Low GI
Moderate GI
High GI
Food
GI
Food
GI
Food
GI
Chana dal
8
Apple juice
40
Life Savers™
70
Peanuts
14
Snickers™
41
White bread
70
Plain yogurt
14
Peach
42
Bagel
72
Soy beans
18
Pudding
43
Watermelon
72
Rice bran
19
Pinto beans
45
Graham crackers
74
Peas
22
Orange juice
46
French fries
75
Cherries
22
Baked beans
48
Total™
76
Barley
25
Strawberry jam
51
Vanilla wafers
77
Grapefruit
25
Sweat potato
54
Gatorade™
78
Kidney beans
27
Pound cake
54
Fava beans
79
Link sausages
28
Popcorn
55
Jelly beans
80
Black beans
30
Brown rice
55
Tapioca pudding
81
Lentils
30
Fruit cocktail
55
Rice cakes
82
Butter beans
31
Pita bread
57
Team Flakes™
82
Soy milk
31
PowerBar™
58
Pretzels
83
Lima beans
32
Honey
58
Corn Chex™
83
Skim milk
32
Blueberry muffin
59
Corn flakes™
84
Split peas
32
Shredded wheat
62
Baked white potato
85
Fettucini
32
Black bean soup
64
Mashed potatoes
86
Chickpeas
33
Macaroni and cheese
64
Dark rye
86
Peanut M&M’s™
33
Raisins
64
Instant rice
87
Chocolate milk
34
Canteloupe
65
Crispix™
87
Vermicelli
35
Mars Bar™
65
Boiled Sebago
87
Whole wheat spag.
37
Rye bread
65
Rice Chex™
89
Apple
38
Pineapple
66
Gluten-free bread
90
Pear
38
Grapenuts™
67
Baked red potato
93
Tomato soup
38
Angel food cake
67
French baguette
95
Ravioli
39
Stoned wheat thins
67
Peeled Desiree
101
Pinto beans
39
Taco shells
68
Dates
103
Plums
39
Whole wheat bread
69
Tofu frozen dessert
115
Sidebar
The glycemic index is a ranking of foods based on their postprandial blood glucose response compared to a reference food, either glucose or white bread. The glycemic index concept was first developed in 1981 to help determine which foods were best for people with diabetes. The glycemic index of a food is based on several factors, including the physical form of the food, the amylose/amylopectin ratio (two types of starch), sugar content, fiber content, fat content, and the acidity of a food. The index consists of a scale from 0 to 100 with 0 (water) representing the lowest ranking and 100 (pure glucose) the highest ranking. The glycemic index is obtained through use of an oral glucose tolerance test utilizing 50 g of carbohydrate from the test food. Blood samples are obtained periodically throughout a 2-h period, glucose levels are measured, and the area under the curve is calculated.
The glycemic index of a carbohydrate has a profound effect on subsequent glucose and insulin responses. High-glycemic index carbohydrates (i.e., dextrose, maltose) produce large increases in glucose and insulin levels. Moderate-glycemic index carbohydrates (i.e., sucrose, lactose) traditionally produce only modest increases in glucose and insulin. Finally, low-glycemic index carbohydrates (i.e., fructose, maltodextrin) have little if any effect on glucose and insulin responses. It has been suggested that manipulating the glycemic index of a sports supplement may optimize carbohydrate availability for exercise, particularly prolonged intense exercise. Caution should be used when applying the glycemic index to whole foods that contain several ingredients.
Nutrition during an intense endurance training session can also aid workout quality as participation time exceeds 60–90 min and usually centers on supplementation more than pre- and post-exercise nutrition if for no other reason than the convenience supplements provide. Convenience supplements include glucose–electrolyte solutions, meal replacement powders, ready-to-drink supplements, energy bars, energy gels, and fitness waters. They are typically fortified with various amounts of vitamins and minerals and differ on the amount of carbohydrate, protein, and fat they contain. The beneficial effects of solid and liquid carbohydrate/protein supplements are similar when thermal stress is not a factor. Liquid supplements also aid rehydration and tend to digest easier for most athletes while exercising.
