Nutritional Support of the Injured
Ernest F.J. Block
The important role of nutritional support in surgical patients is widely recognized. It is now becoming increasingly clear that this role goes far beyond simply providing the patient with adequate amounts of protein and calories. One must not only be concerned with the calories supplied but must also take into consideration the timing, route of administration, and individual components of a nutritional regimen. The gastrointestinal (GI) tract is the largest immune organ in the body and contains 65% of the body’s overall immune tissue and up to 80% of the immunoglobulin producing cells. It is a rich source of lymphocytes, plasma cells, and macrophages and has a pivotal role in modulating the patients’ response to injury. Providing the GI tract with nutrients directly in the form of enteral nutrition can help prevent mucosal atrophy and increased permeability with resultant bacterial translocation and priming of neutrophils and macrophages. Early aggressive nutritional support within 12 to 24 hours of injury is important to decrease the incidence of the systemic inflammatory response syndrome and organ dysfunction. The goals of nutritional support have spread beyond providing the injured host with energy to actually modifying an individual’s response to the insult at the cellular and biochemical level. A basic understanding of these biochemical principles will help the surgeon better understand the rationale behind the evidence practiced.
ENDOCRINE AND METABOLIC RESPONSE TO TRAUMA
Acutely traumatized patients have a well-characterized orchestrated response to injury, which eventually leads to the activation of the hypothalamus-pituitary-adrenal axis, the renin-angiotensin system, and the autonomic nervous system. The end result is generalized catabolism leading to protein wasting, negative nitrogen balance, and erosion of lean body mass and circulating proteins such as albumin and prealbumin.
Initial activation is multifactorial and is the result of mediators produced both systemically and locally at the site of injury. The inciting events are intravascular and extracellular fluid volume losses, tissue hypoperfusion, tissue damage, pain, fear, and emotion. Tissue damage, either as a direct result of trauma or secondary to hypoperfusion or infection, is perhaps the most potent stimulus. Changes in pH, partial pressures of oxygen and carbon dioxide, temperature, and blood pressure are detected through various central and peripheral receptors, which ultimately lead to the activation of these biochemical pathways. The mediators that regulate one’s response to injury and sepsis have been well characterized. With acute injury, we see a rapid rise in the serum concentrations of adrenocorticotropin (ACTH), cortisol, epinephrine, norepinephrine, glucagon, vasopressin (antidiuretic hormone), and aldosterone. Various proinflammatory cytokines, primarily tumor necrosis factor and interleukin 1, are also elevated.
Hepatic glycogen is rapidly utilized and the goal is to provide substrates for hepatic gluconeogenesis to support the massive energy needs of acute injury. The counter-regulatory hormones previously mentioned lead to proteolysis and amino acid production as well as lipolysis with the generation of glycerol and free fatty acids. Amino acids (namely, glutamine and alanine), lactate, and glycerol serve as carbon skeletons for the hepatic production of glucose for use by erythrocytes, leukocytes, and cells of the central nervous system that do not require
insulin for glucose transport and utilization. Release of these substances also leads to decreased levels of insulin produced from the pancreas as well as a state of peripheral insulin resistance and hyperglycemia. Insulin is normally a dominant anabolic stimulus and its absence in times of injury and stress is not without consequence. Normally, insulin stimulates amino acid transport into muscle. Low levels of insulin stimulate protein degradation, adding to the state of negative nitrogen balance and catabolism. In contrast to starvation, suppression of proteolysis does not occur and gluconeogenesis continues at an accelerated rate at the expense of lean body mass (mainly skeletal muscle). Low levels of insulin further promote lipolysis and the production of free fatty acids, which will also serve as an important fuel source to support hypermetabolism. Early and aggressive nutritional therapy is necessary to minimize the insults put forth by the neurohumoral axis.
insulin for glucose transport and utilization. Release of these substances also leads to decreased levels of insulin produced from the pancreas as well as a state of peripheral insulin resistance and hyperglycemia. Insulin is normally a dominant anabolic stimulus and its absence in times of injury and stress is not without consequence. Normally, insulin stimulates amino acid transport into muscle. Low levels of insulin stimulate protein degradation, adding to the state of negative nitrogen balance and catabolism. In contrast to starvation, suppression of proteolysis does not occur and gluconeogenesis continues at an accelerated rate at the expense of lean body mass (mainly skeletal muscle). Low levels of insulin further promote lipolysis and the production of free fatty acids, which will also serve as an important fuel source to support hypermetabolism. Early and aggressive nutritional therapy is necessary to minimize the insults put forth by the neurohumoral axis.
