Fig. 9.1
Algorithm for the acute management of open fractures (Used with permission from Slack Orthopedics: Mauffrey et al. [116])
Initial Debridement
Timing
In 2009, the guidelines on the management of open tibial fractures from the British Orthopaedic Association (BOA) and the British Association of Plastic, Reconstructive and Aesthetic Surgeons (BAPRAS) were modified to change the recommended timing of debridement from within 6 h of injury to within 24 h of injury [15]. In fact, the historical cutoff of 6 h was based on scarce evidence.
More recently, new clinical evidence has arisen supporting the change in practice from emergent debridement to debridement within 24 h. Patzakis and Wilkins looked at the relation between infection rate and timing of debridement. Seven percent was the rate of infection in both groups treated within 12 h of injury or more than 12 h after their open fracture [16]. Other authors confirmed the lack of correlation between urgent debridement and reduced infection rates both in humans [17–19] and in animal models [20].
It appears that the most important aspect of management of open fractures in reducing the risk of infection is by the early initiation of prophylactic intravenous antibiotics. The timing of debridement does not appear to relate to the development of deep infection if performed within 24 h.
Surgical Technique
In 2010, the British Orthopaedic Association (BOA) and the British Association of Plastic, Reconstructive and Aesthetic Surgeons (BAPRAS) working party on the management of open tibial fractures [15] agreed on a protocol that is now followed nationwide across the United Kingdom. This includes antibiotic prophylaxis but also a well-defined step-by-step approach for initial wound debridement that includes:
Removal of all dead tissue.
Systematic debridement in the following sequence:
Application of a pre-prep with a soapy solution.
Preparation of the limb with a chlorhexidine alcohol solution, avoiding direct contact of the chlorhexidine with the open wound.
Wound extensions are done ideally following potential fasciotomy incisions.
Systematic assessment of the tissues, from superficial to deep and from the periphery to the center of the wound.
In order to ensure bone viability during the debridement process, deflation of the tourniquet should be performed to assess bleeding of the bony segment. At this stage, nonviable fragments or loose fragments of bone with no attachment to soft tissue should be discarded.
Once the debridement has been performed, thorough irrigation can be achieved.
It is only following this radical and systematic approach that the injury can be classified and a multidisciplinary treatment plan be elaborated by the orthopedic and microvascular surgery teams.
Acute Wound Management
Delayed vs. Primary Wound Closure
When closing the tissue defect created by an open fracture, the surgeon much choose whether to close it soon after the injury or wait and close it later as a means to control potential infection. Primary closure, defined as approximation of wound edges immediately following debridement or cleaning within 6 h of injury, has the benefit of rapid wound healing but presents the threat of increased wound infection. Delayed closure, defined as approximation of wound edges more than 48 h after debridement or cleaning, is often used for wounds judged to be “dirty” or contaminated—i.e., with a risk of infection perceived to be higher due to the environment and circumstances surrounding the injury. However, no good evidence exists to guide this decision; as of July 2011, a review of the Cochrane Database by Eliya and Banda indicated there were no randomized controlled trials comparing primary versus delayed wound closure [21].
Negative Pressure Wound Therapy
Negative pressure wound therapy, in which vacuum suction is applied across an airtight topical dressing, has been used in the treatment of chronic and surgical wounds. The negative pressure is thought to aid the drainage of excess fluid, reduce infection rates, and increase localized blood flow. It is also known as topical negative pressure (TNP) therapy, vacuum-assisted closure (VAC), and sealed surface wound suction (Fig. 9.2). A systematic review in 2008 by Ubbink et al. found only a small number of flawed trials and thus little evidence to support the use of negative pressure wound therapy in the treatment of wounds [22].
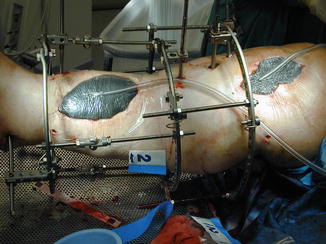
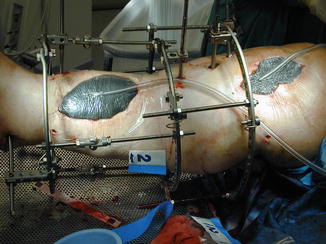
Fig. 9.2
Application of vacuum-assisted closure (VAC) dressing
In Situ Antibiotics
The use of antibiotic beads is advocated by Ostermann and Seligson in a paper reviewing 1,085 consecutive cases of severe open fractures [23]. The first group was managed solely with systemic antibiotics and debridement at the time of presentation, while the second group was supplemented with local aminoglycoside-polymethylmethacrylate (PMMA) beads. The difference between the overall infection rates in both groups was shown to be statistically significant (p < 0.001), with a 12 % infection rate in patients managed with IV antibiotics and debridement only versus a 3.7 % infection rate in the group managed with additional placement of antibiotic beads. The authors also analyzed fracture subtypes and concluded that while both acute infection and osteomyelitis were reduced in the second group for all fractures types overall, the reduction reached the level of statistical significance only for type IIIB and IIIC fractures for acute infection and type II and IIIB fractures for chronic osteomyelitis (Fig. 9.3).