Cyclists can generally empty from the stomach up to 1,000 ml of fluid per hour, and therefore 40–60 g of carbohydrate can be easily ingested while consuming a large volume of fluid [14]. On the other hand, runners generally consume less than 500 ml of fluid per hour due to the [15]. Therefore, runners tend to use more concentrated solutions than cyclists in order to consume adequate amounts of carbohydrate. For cyclists, a carbohydrate solution of 4–6 % is generally sufficient when fluid replacement is important. For runners, this concentration may have to be 8–10 % to provide adequate carbohydrate. Glucose concentrations in excess of 10 % seem to delay gastric emptying and compromise fluid replacement (Fig. 11.1).
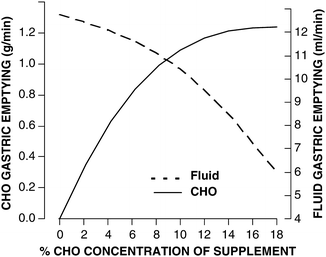
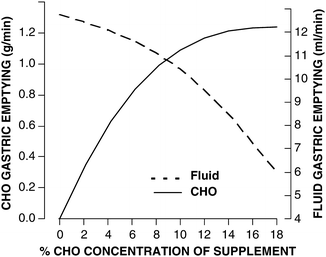
Fig. 11.1
Effect of carbohydrate (CHO) concentration on the rate of gastric emptying of fluids and carbohydrate from the stomach during exercise. Carbohydrate concentrations of 8–10 % maximize carbohydrate gastric emptying without substantially reducing fluid delivery. From Gisolfi and Lamb [16], with permission
Adequate sodium intake is also important to combat potential electrolyte imbalances during exercise. Most GES solutions contain sufficient quantities of sodium. If sodium is lacking in the supplement, however, electrolyte tablets are also available. The bottom line is that rapid nutrient availability is especially important during a workout to maintain energy levels and training intensity. Thus, high-glycemic index sources should make up most of the supplements ingested during an endurance-type workout. Once again, athletes should experiment with different formulations to find the one that works best for them prior to competition.
It is now well established that with prolonged continuous exercise the time-to-fatigue at moderate sub-maximum exercise intensities is related to pre-exercise muscle glycogen concentrations—thus the importance of everyday nutrition along with pre-exercise nutrition [17]. In addition, a GES has been the recommended supplement of choice for decades during exercise to preserve muscle glycogen and maintain blood glucose levels. With short-term, high-intensity exercise, the relation between the availability of muscle glycogen and performance is less clear. One study that utilized 15 high-intensity 6-second bouts on a cycle ergometer concluded that a high carbohydrate regimen over 48 h helped subjects maintain a higher power output than did the exercise and dietary regimen that included a low carbohydrate content [18]. This study demonstrated the importance of a high carbohydrate diet in relation to short-term, high-intensity exercise.
Recent research has shown that the addition of protein can have added benefits to a supplement ingested during exercise by reducing muscle protein degradation and speeding post-exercise recovery. Carbohydrate and protein intake significantly alters circulating metabolites and the hormonal milieu (i.e., insulin, testosterone, growth hormone, cortisol) as well as the response of muscle protein and glycogen balance [19]. Furthermore, the addition of protein to a carbohydrate supplement enhances the insulin response of a carbohydrate supplement compared to a placebo [13, 20], which can ultimately lead to performance gains [19].
Saunders et al. examined the effects of a carbohydrate–protein beverage on cycling endurance and muscle damage [21]. They utilized 15 male cyclists who were randomly administered either a carbohydrate or carbohydrate–protein beverage (4:1 ratio) every 15 min during exercise and immediately upon completion of a ride to volitional exhaustion. The carbohydrate–protein beverage produced significant improvement in time-to-fatigue and reduction of muscle damage in the selected endurance athletes. The authors concluded that the benefits observed were the result of a higher total caloric content of the carbohydrate–protein beverage or were due to specific protein-mediated mechanisms.
Controversy exists among numerous studies examining the addition of protein because such additions increase the total caloric content of the supplement. Anytime a larger amount of calories is consumed, an athlete is likely to perform and recover more rapidly. Therefore, when examining studies that are not based on isocaloric data, one should give them careful consideration.
