Context
What does the shoulder have to do?
Anchor point
Foot
Hip
Trunk
Release point/action point
Above shoulder
Align with shoulder
Below shoulder
Unilateral
Bilateral
Transverse rotation
No
Symmetrical
Asymmetrical
Arm(s)
Single arm
Double arm
Characteristic
High force
High rate of force development (RFD)
High endurance
High speed
Since the kinetic chain has a role in optimal function of the shoulder girdle, one must take into consideration the distal parts and their influence on local function; the scapula acts as a link between the lower limb and trunk (e.g. through the fascial connection between gluteus maximus and latissimus dorsi), the glenohumeral joint and upper limb, permitting effective transfer of forces and joint alignment [21]. Establishing a stable scapular platform is essential in minimising stresses to the shoulder during overhead movements, enabling the rotator cuff muscles to help stabilise the humeral head within the glenoid. The scapula will be influenced by the architecture and geometry of the thoracic spine, which will be influenced by the function of the lumbar spine, pelvis and lower limbs [22]. Therefore, it is essential that sub-optimal movement strategies elsewhere in the kinetic chain should be identified and included in the rehabilitation process.
Optimal demands of the kinetic chain
Joint | Requirement |
---|---|
Ankle | Mobility |
Knee | Stability |
Hip | Mobility |
Lumbar spine | Stability |
Thoracic spine | Mobility |
Scapula | Stability |
Glenohumeral | Mobility |
Screening tools for sporting shoulder
Area | Key test | Reference |
---|---|---|
Thoracic rotation | Locked lumbar rotation | Johnson and Grindstaff [24]
|
Thoracic extension | Combined elevation test | Dennis et al. [25]
|
Shoulder Internal rotation | Cools et al. [26]
| |
Shoulder External rotation | Cools et al. [26]
| |
Hip Internal/external rotation | Barbee-Ellison et al. [27]
| |
Trunk endurance | Extensor endurance test | Biering-Sorensen [28]
|
Lateral endurance test | McGill et al. [29]
| |
The flexor endurance test | McGill et al. [30]
| |
Flexion-rotation trunk test | Brotons-Gil et al. [31]
| |
Trunk muscle strength (the ability of the musculature to generate force through contractile forces and intra-abdominal pressure) | Double leg lowering test | Cutter and Kevorkian [32]
|
Trunk rate of force development | Front abdominal power test | Cowley and Swensen [33]
|
Side abdominal power test | Cowley and Swensen [33]
| |
Single leg power | Single leg counter movement jump | Hewit et al. [34] |
Single leg force capacity | Single leg mid-thigh pull/single leg isometric squat | Owens et al. [35] |
Single leg reactive strength | Single leg reactive strength index 3 hop for distance | Stalbom et al. [36] |
Single leg stability | SEBT | Gribble et al. [37] |
13.3 Thoracic Spine (T-spine)
The T-spine is comprised of 12 vertebrae, which allow flexion, extension, and rotation within those 12 segments. The ribs attach from T1 to T10 and the T-spine has thinner intervertebral discs than the lumbar spine, which adds to its relative inflexibility. T-Spine movement is described as “coupled” such that lateral binding and rotation are obligated to occur together. The T-spine essentially works as two distinctly different subgroups. The upper T-spine (T1–T5) has ipsilateral coupling of the lateral bending and rotation whereas the mid-lower T-spine (T6–T12) has contralateral coupling i.e. lateral bending and rotation occur in opposite directions [38]. Crosbie et al. [39] report that the ratio of upper to lower thoracic extension during bilateral arm elevation was 1:3, and with unilateral arm elevation ipsilateral thoracic rotation occurs. Hence the clinical assessment of the spine needs to be incorporated into management.
13.4 Rotation Range of Movement
The physical demands of sport-specific performance on an athlete’s body is responsible for specific musculoskeletal adaptation. Professional athletes are engaged for most of their sporting life in training and competition [40]. Repetitive muscular activity in the upper limb, necessary for optimal performance of overhead activities and specific movement patterns, leads to the development of sport-specific muscular adaptation in overhead players. Muscular imbalances within rotator-cuff and the peri-scapular muscles, combined with sub-optimal muscular endurance and inappropriate biomechanics can be responsible for overuse injury in the glenohumeral joint of overhead activity players [41].