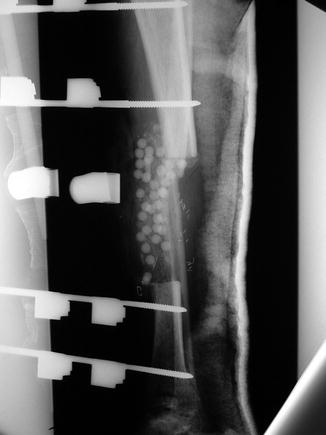
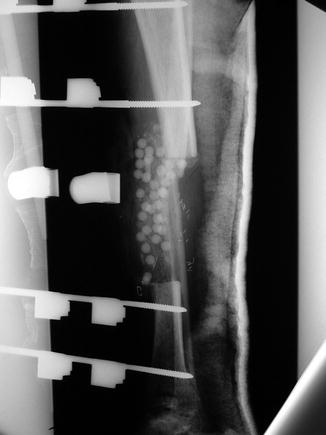
Fig. 9.3
Lateral radiograph of a tibia with segmental bone defect and antibiotic beads placement
Other papers have shown similar results. Henry et al. treated 404 open fractures, with similar distributions of fracture types. Around two thirds of patients were treated with IV antibiotics and tobramycin antibiotic bead chains, while the remaining third was treated with IV antibiotics only. The group supplemented with antibiotic beads had a statistically significant lower rate of infection (4.2 %) than the group that did not receive beads (21.4 %) [24, 25].
Fracture Stabilization in the Acute Setting
The surgeon has several options to stabilize an open fracture, including techniques commonly employed during an immediate fracture presentation such as splinting, casting, and traction, and other operative interventions like external fixation, plating, and intramedullary nailing. The choice of methods is dependent upon a number of factors, including the bones involved, the location, and qualities of the fracture, and several studies have attempted to address this question specifically, as Okike and Bhattacharyya summarize in their 2006 article [26].
Fractures of the Femur
Beginning with a report by Winquist et al. in 1984, early intramedullary nailing has been shown to be effective in the treatment of femoral shaft fractures [27]. Okike and Bhattacharyya identified several articles studying early intramedullary nailing with reaming for open femoral shaft fractures. A prospective, randomized, controlled trial by Bone et al. in 1989 compared early (within 24 h) and late (after 48 h) stabilization of 178 femoral fractures, which demonstrated no differences for patients with isolated injuries but decreased complications for poly-trauma patients. Specifically, these patients, when undergoing stabilization within 24 h, were shown to have a decreased rate of pulmonary complications, shorter hospital stays, and less time in the ICU [28]. In 1988, Lhowe and Hansen concluded that immediate intramedullary nailing could be accomplished safely with an acceptable rate of complications after their series of 67 patients with open femoral shaft fractures. Only 2 of 67 patients suffered a wound infection, and all fractures healed within 4 months of injury [29]. Brumback et al. published a series of 89 open femoral fractures stabilized by intramedullary nailing with reaming and reported no infections among 62 type I, II, and IIIA fractures. Of the 27 type IIIB fractures, only 3 became infected, and the rate of infection did not differ for patients treated early (within 24 h) or late (after 48 h) [30]. More recently, 2009 study by Taitsman et al. identified open fractures as a risk factor for nonunion for fractures treated by intramedullary nailing [31], but the preponderance of evidence still supports early reamed intramedullary nailing as the preferred method for treatment of open femoral shaft fractures.
Fractures of the Tibia
The current literature favors intramedullary nailing for fixation of open tibia fractures. In their 2006 paper, Okike and Bhattacharyya chronicle the evolution of opinion regarding treatment for open tibia fractures. They list two articles written in the 1980s that addressed external fixation in tibia fractures: Edwards et al. concluded that external fixation was successful for treatment of severe open tibia fractures [32], and Bach and Hansen [33] reported fewer complications with external fixation than with internal plate fixation. The 1990s saw a shift away from external fixation toward intramedullary nailing, and Okike and Bhattacharyya point out several studies making this argument. Henley et al. found fewer incidences of malalignment (8 % vs. 31 %, p < 0.001), fewer subsequent procedures (mean 1.7 vs. 2.7, p = 0.001), and lower rate of infection (13 % vs. 21 %, not significant) comparing 104 patients treated with unreamed intramedullary nailing to 70 patients treated with external fixation [34]. Schandelmaier et al. reported a retrospective study of 114 patients with fresh tibial shaft fractures with severe soft tissue injury; 48 were treated with unreamed tibia nails and 66 were treated with external fixators. The unreamed tibia nail group underwent fewer subsequent procedures and achieved better functional outcomes, though the authors articulate that this result may not hold true for patients with less severe injuries [35]. Similarly, in 1994 Tornetta et al. compared intramedullary nailing with external fixation in a randomized, controlled trial of 29 patients with severe (grade IIIB) open tibia fractures. Though they detected no significant differences in healing or range of motion, they considered intramedullary nailing superior due to patient preference and easier fracture management [36]. More recently, the usefulness of external fixation for “damage control orthopedics” has been described by Lebel et al. following their experiences in the aftermath of the 2010 Haiti earthquake [37]. Furthermore, a review by Bhandari et al. has shown that intramedullary nailing after external fixation achieves good union and infection rates and may be superior to casting for definitive treatment, as casting has similar rates of infection but significantly higher rates of nonunion. Removal of the external fixator within 28 days may reduce the risk of infection by 83 % [38].