Whey is the preferred protein to ingest during exercise because of its rapid absorption rates and the fact that is contains all of the essential amino acids as well as a high percentage of leucine and glutamine, which are amino acids the body uses during sustained exercise [22]. High-glycemic index carbohydrates (glucose, sucrose, maltodextrin) should be combined with the protein in a 4:1 ratio to provide optimal benefits. Table 11.2 gives an example of the ideal nutrient composition for a sports drink during exercise [24]. Sports drinks such as that shown in Table 11.2 should be ingested every 20 min during an endurance training session to help improve performance and reduce muscle protein breakdown.
Table 11.2
Ideal nutrient composition for a sports drink during exercise
Nutrient objectives |
Replace fluids and electrolytes |
Preserve muscle glycogen |
Maintain blood glucose levels |
Maintain hydration |
Minimize cortisol increases |
Set the stage for a faster recovery |
Satisfy thirst |
Ideal composition (per 12 oz water) |
High-glycemic carbohydrates (e.g., glucose, sucrose, maltodextrin): 20–26 g |
Whey protein: 5–6 g |
Vitamin C: 30–120 g |
Vitamin E: 20–60 IU |
Sodium: 100–250 mg |
Potassium: 60–120 mg |
Magnesium: 60–120 mg |
Post-exercise nutrition for the endurance athlete is vital to restore muscle glycogen stores, enhance skeletal muscle fiber repair and growth, and maintain overall health and wellness. After an intense exercise bout, the body is in a catabolic state (thus key muscle nutrients are being broken down). However, the opportunity exists to alter the catabolic state into a more anabolic hormonal profile where the athlete begins to rebuild muscle and thus initiates a much faster recovery. Exercise that results in glycogen depletion activates glycogen synthase, the enzyme responsible for controlling the transfer of glucose from UDP-glucose to an amylase chain [24, 25]. This also happens to be the rate-limiting step of glycogen formation.
The degree of glycogen synthase activation is influenced by the extent of glycogen depletion [24]. Complete resynthesis of muscle glycogen, however, ultimately depends on adequate carbohydrate intake. Carbohydrates composed of glucose or glucose polymers are the most effective for replenishing muscle glycogen, whereas fructose is most beneficial for replenishing liver glycogen [26]. Glucose and fructose are metabolized differently. They have different gastric emptying rates and are absorbed into the blood at different rates [25, 27]. Furthermore, the insulin response to a glucose supplement is generally much greater than that of a fructose supplement [18]. The fact that approximately 79 and 14 % of total carbohydrate is stored in skeletal muscle and the liver, respectively, is further indication of the importance of consuming glucose or glucose polymers after exercise [9].
Blom et al. found that ingestion of glucose and sucrose was twice as effective as fructose for restoring muscle glycogen [28]. The maximum stimulatory effect of oral glucose intake on post-exercise muscle glycogen synthesis was reached at a dose of 0.70 g/kg taken every second hour following exercise in which the muscle glycogen concentration was reduced by an average of 80 %. In addition, the rate of post-exercise muscle glycogen synthesis increases with increasing oral glucose intake, up to a maximum rate of approximately 6 mmol/kg/h. Blom et al. indicated that the differences between glucose and fructose supplementation were the result of the way the body metabolized these sugars [28]. Fructose metabolism takes place predominantly in the liver, whereas most glucose appears to bypass the liver and is stored or oxidized by muscle [26].
Subsequent research by Burke and associates found that the intake of high-glycemic index carbohydrate foods after prolonged exercise produces significantly more glycogen storage than consumption of low-glycemic index carbohydrate foods 24 h after exercise [29]. Although the meal immediately after exercise elicited exaggerated blood glucose and plasma insulin responses that were similar for the low-glycemic index and high-glycemic index meals, for the remainder of the 24 h the low-glycemic index meals elicited lower glucose and insulin responses than the high-glycemic index meals. As previously mentioned, protein has shown additive effects to that of carbohydrate alone in regard to the rate of muscle glycogen resynthesis and overall post-exercise recovery. The addition of protein to a carbohydrate supplement increases insulin levels more than that produced by carbohydrate or protein alone [30]. Tarnopolsky et al. showed that post-exercise carbohydrate and carbohydrate–protein–fat nutritional supplements can increase glycogen resynthesis during the first 4 h after exercise to a greater extent than placebo for both men and women [31]. The supplements administered were both isoenergetic and isonitrogenous. Insulin has a profound anabolic effect on skeletal muscle. In the resting state, insulin decreases the rate of muscle protein degradation [32].
