue. The normal flow of fluid into and out of the bowel lumen creates a circulatory pattern: Secretions from salivary glands, the pancreas, and the gallbladder are added to the intrinsic bowel secretions to digest nutrients, and the vast bulk of fluid, as well as sodium and other electrolytes, is rapidly reabsorbed by mechanisms residing in the epithelial cells of the small and large bowel. This complex system is modulated by the interactions of hormonal and intracellular mediators common to most tissues: the adenosine and guanosine nucleotide messenger systems, intracellular calcium, and metabolites of arachidonic acid (leukotrienes and prostaglandins).
The immediate driving force for active water absorption in the bowel is the movement of sodium. Uptake of sodium occurs by means of a two-step process, the first involving active pumping of the ion out of intestinal epithelial cells at the basolateral membrane, followed by sodium entry down the resulting gradient from the bowel lumen into the cell (Fig. 350.1). Sodium entry also occurs passively by several ion-coupled mechanisms: sodium–hydrogen exchange and coupled sodium chloride absorption. Sodium cotransport with small organic molecules such as glucose and amino acids also occurs. Although various mechanisms and ratios of sodium to organic substrate have been proposed, the clinical relevance resides in the fact that uptake of sodium and therefore water is dramatically increased when organic substrate is present with sodium at a molecular ratio of approximately 1:1 in the intact intestine. This observation is exploited in the design of oral rehydration solutions (ORSs).
Modulation of sodium uptake and water absorption occurs in part by the effects of aldosterone on sodium channels in enterocyte membranes. Elevated aldosterone levels result in increased sodium absorption. Antidiuretic hormone and glucocorticoids also affect water and salt uptake from bowel. Catecholamines have profound acute stimulatory effects on sodium absorption.
Absorption of other electrolytes is also important, but these substances play a smaller direct role in the movement of fluid, except as modulators of sodium uptake. Luminal potassium rapidly equilibrates with serum levels; even a severely potassium-depleted patient may continue to lose potassium in the stool. Additionally, elevated aldosterone in dehydration
contributes to urinary potassium losses. Bicarbonate is secreted in substantial quantities by the pancreas and must be reabsorbed to maintain systemic pH. Luminal bicarbonate stimulates absorption of sodium as well.
contributes to urinary potassium losses. Bicarbonate is secreted in substantial quantities by the pancreas and must be reabsorbed to maintain systemic pH. Luminal bicarbonate stimulates absorption of sodium as well.
Features of Intestinal Secretion
As with water and electrolyte absorption, secretory processes in the mammalian intestine occur by various mechanisms that are responsive to a host of intrinsic and extrinsic modulators. Rates of secretion between the small and large intestine vary greatly; therefore, we discuss each portion of the bowel individually and then describe the action of various substances on secretion in the intestine as a whole.
In the healthy gastrointestinal tract, net fluid absorption exceeds secretion, whereas in dehydrating diarrheal illness, net intestinal losses exceed absorption. Such net loss may result from increased secretion in the proximal gut or from diminished absorption distally, or both. Each of the substances considered here may act on one or more of the secretory or absorptive processes to affect this balance. Their mechanisms of action are briefly discussed here.
Secretion in the Small Intestine
Most absorptive processes in the small intestine occur in cells at the villous tip, whereas secretion takes place chiefly in crypt cells. The secretion of chloride ion is a major determinant of fluid movement into the gut lumen. Chloride secretion occurs by energy-requiring, pump-mediated entry at the basal cell membrane, with subsequent conductive, channel-mediated exit at the apical membrane.
Control and modulation of chloride secretion in the small bowel depend on the interrelationship of several intracellular messenger systems, which appear to be fundamental signaling processes in most enterocytes. Known mediators of chloride secretion include free calcium ion (Ca2+), cyclic adenosine monophosphate (cAMP), cyclic guanosine monophosphate (cGMP), and intracellular pH. Various endocrine and paracrine substances exert their ultimate effects on intestinal secretion by affecting one or more of these messenger systems.