There has been some controversy as to whether intramedullary nails should be inserted reamed or unreamed, and several smaller trials were inconclusive [39–41]. A meta-analysis by Bhandari et al. in 2001 presented strong evidence in favor of intramedullary nailing over external fixation but failed to find any definitive evidence for reamed versus unreamed nailing, suggesting a much larger study was necessary to analyze the question [42].
Accordingly, the SPRINT trial, a multicenter, blinded randomized trial completed in 2007 and reported in 2008, studies reamed versus unreamed nails in both closed and open tibia fractures on a much larger scale. One of the largest orthopedic studies ever conducted, over 1,226 patients with 1,248 fractures of the tibial shaft were included. Of these, 400 patients with 406 open fractures (108 [26.6 %] Gustilo type I, 161 [39.7 %] Gustilo type II, 107 [26.4 %] Gustilo type IIIA, 30 [7.4 %] Gustilo type IIIB) were treated with reamed (210 total) and unreamed (196 total) intramedullary nails. As its primary outcome, the study evaluated reoperation and/or autodynamization within 1 year. A total of 106 patients experienced a primary event (reoperation)—60 from the reamed group and 46 from the unreamed group. There was no statistically significant difference between these two groups (p = 0.16), and the study concludes that the optimal nailing technique for open fractures remains uncertain [43].
Soft Tissue Reconstruction
Extremity soft tissue defects continue to be a challenging problem for orthopedic trauma surgeons and complicate the management and salvage of severe extremity injuries. In the last decades, an increase of civilian high-energy trauma and significant battlefield trauma together with improved trauma survival has increased the incidence of complex extremity wounds, necessitating soft tissue reconstruction. Open fractures are associated with higher rates of infection, malunion, nonunion, as well as limb loss [44]. The individuality of each injury and patient characteristics call for custom solutions. Limb salvage has to be seen in context with the injury profile of the patient and expected functional demands. Traumatologists need to closely coordinate damage control surgery, definite fixation, and soft tissue reconstruction in order to achieve optimal outcome. This calls for a close interdisciplinary cooperation between orthopedic trauma specialist and reconstructive plastic surgeons or special, orthoplastic trained surgeons. This section will give an overview of current algorithms and options for soft tissue reconstruction in the lower extremity. The continuous development and refinement in flap reconstructions and increased utilization of adjuvant techniques such as vacuum-assisted closure have changed the reconstructive practice and will be highlighted.
General Principles
Epidemiology
The majority of extremity soft tissue defects is seen in the lower extremity and overwhelmingly related to trauma. Most commonly these injuries occur after motor vehicle accidents followed by penetrating injuries, blast trauma, and burn injuries. Soft tissue loss due to management of necrotizing infections and tumor resections often necessitate soft tissue reconstruction. The focus of this review will be on traumatic injuries.
Indication for Soft Tissue Reconstruction
The indication and decision for soft tissue reconstruction arise after formal debridement of the traumatic wound at which point a decision regarding extent, depth, and involvement of vital structures can be made. Many mangling limb injuries in a multi-injured patient preclude salvage based on ATLS principles of life before limb. The indication for reconstruction can only be seen in a stabilized patient.
Most commonly, open fractures and associated soft tissue injuries are classified according to the Gustilo and Anderson classification (Table 9.1) [45].
Table 9.1
Gustilo and Anderson Classification for open fractures
Type | Definition |
---|---|
I | Open fracture, clean wound, wound <1 cm in length |
II | Open fracture, wound >1 cm in length, limited soft tissue damage |
III | Higher-energy open fractures with extensive soft tissue injury |
IIIA | Type II, adequate periosteal coverage of the bone, primary closure possible |
IIIB | Type II, extensive soft tissue loss necessitating soft tissue management including flaps |
IIIC | Type III, arterial injury requiring emergent repair, irrespective of soft tissue injury severity |
The indication for soft tissue reconstruction in the lower extremity is usually seen in IIIB type injuries with extensive soft tissue defects, which cannot be closed without soft tissue management or flaps and in the majority of IIIC injuries, which are soft tissue injuries with an arterial injury necessitating vascular repair for perfusion of the foot. In addition to this classification concept, the type of defect, location, and exposed structures dictate the scale and complexity of reconstruction. Generally speaking: Exposed neurovascular bundles, tendons, bone, and joints mandate in most instances flap coverage. This said, full thickness skin loss in functionally important regions such as the popliteal and antecubital fossa and the wrist/hand benefit from early, higher complexity reconstructions, such as a flap surgery instead of skin grafting, to provide for full thickness coverage of vital structures and allow better functional rehabilitation, prevention of contractures, and disability.