More recent research suggests that carbohydrate taking the form of a long chain glucose polymer (LCGP) results in faster gastric emptying versus a moderately hypertonic carbohydrate source [33]. Follow-up studies using the same compound also show a faster rate of glycogen synthesis 2 h following ingestion suggesting that LCGP may optimize glycogen replacement following depletion [34]. During exercise, however, two studies by Rowland and colleagues show no improvement in carbohydrate oxidation accompanying LCGP ingestion during exercise [35, 36]. Therefore, the potential ergogenic effects of LGCO during exercise remain questionable without further research. One interesting finding presented by Stephens et al. (2008) does suggest that LCGP may improve exercise responses when training or competition is broken up. In this study, participants consumed a (a) control beverage, (b) a 100 g of a low molecular weight glucose polymer, or (c) 100 g of a LCGP immediately following 90 min of cycling at ~73 % of VO2max. After 2 h of rest, they then performed a 15-min time trial. The results of the time trial showed that participants consuming the LCGP were able to perform more work versus the control or low molecular weight glucose polymer solutions. These latter results suggest that LCGP may be a useful supplement strategy during training or competition requiring heavy exertion during several points within the same day.
To summarize, research has clearly shown that muscle glycogen resynthesis occurs more quickly if carbohydrate is consumed immediately following exercise in contrast to waiting for several hours [37]. Whereas most of the everyday diet for the endurance athlete should be a low- to moderate-glycemic index diet, the post-exercise diet should be centered on moderate- to high-glycemic index sources. This nutritional approach accelerates glycogen resynthesis and promotes a more anabolic hormonal state, which may speed recovery [31].
The increased protein and glycogen synthesis is believed to be due to insulin secretion from the pancreas combined with an increase in muscle insulin sensitivity [38]. This was demonstrated in a study (Fig. 11.2) showing that a carbohydrate–protein combination was 38 % more effective in stimulating protein synthesis than a protein supplement and more than twice as effective as a carbohydrate supplement [39]. Insulin appears to stimulate biosynthetic pathways that lead to increased glucose utilization, increased carbohydrate and fat storage, and increased protein synthesis. This metabolic pattern is characteristic of the absorptive state. The rise in insulin secretion during this state is responsible for shifting metabolic pathways to net anabolism [9]. In contrast, when insulin secretion is low, the opposite effect occurs. The rate of glucose entry into the cells is reduced and net catabolism occurs, rather than net synthesis of glycogen, triglycerides, and protein. This pattern is reminiscent of the post-absorptive state.
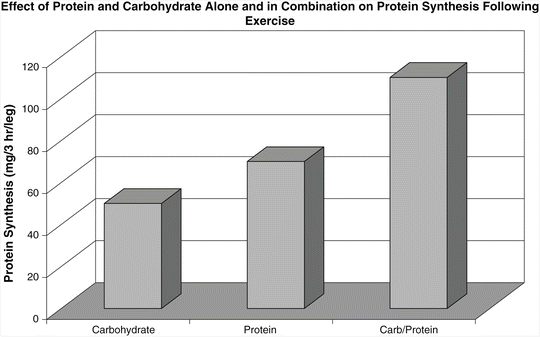
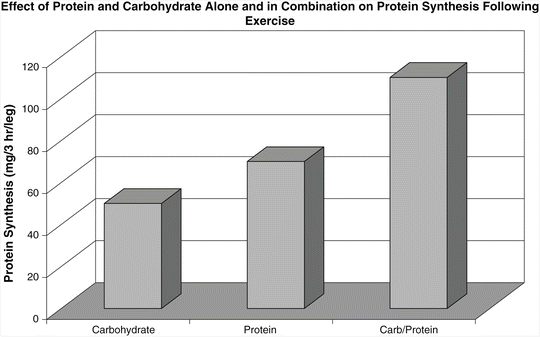
Fig. 11.2
Effect of protein and carbohydrate alone and in combination on protein synthesis, measured after exercise. From Tarnopolsky et al., with permission [30]
11.3.1 Branched-Chain Amino Acids