In the absence of preexisting nutritional defects, the goal of nutritional support in the critically ill patient is to support the hypermetabolic state and preserve as much lean body mass as possible. It is important to realize that nutritional support is an adjunct to the overall care of these critically injured patients. The underlying disease process is the cause of the malnutrition. One cannot expect to convert a hypermetabolic, catabolic individual to a state of positive nitrogen balance with nutrition alone. It is impossible to completely halt amino acid degradation, and some degree of muscle wasting is inevitable. Depending on the severity of the illness, it may take weeks to months to achieve anabolism and one must be patient enough to avoid the complications associated with overfeeding. It is equally important to concentrate on the initial cause of the hypermetabolic illness by eliminating ongoing blood loss, sources of sepsis, and other secondary insults that can prolong the catabolic state.
FORMULATION AND COMPOSITION
Caloric Requirements
The fundamental goal in nutritional supplementation is to meet the energy requirements for metabolic processes, to support hypermetabolism, and to minimize protein catabolism. Nutritional support begins with an estimation of the patient’s caloric requirements. The Harris Benedict equation is one of the most commonly used methods for estimating caloric needs or basal energy expenditure (BEE):
Males: BEE = 66.5 + 13.8(weight in kg) +5 (height in cm) − 6.8 (age)
Females: BEE = 65.5 + 9.6(weight in kg) +1.7 (height in cm) − 4.7 (age)
(Stress factors: minor surgery 1.2, trauma 1.35, sepsis 1.6, major burns 2.1)
These equations yield basal energy requirements that are frequently multiplied by various activity and/or stress factors to generate a resting energy expenditure (REE), although this may lead to an overestimation of the patient’s actual caloric needs which could lead to unnecessary administration of nutrients and overfeeding. Another important consideration is the patient’s weight that is used for these calculations. One must avoid using the patient’s actual body weight (ABW), which could be quite elevated after a period of aggressive volume resuscitation. Ideally an admission weight before interventions should be obtained. In practice, this assessment is impractical and not normally part of the primary and secondary surveys in the acutely injured patient. An accurate measurement of body weight (BW) is an arduous task in critically ill patients with bulky dressings, catheters, monitoring wires, tubes, and drains. The ideal body weight (IBW) could prove useful in such circumstances and is calculated as follows:
IBW = 2.3 (height in inches over ft) + (45.5 [females] or 50 [males])
Obesity poses another scenario where overfeeding may result if one uses the patient’s ABW. In such circumstances (Obesity = ABW 20% over IBW or a body mass index [BMI] >30), we typically use the adjusted BW for energy calculations and requirements:
Adjusted BW = (ABW − IBW) 0.25 + IBW
A more specific method frequently used to determine caloric needs in ventilated patients is to perform indirect calorimetry. A metabolic cart is used to collect expired gases to determine CO2 production and O2 consumption which are used to calculate the REE using the Weir equation:
REE = (Vo2[3.941] + VcO2 [1.11]) 1,440 minutes/day
Indirect calorimetry also provides the respiratory quotient (RQ) (RQ = CO2 production divided by O2 consumption), which can be further used to monitor the adequacy of nutritional support. An RQ of >1 suggests overfeeding and lipogenesis. An RQ of 1 indicates pure carbohydrate utilization. Unlike glucose metabolism, oxidation of fatty acids requires less oxygen and produces less carbon dioxide. Pure fat utilization produces an RQ of 7 and a value of <7 suggests underfeeding and ketogenesis. Mixed substrate utilization is suggested when the RQ value falls within the 0.8 and 0.9 range.