Indication for Primary Amputation
The question of amputation versus salvage has been extensively addressed by the LEAP study consortium, which showed similar functional outcomes over time in regard to patients with mangled lower extremity injuries treated with either amputation or salvage [46]. The group found significantly higher lifetime costs associated with amputations. There was no significant difference in the two groups with regard to return to work or patient satisfaction scores. The research highlighted the significant impact soft tissue injuries had on the decision for or against salvage. The evidence gained from this study showed a poor predictive value and clinical utility of existing extremity injury scores in the decision of limb salvage versus amputation. It was found that mangled extremities with initially insensate feet regained a protective level of sensation and were of benefit to patients. Thus the denervated foot cannot be seen as an indication for amputation [47].
Clear indications to amputate are currently seen:
Whenever there is an imminent risk of death. For the lower extremity injured patient, uncontrollable hemorrhage is the foremost indication to amputate. This is seen in mangled and blast-type injuries inflicted by IED and mines.
In limb ischemia times greater than 6 h, by which time myolysis and tissue necrosis has set in. Attempts of salvage could expose the patient to ischemia reperfusion injury [48].
Segmental limb loss in the lower extremity of greater than one-third of length.
Incomplete amputations with a mangled distal part.
Timing
Soft tissue reconstruction has to follow resuscitation and stabilization of the patient. In the case of a monotrauma to a limb, early or immediate reconstruction is feasible and had been initially propagated by Godina, who described favorable results with one stage—primary free flap reconstructions for complex injuries [49]. This has to be differentiated from emergent flap reconstruction in IIIC injuries where a combined vascular and flap reconstruction is performed to revascularize a limb. Some authors have termed all flap surgeries performed within 24 h after trauma as “emergency” flaps; this practice dilutes the true meaning of the term—emergency flap [50]. In a new classification, Ninkovic proposes calling flaps performed within 12–24 h after initial debridement “primary flaps.” Flaps done between 2 and 7 days after debridement are termed “delayed primary flaps,” and all other flaps performed after 7 days are “secondary flaps” [51].
The proponents of primary reconstruction demonstrated improved outcomes and less flap failures as opposed to the outcomes after secondary reconstruction. Inflammatory changes to the wound bed leads to more friable vessels and induration of periadventitial fat. This together with bacterial wound colonization or frank infection can all contribute to the adverse outcomes seen in late reconstructions. Most authors favor a delayed primary reconstruction within the first 72 h to 5 days after trauma [52]. With this approach, one can address and condition wound contamination, establish definite fixation, and optimize availability of best-qualified surgical resources.
History and Classification of Flaps
The term “flap” derived from the Dutch word “flappe”—designating a loose piece of tissue hanging on to only one side. Flaps have been reportedly used well before Christ for nasal reconstructions in India [53]. Major advances in the field have been associated with our increased anatomic knowledge of vascular supply to bone, musculature, and skin. One can generally categorize flaps based on blood supply, the tissue entity of which the flap is composed of, such as musculocutaneous or fasciocutaneous flaps, and finally the shape or location of the donor tissue—as, for example, in local V-Y advancement flap of the fingertip.
Early flaps were elevated on a random pattern of dermal blood supply and were limited in their arc of rotation and tissue advancement. The development of tubed and pedicled flaps and knowledge of revascularization of transferred tissue allowed for sequential, distal originating tissue transfers—such as groin to forearm to face transfers for reconstruction of facial defects as practiced during World War I. Detailed anatomic studies and definition of axial blood supply allowed the harvest of longer skin flaps based on a dominant vascular pedicle (e.g., groin flap). Studies by Nahai and Mathes differentiated muscle perfusion into five subtypes. This knowledge allowed for the controlled transfer of muscles based on defined pedicles. These findings significantly enhanced the development of free-tissue transfer based on reliably encountered vascular pattern pedicles [54].
While in the past the primary aim of flap coverage was defect closure and prevention of infection, in today’s practice free flap reconstruction has advanced to the point of not just covering wounds but individually addressing functional deficiencies of composite defects by restoring bony support [55] and musculotendinous function [56], including innervation and tissue coverage as in composite or chimeric flaps, which include several tissue types pedicled on a common blood vessel [57]. Increasingly restoration of function and form—meaning the aesthetic result—is seen as the ultimate goal in reconstructive surgery. Patients pleased with the aesthetic result were found to have much higher level of functionality and improved quality of life [58]. Newer developments are seen in “cut as you go” refined distal perforator flaps, prefabricated and pre-expanded flaps, as well as supermicrosurgery flaps in the below than 1 μm level [59, 60].