Another popular nutritional ergogenic aid is the branched-chain amino acids (BCAAs): leucine, isoleucine, and valine. They are prevalent in both protein/meal replacement powders and energy drinks. Table 11.3 shows the typical amino acid composition of some common protein preparations. The RDA for BCAAs is less than 3 g per day, although supplementation studies frequently utilize 5–20 g per day in tablet form and 1–7 g per liter in solutions [40]. The theory behind BCAA supplements relates to a phenomenon known as central fatigue, which holds that mental fatigue in the brain can adversely affect physical performance in endurance events. The central fatigue hypothesis suggests that low blood levels of BCAAs may accelerate the production of the brain neurotransmitter serotonin, or 5-hydroxytryptamine (5-HTP), and prematurely lead to fatigue [41]. Tryptophan, an amino acid that circulates in the blood, is a precursor of serotonin and can be more easily transported into the brain to increase serotonin levels when BCAA levels in the blood are low because high blood levels of BCAAs can block tryptophan transport into the brain [42]. During endurance exercise, as muscle and liver glycogen are depleted for energy the blood levels of BCAAs also decrease, and fatty acid levels increase to serve as an additional energy source [43]. The issue with extra fatty acids in the blood is that they need to attach to albumin as a carrier protein for proper transport. In doing so, the fatty acids displace tryptophan from its place on albumin and facilitate the transport of tryptophan into the brain for conversion to serotonin [44]. Thus, the combination of reduced BCAAs and elevated fatty acids in the blood causes more tryptophan to enter the brain and more serotonin to be produced, leading to central fatigue [45].
Table 11.3
Typical amino acid composition of whey, casein, and soy isolates
Amino acid | Whey | Casein | Soy |
---|---|---|---|
Alanine | 4.6 | 2.7 | 3.8 |
Arginine | 2.3 | 3.7 | 6.7 |
Aspartic acid | 9.6 | 6.4 | 10.2 |
Cysteine | 2.8 | 0.3 | 1.1 |
Glutamic acid | 15.0 | 20.2 | 16.8 |
Glycine | 1.5 | 2.4 | 3.7 |
Histidinea | 1.6 | 2.8 | 2.3 |
Isoleucinea,b | 4.5 | 5.5 | 4.3 |
Leucinea,b | 11.6 | 8.3 | 7.2 |
Lysinea | 9.1 | 7.4 | 5.5 |
Methioninea | 2.2 | 2.5 | 1.1 |
Phenylalaninea | 3.1 | 4.5 | 4.6 |
Proline | 4.4 | 10.2 | 4.5 |
Serine | 3.3 | 5.7 | 4.6 |
Threoninea | 4.3 | 4.4 | 3.3 |
Tryptophana | 2.3 | 1.1 | 1.1 |
Tyrosine | 3.3 | 5.7 | 3.3 |
Valin a,b | 4.5 | 6.5 | 4.5 |
For endurance athletes competing in long races (more than 2 h), BCAA supplements can help delay central fatigue and maintain mental performance [46]. One study looked at BCAA supplementation during a marathon and showed improved performance for slower runners (3+ hours) but no effect on faster runners (less than 3.05 h) [2]. Chronic BCAA supplementation (2 weeks) has also been shown to be effective in improving time-trial performance in trained cyclists [39]. In addition to their effects on prolonging endurance and delaying central fatigue, BCAA supplements have been associated with a reduced rate of protein and glycogen breakdown during exercise and an inhibition of muscle breakdown following exhaustive endurance exercise [41, 47]. A number of studies in trained and untrained subjects, however, have shown no effect of BCAA supplements on exercise performance or mental performance [48]. In some cases, BCAAs have been compared with carbohydrate supplementation during exercise, with results showing they both delay fatigue to similar degrees [43]. The data on BCAA supplements are mixed, but they clearly do not harm endurance performance. Some studies have shown positive adaptations, whereas others have displayed no effect. Biological variations may determine whether BCAAs are effective for the individual athlete and the particular sport or event.