Range of motion changes have also been reported in painful throwing shoulders [47–49]. Burkhart et al. [48] suggest that primary posterior inferior capsular contracture could be the potential source of the disabled throwing shoulder and that it can be measured by a glenohumeral internal rotation deficit (GIRD), and report that GIRD occurs before any other motion adaptation occurs.
Measurement of GIRD is assessed relative to the total arc of motion of the glenohumeral joint; total arc of motion is the sum of the measured glenohumeral IR + ER. It has been proposed that a healthy shoulder should present with a 180° arc of motion or, to be more functionally correct, the arc of motion should be equal bilaterally [11]. Previous researchers have documented <20° side difference for IR, and <10% side difference for total ROM as being acceptable values that are unlikely to contribute to pathology [50–54]. Predictive findings have been proposed as; loss of >25° into IR [55] in baseball and softball, and a loss of 20° IR and a loss of 5% in total ROM doubles the risk for injury in professional baseball pitchers. Although Clarsen et al. [56] were unable to find any associations between glenohumeral internal rotation deficits, external rotation deficits or total range of movement differences and injury.
13.5 Shoulder Strength
One of the contributing factors to shoulder injury, and detected on clinical assessment of symptomatic patients is reduction in shoulder strength around the rotator cuff and scapular muscles. The existence of an imbalance between the agonist and antagonist muscle groups has been shown to be one of the major risk factors for developing shoulder injuries [57], with a reduction in the external rotator strength conceivably causing an injury [58].
Controversy exists in the literature as to whether absolute strength or the IR:ER strength ratio should be utilised to quantify the ideal levels of dynamic shoulder stability, particularly in overhead athletes [59]. Several researchers [11, 46–48, 60] have advocated that the combination of forceful, eccentric contractions coupled with high distraction forces may cause microtrauma to the external rotators and posterior cuff during the follow through/deceleration that will re-model in accordance with Wolff’s Law, which states that tissues will adapt to the stresses placed on them [61].
Many methods of assessing the strength around the shoulder girdle have been used; isokinetic dynamometers [62, 63], weight lifting [64], manual muscle testing (MMT) [65, 66], and hand held dynamometers (HHD) [26, 65].
Isokinetic dynamometers have been used as a clinical measure of muscle strength and endurance of the rotator cuff and the scapular stabilisers [26] and have the capability to measure strength at different speeds [67, 68], but these dynamometers are not readily available and the clinical validity of the results can be brought into question.
MMT has good clinically utility but is highly subject to user error and bias [65, 69] and it is difficult to assess small changes in muscle strength and present objective data utilising this method [65]. The results can be influenced by the experience and strength of the examiner [70, 71].
HHD is a more objective method of evaluation and is far superior to MMT when evaluating changes in muscle strength caused by dysfunction [72]. Numerous studies have reported the reliability of HHD to assess upper limb muscle strength including scapular muscles [65, 73, 74].