Reconstructive Ladder
Wound closure can be achieved by various techniques ranging from wet to dry dressing changes stimulating granulation and secondary healing as the most basic form of achieving healing all the way to complex free flaps. Most authors define a ladder of reconstruction, which increases in complexity and skills needed as you go up the rungs and potentially also leading to an increase in patient morbidity [61] (Fig. 9.4). In this concept the lowest rung of the ladder—healing by secondary intention—starts out with what is a nonsurgical wound closure and climbs up through primary, delayed primary closure to skin grafts in the middle rungs of the ladder. At this level a secondary defect is created due to tissue harvest. The benefit of achieving wound closure at the primary site must outweigh the risk associated with harvest wound problems. This said, the decision for each reconstructive option is based on defect requirements, functional demands, and donor site morbidity. The more complex flap will not necessarily yield the better result. It is the practice of most surgeons to utilize the most reliable, technically less demanding procedure as preferred choice. However, when encountering defects in functionally high demand areas, such as the dorsum of the hand, jumping up the ladder to more complex reconstructions, such as pedicled flaps or free flaps, can be necessary. When deciding on which type of flap to choose, the size of the recipient site, depth and bulk needed for reconstruction as well as anticipated pedicle length, and need for composite tissue reconstruction and options for innervation all have to be considered. The reconstructive team must either be able to offer all surgical options or refer the patient to an appropriate center. One should not choose a solution based on what is feasible for the team but what is optimal for the patient.
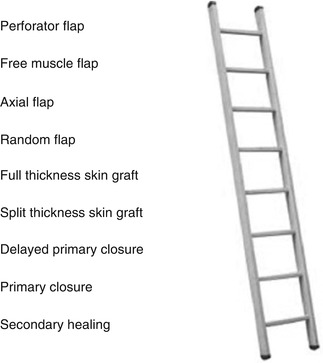
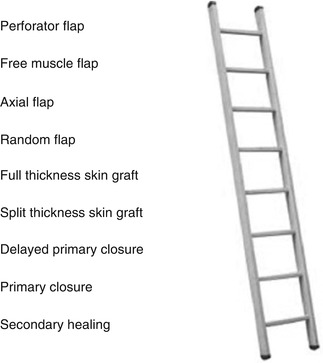
Fig. 9.4
The reconstructive ladder describing reconstructive options for soft tissue defect management. Climbing up the rungs, both technical complexity and risk of morbidity increases
Figure 9.5 shows a reconstructive algorithm.
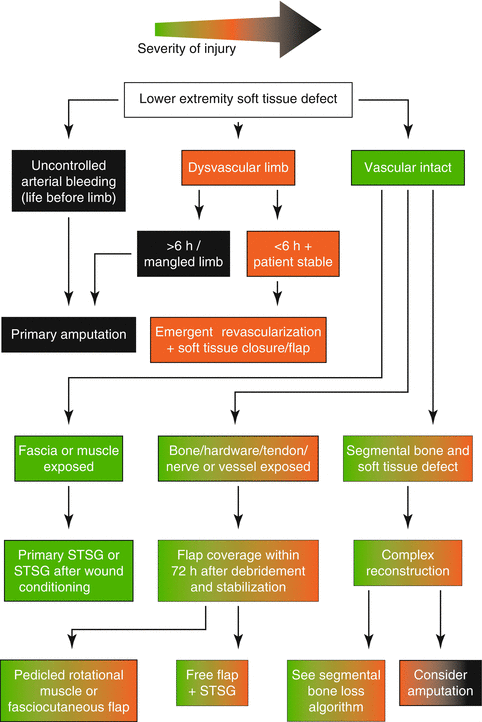
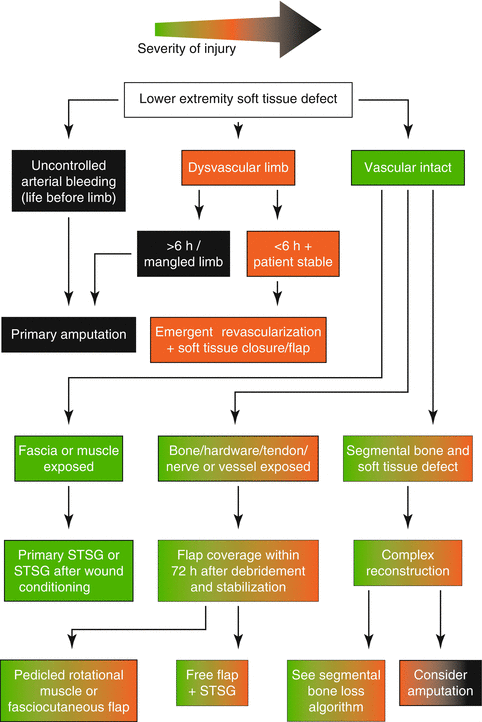
Fig. 9.5
Reconstructive algorithm for severe open lower extremity injury
Monitoring
Whenever tissue is transferred—pedicled or free—there is the risk for graft or flap loss. Successful reconstructions rely on a competent team which includes the primary surgical team as well as nursing and midlevel support staff which are often the first to detect in the postoperative course alterations to the flap condition [62]. Parallel to the reconstructive ladder, the complexity and demand in monitoring increases. Most flap failures are seen in the first 24–48 h after surgery, and during this time, patients need to be closely monitored in an intensive or intermediate care level unit which allows for hourly checks of color, turgor, temperature, and recapillarization of the flap as well as Doppler evaluation of pulse signals. A recent study showed a salvage rate of 62 % in failing flaps with higher salvage rates seen in outflow (venous) occlusions [63]. Although newer techniques such as implantable laser flow Doppler probes suggest optimization of technical flap monitoring—still clinical exam and expertise appears to the most important factor in flap monitoring [64, 65].