11.3.2 Caffeine
Pharmacological sports ergogenics are drugs designed to function like hormones or neurotransmitter substances that are found naturally in the human body. Like some nutritional sports ergogenics, pharmacological sports ergogenics may enhance physical power by affecting various metabolic processes associated with sport success. The most popular pharmacological sports ergogenics used by endurance athletes today are caffeine and ephedrine. Caffeine is theorized to enhance endurance performance by first stimulating the central nervous system (CNS) and increasing psychological arousal. Caffeine also stimulates the release of epinephrine from the adrenal glad, which may further enhance physiological processes such as cardiovascular function and fuel utilization. The caffeine-mediated increase in free fatty acid mobilization and sparing of muscle glycogen is the primary theory underlying the ergogenic effects of caffeine on prolonged endurance activities. Lastly, caffeine increases myofilament affinity for calcium and/or increases the release of calcium from the sarcoplasmic reticulum in skeletal muscle, resulting in more efficient muscle contractions.
In one of the first studies conducted on caffeine’s ergogenic effect, subjects consumed decaffeinated coffee or decaffeinated coffee combined with 330 mg pure caffeine 60 min prior to exercise. Time to exhaustion was more than 19 % longer in the caffeine trial than in the decaffeinated trial [49]. The authors concluded that the performance increase was more likely due to the increase in fat oxidation, as muscle glycogen was not measured. Another study measured muscle glycogen utilization and found that caffeine prior to exercise reduced muscle glycogen utilization by 30 % [50]. A later study supported the muscle glycogen-sparing hypothesis by reporting a 55 % decrease in muscle glycogenolysis during the first 15 min of exercise in the caffeine trial [51]. The decrease in glycogenolysis during the initial stages of exercise allowed more glycogen to be available during the final stages, subsequently leading to an increased time to exhaustion. Further support of caffeine supplementation was demonstrated in a study examining the effects of acute caffeine ingestion (6 mg/kg) on prolonged, intermittent sprint performance on a cycle ergometer. The total amount of sprint work performed during the caffeine trial was 8.5 % greater than that performed during the placebo trial [52]. The authors concluded that acute caffeine ingestion can significantly enhance performance of prolonged, intermittent sprint ability in competitive male, team-sport athletes.
Despite the overwhelming ergogenic evidence of caffeine supplementation, caution should be exercised when considering its use. A recent study showed that in healthy volunteers a caffeine dose corresponding to two cups of coffee (200 mg) significantly decreased blood flow to the heart during exercise by 22 %. That percentage increased to 39 % for people exercising in a high-altitude chamber, which the researchers used to simulate the way coronary artery disease (CAD) limits the amount of oxygen that gets to the heart [53]. Because an increase in blood to the heart is necessary for aerobic activity, the findings theoretically suggest that caffeine could slow the body down. The study’s purpose, however, was not to look at whether caffeine could help athletes go faster or farther. Instead, it set out to investigate the effect caffeine has on blood flow to the heart. It can thus be concluded that people with CAD or those at a high risk for heart disease should avoid loading up on caffeine before a run or at least check with their primary care physician first.
Though a popular concern of caffeine ingestion has been for dehydration, recent research by Killer et al. (2014) appears to dispel this myth [54]. Using a counterbalanced, crossover design, 50 male coffee drinkers consumed either 4 × 200 mL of coffee containing 4 mg/kg caffeine or water. By examining total body water and deuterium oxide techniques, no significant changes in total body water were noted between trials across any hematological markers or in 24 h urine volume following caffeine ingestion; thus coffee provides similar hydrating qualities to water when consumed in moderation [54].
A final note in regard to caffeine supplementation is that the International Olympic Committee lists caffeine as a banned substance. Although some amount of caffeine is allowed because of its occurrence in foods, a urinary level that exceeds 12 μg/ml results in a doping violation and possible disqualification or suspension. Therefore, it is recommended to keep the daily caffeine intake to less than 3 mg/kg body weight (i.e., 50–200 mg of caffeine). Table 11.4 lists typical caffeine content in common beverages, pills, and other products.