13.6 Methods of Testing
Outcome measures and return to play decision
Outcome measurements and return to play decision | Scapulothoracic joint [51] | |
GH IR and ER ROM | Scapular upward rotation | |
GH rotator cuff strength | Strength of the scapular stabilisers | |
Eccentric strength of the external rotators | PM length/PM index
|
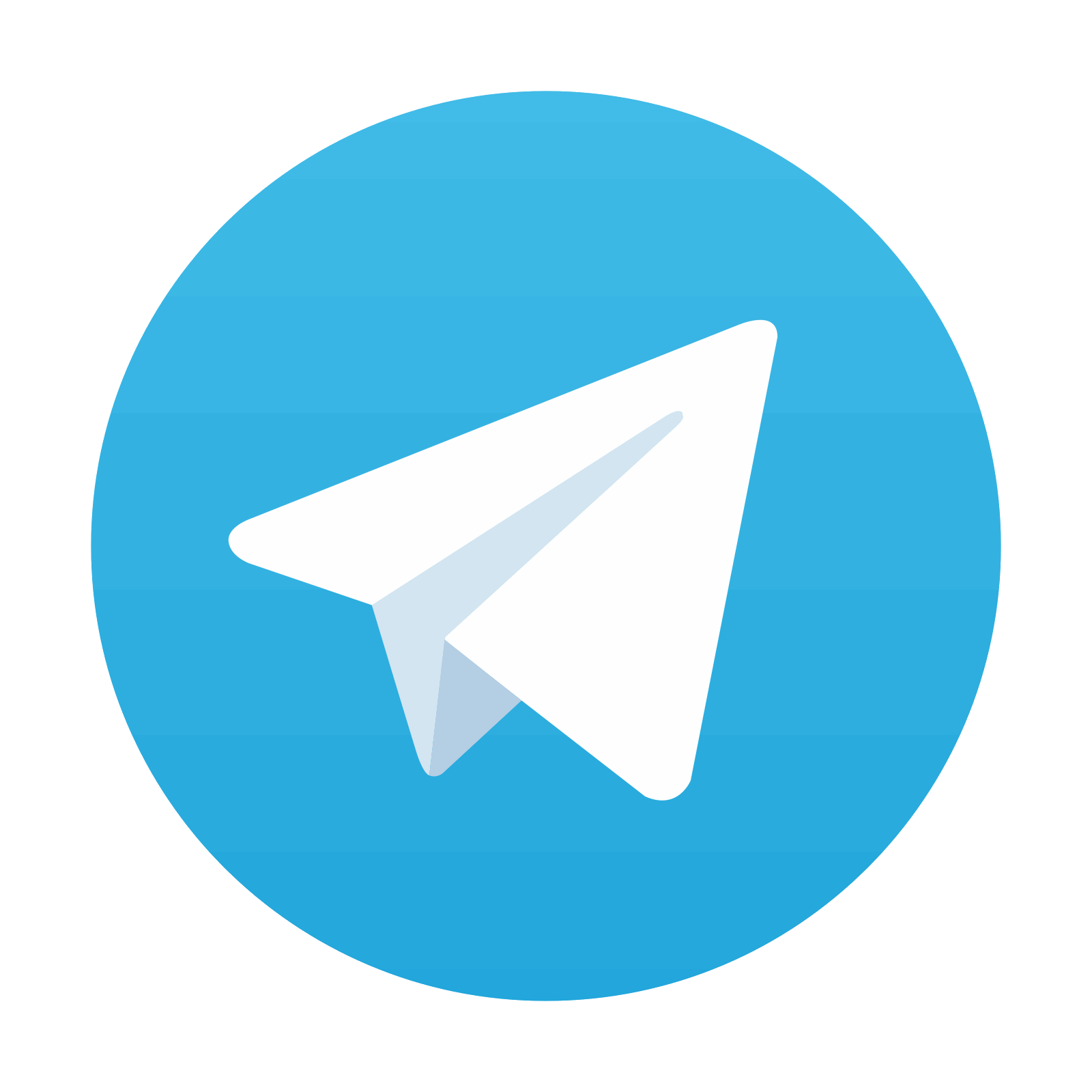
Stay updated, free articles. Join our Telegram channel

Full access? Get Clinical Tree
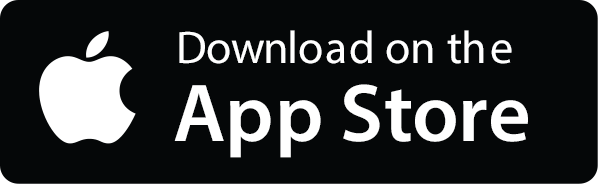
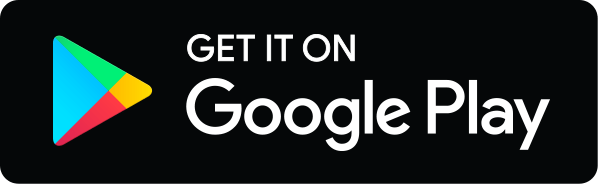