Regulation of Absorption and Secretion in the Colon
The mammalian colon shares certain features of fluid and electrolyte transport with the small intestine but differs in a number of significant aspects. The rate and volume of electrolyte and water absorption are segmentally heterogeneous in the colon and may depend on volume, composition, and rate of flow of the luminal contents. Colonic sodium recovery occurs across a gradient three to four times greater than that found in the jejunum and responds to both mineralocorticoid and glucocorticoid effects.
Increased fecal water excretion in diarrhea may result from increased small or large intestinal fluid secretion, from diminished capacity of the colon to absorb water and electrolytes, or from a combination of these factors. Such effects may be mediated by a number of exogenous substances, which are covered in the following discussion, after a brief discussion of colonic mechanisms for normal absorption and secretion.
Under normal circumstances, the colon absorbs sodium and chloride by mechanisms similar to those in the small bowel and secretes potassium and bicarbonate in substantial quantities. Unlike the small bowel, the colon lacks important mechanisms for the active cotransport of glucose, amino acids, and sodium beyond the neonatal period.
Intracellular mediators of secretion in the colon include those discussed previously for the small intestine: calcium, cAMP, and cGMP. cAMP appears to increase colonic secretion of chloride and potassium, probably by increasing apical membrane conductances. Additionally, cAMP reduces net sodium chloride absorption. The effects of increased intracellular calcium are similar to those in the small intestine, and evidence exists that increased cAMP may result in elevation of intracellular concentrations of Ca2+. cGMP may also play a role in colonic electrolyte modulation, but its effects are less well understood than those of calcium or cAMP.
As in the small bowel, the metabolites of arachidonic acid, prostaglandin (PG) E and leukotrienes, play a role in the regulation of colonic fluid and electrolyte movement. These arachidonic acid metabolites serve as intermediaries that increase cAMP levels and exert a direct effect by some as yet unspecified intracellular mechanism independent of adenylate cyclase.
Etiologic Agents of Diarrhea: Mechanisms of Action
A variety of intrinsic and extrinsic agents pathologically stimulate secretion in the small or large intestine. These substances may be conveniently divided into three categories: (a) a single group of intrinsic biochemical signals (hormones, neurotransmitters, and mediators of inflammation), (b) extrinsic biologic pathogens (bacteria, viruses, and enterotoxins), and (c) extrinsic chemical secretagogues (laxatives). Each of the clinically important members of these categories appears to have its ultimate effect on one of the final common intracellular mediators discussed previously—calcium, cAMP, or cGMP.
The effects of enterotoxins on secretory processes are most prominent in the small bowel. Many of these agents act by raising intracellular levels of cAMP; they are all heat-labile multiunit toxins that bind and activate cellular adenylate cyclase. The ultimate effect of these intracellular changes is that secretion in crypt cells is switched on, whereas absorption in villous cells is switched off. Toxins that act by this mechanism include the heat-labile V. cholerae and Escherichia coli toxins, as well as those produced by certain strains of Salmonella, Campylobacter, and possibly Shigella.
A second group of toxins appears to function by stimulation of the guanylate cyclase pathway. This group comprises the heat-stable toxins of E. coli, Yersinia enterocolitica, and Klebsiella pneumoniae. Evidence suggests that the effect of the toxins is mediated in part by calcium and PGE, which in turn stimulate cGMP production.
Effects of enterotoxin on fluid and electrolyte transport in the colon are less well understood and may be less important clinically in water loss from diarrhea. Nonetheless, reduced sodium chloride absorption in cholera has been suggested. In addition to the enterotoxin effects, bacterial pathogens stimulate a host inflammatory response, resulting in release of mediators such as PGE and leukotrienes. These act directly as stimulators of secretion and indirectly as modulators of intracellular second messengers.
Viral agents such as rotavirus appear to exert their pathologic effects by inducing sloughing of intestinal villous (i.e., absorptive) cells in excess of crypt (i.e., secretory) cells. Because the damage to villous cells is patchy and absorption in the surviving cells is intact, oral fluid replacement in such cases is usually still effective.
ORAL REHYDRATION SOLUTIONS
ORT is based on glucose–sodium cotransport in the intestinal epithelial cell.