There are several limitations to indirect calorimetry including the need for dedicated equipment and staff. Such measurements are costly and are for the most part limited to those patients on the ventilator. Measurements may be inaccurate in patients with conditions often encountered in the critically ill. These include patients on high levels of oxygen (FIO2 >0.6), high positive-end expiratory pressure,
air leaks that may limit the ability to adequately collect and analyze expired gases, and peritoneal and hemodialysis due to removal across the membrane of CO2 that is not measured by the indirect calorimeter. One must also consider the fact that these measurements are only a reflection of the short window of time that the patient is being studied. In general, the patient selected for this study should be in a stable, steady state. The patient should be protected from noise or other stimulation as permitted.
air leaks that may limit the ability to adequately collect and analyze expired gases, and peritoneal and hemodialysis due to removal across the membrane of CO2 that is not measured by the indirect calorimeter. One must also consider the fact that these measurements are only a reflection of the short window of time that the patient is being studied. In general, the patient selected for this study should be in a stable, steady state. The patient should be protected from noise or other stimulation as permitted.
Despite these limitations, indirect calorimetry serves several roles. Underestimation of the stress factor in the formulas given in the preceding text could lead to inadequate caloric delivery which may be detected. Equally troubling would be overfeeding; the metabolic cart should be considered a diagnostic study in patients who fail attempts at liberation from mechanical ventilation to measure the O2 cost of breathing and the components of or to determine the cause of increased ventilatory requirements.
Specific literature addressing the optimal method for nutritional assessment is lacking. It should be remembered that no specific formula has been proved to be superior to the others and these should be considered to provide at best an estimate of the patient’s initial protein and caloric needs. Currently, recommendations for most surgical patients are to provide approximately 25 to 30 kcal/kg/day through the administration of carbohydrates (70%) and lipids (30%). Protein should not be included in these energy calculations. Oxidation of 1 g of fat yields approximately 9 kcal of energy and 1 g of carbohydrate yields approximately 4 kcal of energy. It should be noted, however, that those sugars found in enteral formulas and total parenteral nutrition (TPN) typically yield 3.4 kcal per g. We typically start out with 30 kcal/kg/day based on the patient’s admission weight, if available. As previously mentioned, in obese individuals we will use the adjusted ABW.
TABLE 1 ENTERAL FORMULATIONS | ||||||||||||||||||||||||||||||||||||||||||||||||||||||||||||||||||||||||||||||||||||
---|---|---|---|---|---|---|---|---|---|---|---|---|---|---|---|---|---|---|---|---|---|---|---|---|---|---|---|---|---|---|---|---|---|---|---|---|---|---|---|---|---|---|---|---|---|---|---|---|---|---|---|---|---|---|---|---|---|---|---|---|---|---|---|---|---|---|---|---|---|---|---|---|---|---|---|---|---|---|---|---|---|---|---|---|
|
Carbohydrate and Lipid Requirements
The exogenous administration of glucose (50 to 100 g per day) favors fat entry into the TCA cycle and avoids the formation of ketone bodies, and in the absence of severe illness limits amino acid degradation and urinary urea production. In the absence of severe hyperglycemia or brain injury, it is therefore important to consider adding dextrose to the patient’s maintenance intravenous fluids. Once formal nutritional support is begun, the exact percentage of the caloric source is predetermined by the enteral formulation one chooses (see Table 1). If one is receiving TPN, the amount of carbohydrate infusion should not exceed the maximum rate of glucose utilization, which is approximately 5 mg/kg/minute. This is rarely a problem when adequate amounts of lipids are given as an additional caloric source.
Protein Requirements
Protein is the building block of life. Once hepatic glycogen stores are depleted, muscle protein is degraded to provide three carbon backbones for hepatic gluconeogenesis. Initially protein catabolism is resistant to the administration of exogenous amino acids and it takes weeks until a critically ill patient is found to be in a state of positive nitrogen balance. In addition to protein catabolism, exogenous protein is required for wound healing and to replace that lost in wounds and fistulae. The goal is to minimize the loss of lean body mass and as a general rule this requires anywhere from 1.0 to 1.5 g/kg/day of protein depending on the degree of illness and injury. A severely burned patient may require in excess of 2.0 g/kg/day to replace the large quantities of protein lost through their wounds. Another circumstance that one must consider is the patient with an open abdomen who may lose additional amounts of nitrogen through the temporary dressing.