Complications
Soft tissue reconstruction of injured extremities must be considered a major surgery. One has to differentiate intraoperative complications from early postoperative and late complications. Intraoperative cardiopulmonary problems due to volume and blood loss must be prevented, and volume and blood component substitution must be administered judiciously. This is especially true in free flaps where adequate tissue perfusion must be maintained and vasopressors avoided. Given the extensive surgery times and often multiple operative sites, hypothermia and associated coagulopathy are feared. Technical problems must be avoided through training and planning. With regard to surgical problems and complications, surgical strategy and plan must include evaluation of donor tissue options as well available recipient vessels. The anastomosis of a free flap must be positioned well outside the zone of injury; this in turn might entail longer pedicles or the need to plan for interpositional vein grafts. One must have a backup solution available should the planned flap prove unsuited or not feasible (e.g., anomalous perforators). Proper microsurgical training is essential as well as establishing a well-trained team. There are ample possibilities for intraoperative complications, even a tight dressing compressing the flap pedicle of what was until then smooth procedure can end up in flap loss. Continuous alertness and extensive experience is mandatory. In the early postoperative course, flap loss is the most feared complication and leaves the patient with two defects and the surgeon with an even greater reconstructive problem. Undersizing the flap and underestimating the injury zone with later demarcation of dead tissue around a vital flap are feared complications. The late amputation of a “salvaged” limb due to dysfunctionality or a multitude of eventually unsuccessful surgeries to address follow-up problems such as infections and nonunions must be seen as a disastrous late complication.
Alternate Techniques
With the wide usage of wound vacuum-assisted techniques, there are many instances where deficient soft tissue coverage can be successfully managed without surgical reconstruction. This is especially true for defects with full thickness skin loss, exposing muscle but not structurally important tissues as defined above. Wound vacuum-assisted therapies can be also utilized in between debridements as a bridge to early primary flap closure.
Soft tissue defects in the extremities can be successfully closed by dynamic closure “Jacobs Ladder” type using various elastic sterile material [66]. This technique together with full thickness skin incisions as relaxation incisions—often called “pie crusting”—can obviate the need for skin grafting and/or flap coverage. In these techniques proper wound border mobilization can be helpful to achieve closure. Given the appropriate indication, these techniques can result in an aesthetic and functional superior result and higher patient satisfaction.
Lower Extremity Reconstructive Options
Thigh and Knee
Although flap reconstructions at the thigh level are uncommon given the copious amounts of well-perfused muscular tissue surrounding the femur, one can commonly see traction injuries, resulting in epifascial degloving of thigh and peripelvic tissue. These Morel-Lavallee lesions can be extensive and are for the most part located around the lateral side of the thigh and may extend high up the pelvis. The management relies on drainage and dead space management. Demarcating degloved skin needs to be excised at which point split thickness skin grafts in combination with a suction drainage or a wound vacuum-assisted device are used [67]. On rare occasions, critical soft tissue defects in the medial thigh have to be addressed such as in exposed vascular reconstructions where either local rotational flaps or free flaps may need to be employed for coverage.