Water passively follows the osmotic gradient generated by the transcellular transport of electrolytes and nutrients. While three principle mechanisms of sodium absorption have been described, the mechanism essential to the efficacy of ORSs was
shown to result from the coupled transport of sodium and glucose molecules at the intestinal brush border (Fig. 350.1). Cotransport across the luminal membrane is facilitated by the protein SGLT1 (sodium glucose cotransporter 1). Once in the enterocyte, the transport of glucose into the blood is facilitated by GLUT2 (glucose transporter type 2) in the basolateral membrane. The Na+-K+-ATPase provides the gradient that drives the process. Clinical studies have demonstrated that this mechanism remains intact even in patients with severe diarrhea.
shown to result from the coupled transport of sodium and glucose molecules at the intestinal brush border (Fig. 350.1). Cotransport across the luminal membrane is facilitated by the protein SGLT1 (sodium glucose cotransporter 1). Once in the enterocyte, the transport of glucose into the blood is facilitated by GLUT2 (glucose transporter type 2) in the basolateral membrane. The Na+-K+-ATPase provides the gradient that drives the process. Clinical studies have demonstrated that this mechanism remains intact even in patients with severe diarrhea.
Composition of Standard Oral Rehydration Solutions
In 1975, the World Health Organization (WHO) and the United Nations Children’s Fund (UNICEF) agreed to promote a single ORS (WHO-ORS) containing (in mmol/L) sodium 90, potassium 20, chloride 80, citrate 30, and glucose 111 (2%) for use among diverse populations in different countries. This composition was selected to allow for a single solution to be used for treatment of diarrhea caused by a variety of infectious agents and associated with varying degrees of electrolyte loss. WHO-ORS has been proven for over 30 years to be safe and effective at rehydration and maintenance for children and adults with all types of infectious diarrhea.
A review of the separate components of ORS is helpful in understanding the rationale behind a new and possibly improved version of ORS. The inclusion of sodium and glucose are of course necessary for the cotransport mechanism noted previously, which drives water into the cell. Promotion of sodium cotransport can occur over a range of glucose concentrations (from 10 to 25 g/L, or 56 to 140 mmol/L). However, when glucose concentrations exceed 30 g/L (3%), as is the case with most fruit juices and sodas, osmotic forces may counteract the cotransport phenomenon and draw water back into the gastrointestinal lumen.
The sodium component of ORSs has engendered much research and debate. Since early experience with ORSs originated in areas of the world where cholera was an important cause of diarrhea, early oral solutions contained 100 to 120 mEq/L of sodium to mimic the electrolyte losses of an actively purging cholera patient. Since sodium losses in noncholera diarrhea are lower, the utility of these original solutions for viral and other gastrointestinal infections was questioned by some. Controlled clinical trials later confirmed that solutions with 90 or 75 mEq/L (or even lower amounts) of sodium worked well as a rehydration solution for noncholera diarrhea, as long as adequate replacement fluid was given. However, the unique programmatic advantage of being able to prescribe a single solution for all causes of diarrhea (cholera and noncholera) led the WHO and other policy-making bodies to argue for the deployment and use of a solution with a single composition. Recently, a solution with a sodium concentration of 75 mEq/L has been recommended for global use (see below).
Potassium is needed since stool losses of this electrolyte can be compounded by urinary losses in the setting of increased aldosterone levels, and hypokalemia is a well-recognized complication of diarrhea. With severe hypokalemia, a paralytic ileus can ensue and reduced intestinal fluid absorption can follow. Most ORSs contain 20 mmol/L of potassium, which is an amount that can only begin to replace potassium losses of the child with diarrhea. Additional sources of potassium from diet are needed.