There are several ways to estimate protein requirements. One simple method is to look at the ratio of nonprotein calories to grams of nitrogen. A typical postoperative patient requires a ratio of 150 carbohydrate calories to 1 g of nitrogen to prevent the use of protein as an energy source. Critically ill subjects and burn victims may require values <100. Another method is to calculate the nitrogen balance following collection of a 24-hour urine specimen:
|
where UUN is urine urea nitrogen in grams of nitrogen excreted in the urine over the 24-hour period. Four grams is typically used to estimate insensible nitrogenous losses through the skin and GI tract. This is a very crude estimate of nitrogen balance and may not be valid in the patient with large burns or wounds. Cheatham et al. showed that patients with an open abdomen lose on average 1.9 g nitrogen per L of abdominal fluid. In patients with temporary abdominal dressings, this needs to be added to the insensible losses to avoid overestimating nitrogen balance, which would in turn lead to underfeeding and inadequate nutritional support.1 A patient in positive nitrogen balance excretes less nitrogen than is being consumed and is incorporating nitrogen into newly formed protein. A patient in negative nitrogen balance excretes more nitrogen than they consume and continues to degrade muscle protein for gluconeogenic precursors.
Overfeeding
It is often difficult to estimate a patient’s energy requirements and excess caloric administration can lead to overfeeding. The importance of estimating one’s premorbid dry weight was previously discussed. The Harris Benedict equation and indirect calorimetry can both overestimate caloric needs. An RQ >1.0 suggests overfeeding. Excess carbohydrate administration can lead to lipogenesis, hepatic steatosis, glycogen deposition, hypercarbia, and respiratory insufficiency. Although usually not a problem in the typical young, previously healthy trauma victim, problems may occur in patients with chronic obstructive pulmonary disorder (COPD) and preexisting pulmonary insufficiency. Excess carbohydrate intake also results in hyperglycemia, which can lead to immunosuppression and leukocyte dysfunction, increased infectious complications, glycosuria, and dehydration. Choosing a formulation with a greater proportion of lipids may be necessary to avoid these complications. Lipid overfeeding is not benign, however, and may be associated with hyperlipidemia, cholestasis, increased risk of infection, immunosuppression, and inhibition of the reticuloendothelial system. In patients receiving intravenous lipids, one must periodically check serum triglyceride levels and withhold further infusions when the concentrations rise above 400 to 500 mg per dL.
Special Circumstances
Renal Failure
The goal of feeding patients with renal insufficiency is to prevent further accumulation of nitrogenous wastes, volume, electrolytes, and minerals. Many critically ill patients exhibit varying degrees of renal impairment. In the patient with renal failure and a worsening blood urea nitrogen concentration, it is important to avoid the overzealous administration of protein. This is less of a concern in dialyzed patients and those on continuous forms of dialysis may even require more protein due to the considerable losses in the dialysate. Many different formulas exist for the patient with worsening renal function or acute renal failure. These formulations have lower concentrations of potassium and phosphate and a higher ratio of nonprotein calories to gram of nitrogen. These products are also more concentrated, hyperosmolar, and calorically rich. Adequate collaboration between the nephrologist and the surgical nutritionist should accomplish these goals with intensive dialysis and careful metabolic monitoring and supplementation.