Due to its exposed location and the lack of muscular coverage in the anterior aspect, traumatic soft tissue problems around the knee are common. Based on the defect size, rotational medial or lateral gastrocnemius muscle flaps are the workhorses of this body region. These flaps are pedicled on the medial and lateral sural artery respectively and can cover various soft tissue defects such as exposed patellar bone or hardware. The medial gastrocnemius has a longer muscle belly and can be easier harvested and rotated. The use of the lateral gastrocnemius is somewhat more complicated due to the peroneal nerve coursing the zone of dissection and the fibular head, which can—if the muscle is not completely transposed—shorten the rotational arc. Epimysial incisions are commonly used to increase flap length. Unilateral or bilateral gastrocnemius flaps can cover the entire anterior knee region when completely detached from their condylar insertions and skeletonized on the vascular pedicle [68]. Split thickness skin is used to epithelialize muscle flaps. The use of the tibialis anterior muscle as a proximally based rotational flap is described but seldom used due to its important role in foot dorsiflexion. As a modification the elevation of a slip of tibialis anterior for smaller defects is possible [69]. In cases of significant sized defects around the knee area, which are not amenable to rotational flap coverage—free flap reconstruction is indicated.
Leg: Proximal Third
The proximal third of the leg often requires soft tissue coverage. Defects are either due to direct trauma or seen subsequent to procedures necessitating extensile approaches to the tibial plateau. Hardware exposure is commonplace. Here similar to the already described coverage of the knee area, local rotational gastrocnemius flaps are most commonly used. The flaps can be elevated to include a strip of triceps surae tendon to allow secure flap fixation in sublay technique on the far end of the wound border. Use of suction drains in the harvest area is common practice for most surgeons. In addition to the abovementioned epimysial incisions and flap mobilization, knee flexion can increase flap length. In planning the procedure one has to position the patient such as to allow extensile dissection into the popliteal fossa.
Leg: Middle Third
The middle third of the leg is the most common area necessitating soft tissue reconstruction. The flap choice depends on defect size and injury severity. If local tissues are intact, rotational flaps can be utilized. One has to be mindful that already injured calf flexors can lose residual vitality after elevation and rotation, in which case it is more prudent to plan for a free flap. The preoperative use of angiography can be helpful to determine available recipient vessels, especially in high-energy trauma. However, one can opt for an intraoperative exploration during initial or second look debridement and perform a surgical Allen’s test—which means to clamp the recipient vessel and ascertain foot perfusion.
In going through the reconstructive ladder, simple dermal defects such as seen after full thickness necrosis of skin blisters or abrasions or fasciotomy wounds are usually successfully managed with wound debridement and split thickness skin grafting. Once a IIIA level is encountered, closure can be facilitated by delayed primary closure in a second look procedure once the swelling subsides or use of adjuvant techniques such as pie crusting the skin or dynamic skin closure. Soft tissue defects as seen in IIIB defects usually calls for elevation of a pedicled flap. This can be a muscle flap—such as the gastrocnemius and soleus flap or a fasciocutaneous flap as the sural artery neurovascular flap. The majority of low energy IIIB defects in the middle third of the leg are easily manageable by coverage with a proximal based soleus or hemisoleus flap. Some authors have described a recent increase in usage of rotational flaps and reduction in free flap reconstructions [70]. Extensile coverage can be achieved by using combined gastrocnemius-soleus flaps and skin grafting on top of the muscle flaps [71]. Once one chooses to elevate a flap, one has to be certain that the remaining soft tissues are viable and not undergoing necrosis. Oversizing the flaps to allow for secondary coverage of late demarcating injury zones and inverting the flap ends into the defect zone can be salvage options to prevent the need for additional flap coverage. If the injury severity precludes rotational flaps, one has to resort to various free flaps for coverage. Also immediate flow through flaps as emergency flaps may be indicated to restore pedal blood flow and cover the defects. A rarely used but robust reconstruction is a cross-leg flap which can salvage limbs in patients medically unsuited for extensive free flap surgery or void of recipient vessels [72]. This technique is valuable for attempts of limb salvage in underserved areas of the world. A full thickness flap is raised on the healthy contralateral leg and rotated—“cross leg” into the defect. The flap needs to be secured in place for 2–3 weeks at which point revascularization from the injured limb can occur and the flap can disconnected from the origin.
Structural vascularized bone graft such as a contralateral free fibula is an option for treating segmental defects seen after trauma or sequestrectomy [73, 74]. Vascularized free fibula flaps have a great versatility, can be harvested with attached skin islands and soleus muscle as well as the peroneal vessels as a flow through option for vascular reconstruction, and offer an option to treat segmental bone loss.
Leg: Distal Third
Whereas the proximal and middle one-third of the leg are the domains of rotational flap coverage, in the distal one-third of the leg the indication for free flaps was traditionally seen due to scarcity of tissue for transposition. This algorithm has changed with the development of axial fasciocutaneous and individual “cut as you go” perforator flaps, which allow for regional flaps to be utilized for coverage of distal leg and as well as hind- to midfoot defects [75, 76]. Tissue thickness mismatch can be a greater problem in the distal one-third of the leg where circumference, shoe fit, and esthetics are special importance. This must be a consideration when choosing a free flap. Thinner more pliable flaps such as a free radial forearm flap or free fascia flaps can be advantageous for restoring contour. One of the most commonly used flaps in the lower one-third of the leg is the sural artery flap, a fasciocutaneous flap pedicled on the sural artery. This flap can be elevated with relative ease and does not require microsurgical skills. It is usually pedicled distally and hinges on a peroneal perforator about 5 cm above the tip of the fibula [77].