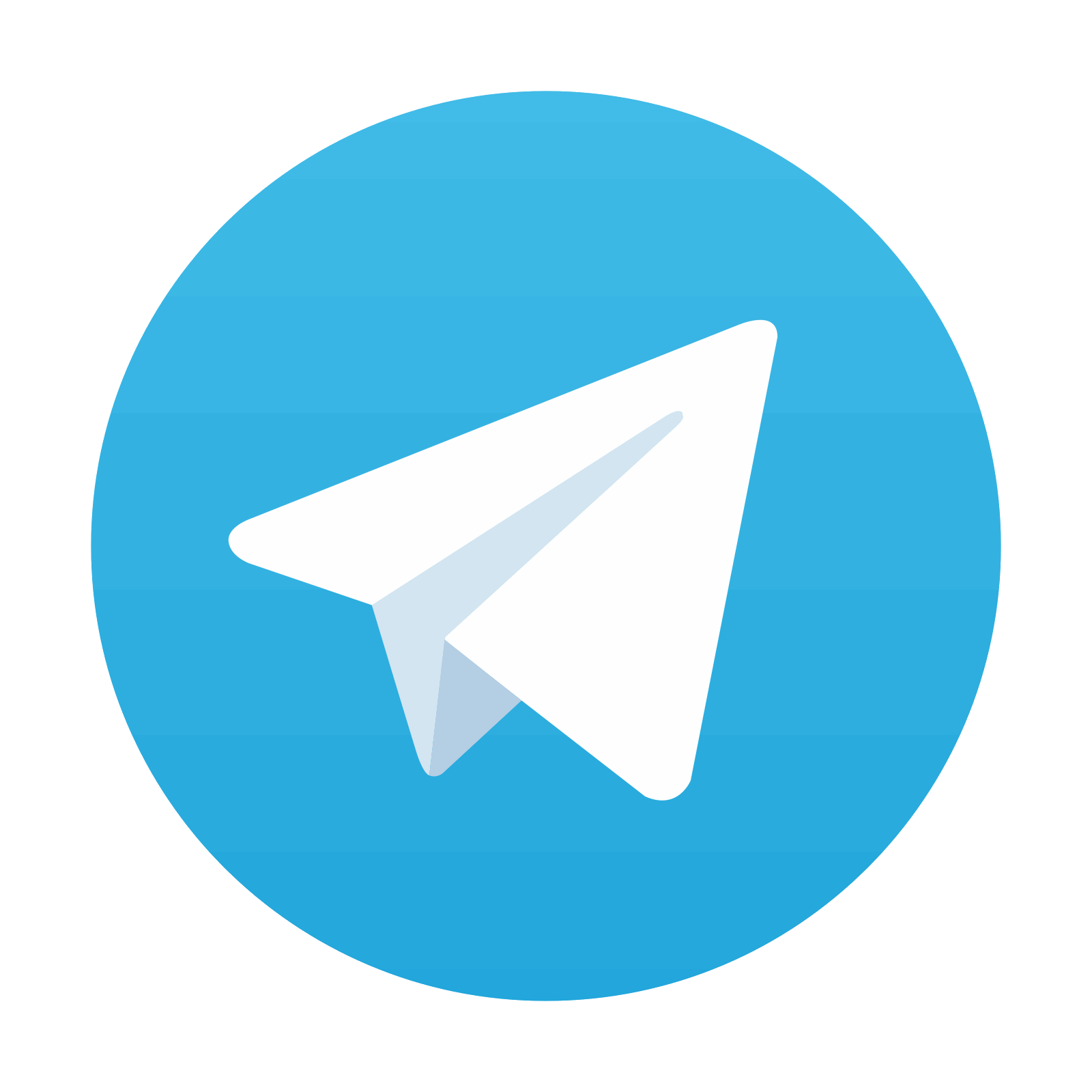
Stay updated, free articles. Join our Telegram channel

Full access? Get Clinical Tree
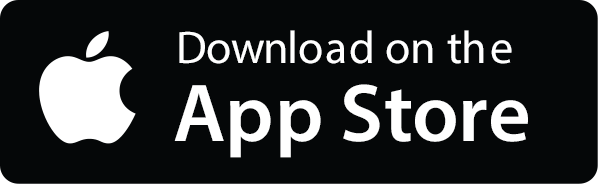
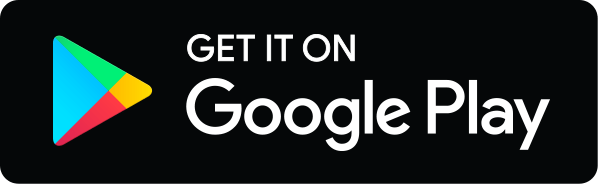