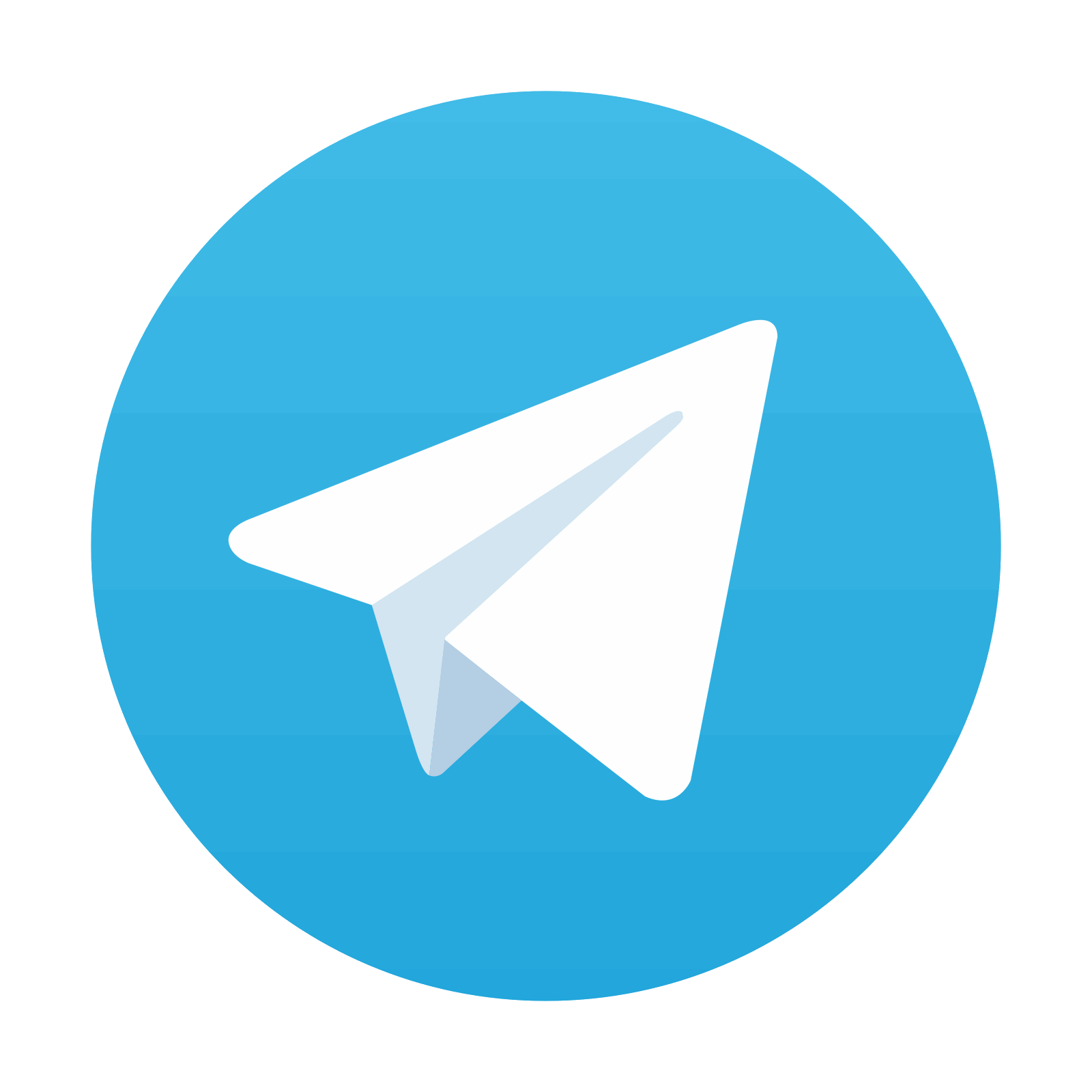
Stay updated, free articles. Join our Telegram channel

Full access? Get Clinical Tree
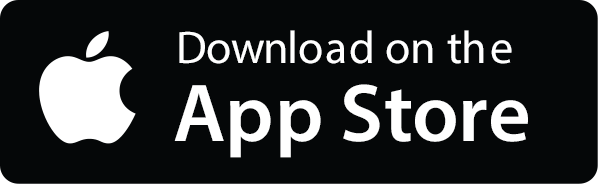
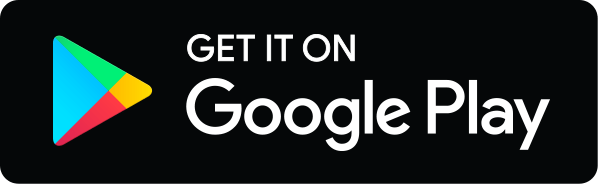