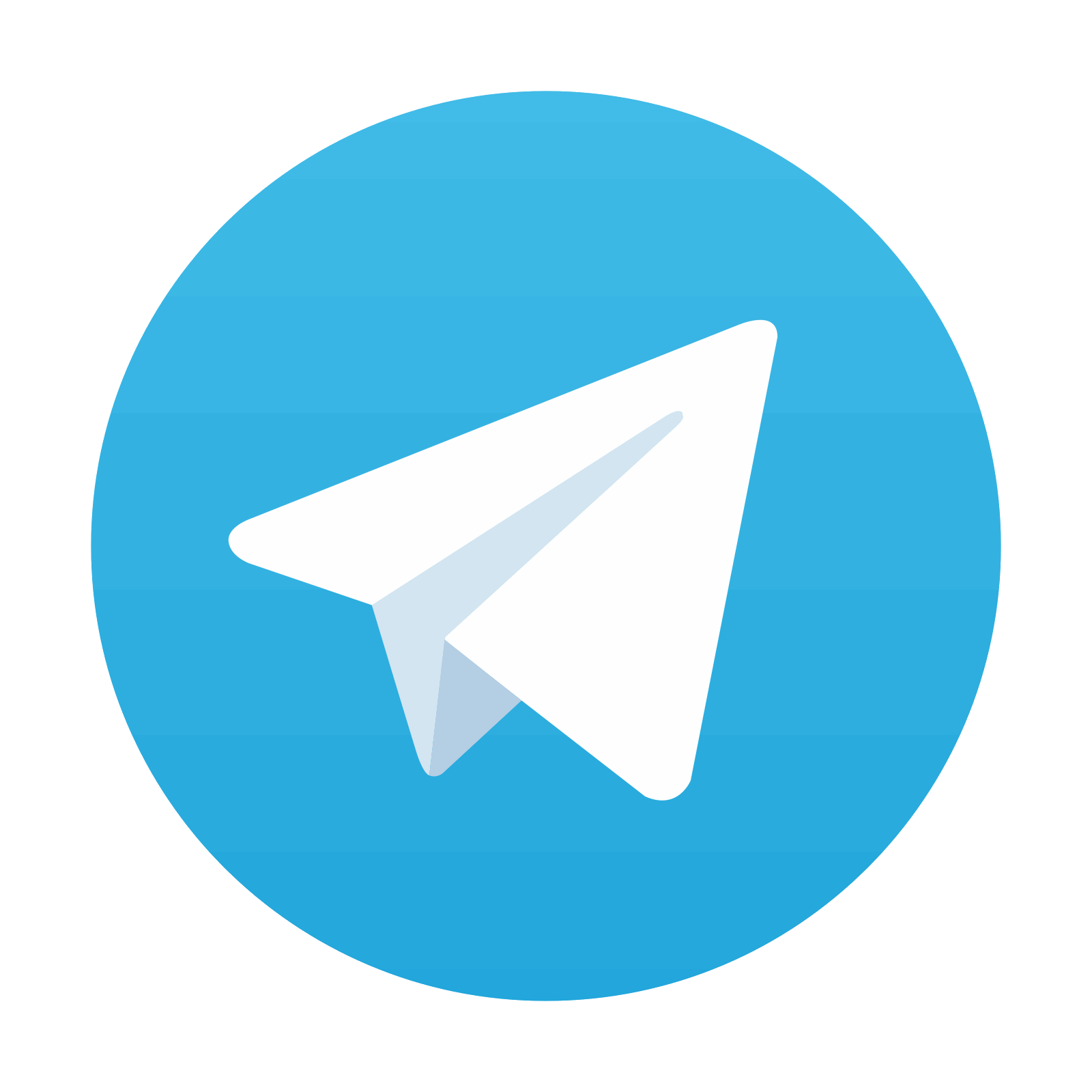
Stay updated, free articles. Join our Telegram channel

Full access? Get Clinical Tree
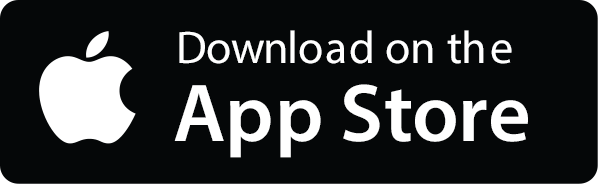
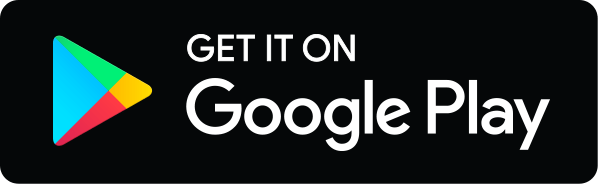