Table 11.4
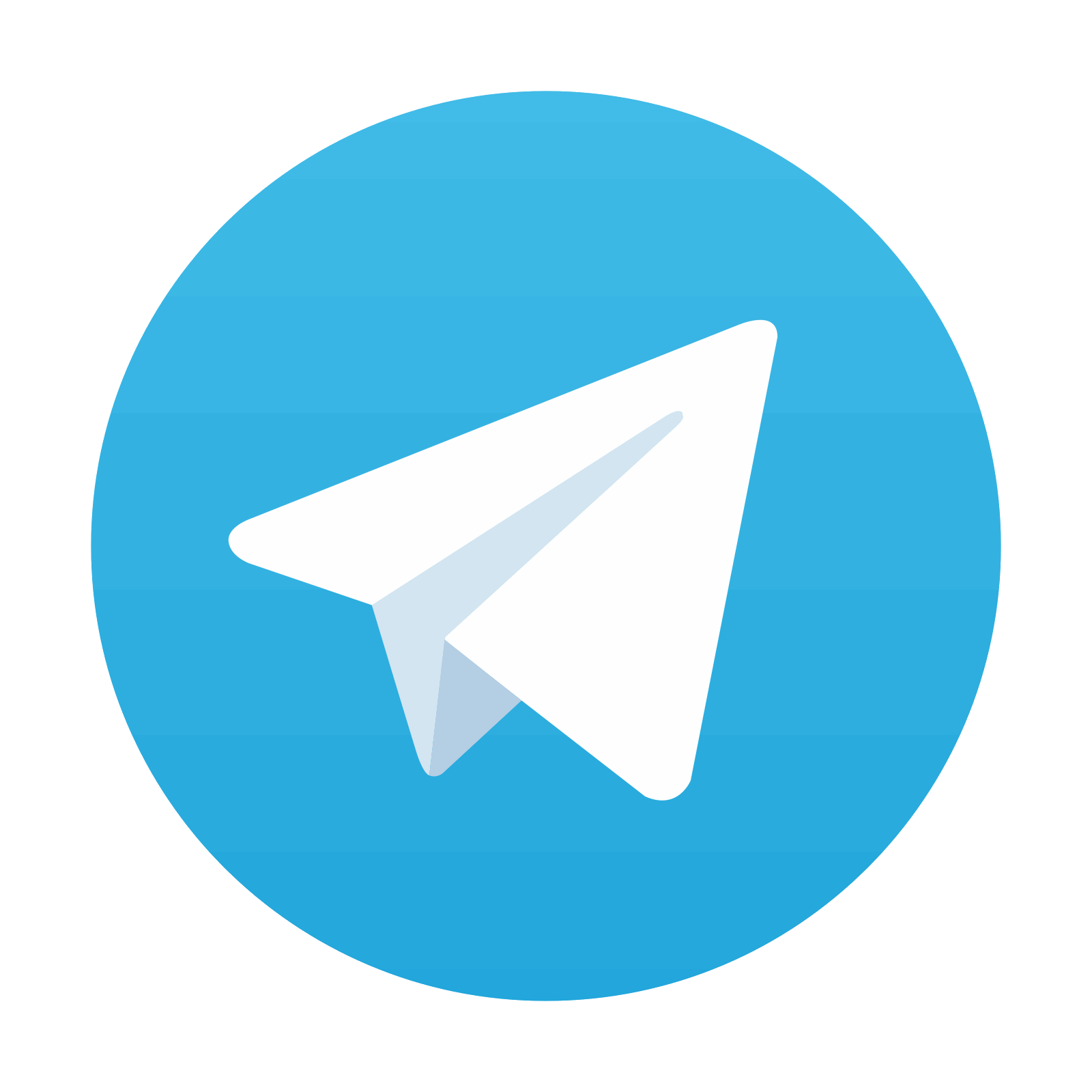
Typical caffeine content in common beverages, pills, and other products
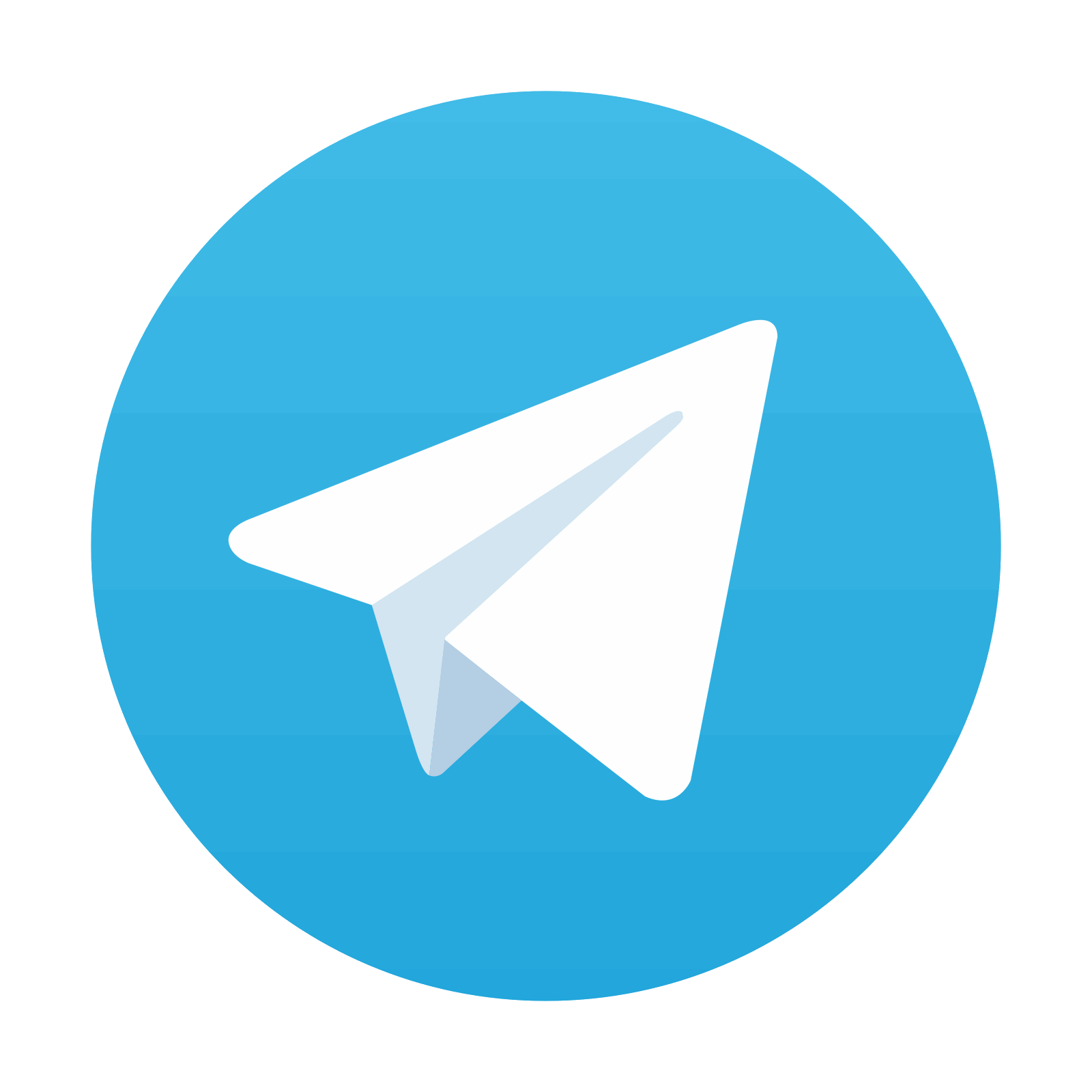
Stay updated, free articles. Join our Telegram channel

Full access? Get Clinical Tree
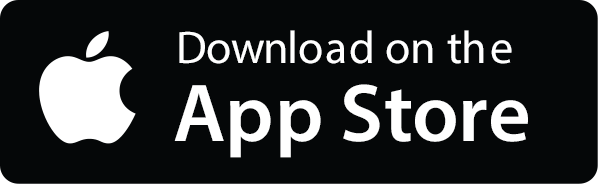
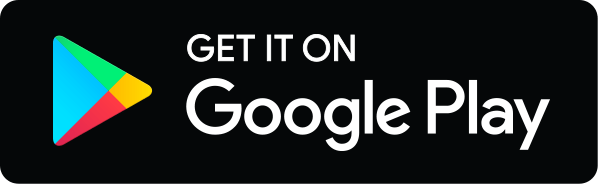
