Reference
Subjects
Study design
Duration
Nutrient
Outcome/comments
Schoenfeld et al. [65]
• 23 studies (n = 525) examining strength
• 20 studies (n = 478) examining hypertrophy
Meta-analysis
N/A
Protein
• Strength: no effect
• Hypertrophy: total protein intake was strongest predictor
• ND between treatment and control for strength or hypertrophy
Weisgarber et al. [114]
• 17 healthy young adults
• No RT for 6 weeks prior
Randomized, double-blind, repeated measures, placebo controlled
4 d/wk for 8 w
• Protein
(0.3 g/kg WPI w/ 0.15 g/kg essential AAs)
• 50 % of total daily dose before and after
• Significant ↑ in muscle mass and some strength measures
• ND between groups
Jakubowicz et al. [100]
• 93 obese/overweight women
• 45.8 ± 7.1 years
• All have metabolic syndrome
Randomized, open-label, parallel-arm design
• 12 weeks
• 2 isocaloric diets (~1,400 kcal)
• 50 % kcals w/breakfast
• 50 % kcals w/dinner
Mixed meals
• Breakfast: ↑ weight loss, ↓ waist circumference, and ↓ blood triglyceride
• Daily glucose, insulin, ghrelin, and mean hunger scores were significantly lower in breakfast group
• Higher mean satiety scores in the breakfast group
Moore et al. [112]
• 24 male subjects participating in regular high-intensity resistance training
• 4–6 x/wk
Randomized grouping
Leg ext (4 × 10 at 80 % 1RM, 3-min rest b/w sets 80-g WPI over 12 h in three patterns:
• 8 × 10g/90 min
• 4 × 20g/3 h
• 2 × 40g/6 h
Whey protein isolate
• ND in net protein balance between groups
• Small to moderate effect for 8 × 10g or 4 × 20g
Mamerow et al. [115]
• 36.9 ± 3.1 y
• BMI: 25.7 ± 0.8
Randomized 7-d crossover feeding study with a 30-d washout period
• 7-d crossover w/30-d washout
• 24-h diet with protein skewed to dinner or evenly distributed
Protein
(feeding study)
• 24-h mixed muscle FSR was 25 % ↑ with even distribution of protein
• Daily protein intake: ~1.2 g/kg
• The animal-to-vegetable protein ratio was ~2:1
Bray et al. [67]
Male wild-type mice
• Randomly assigned to one of four groups
• Access to low- or high-fat diets in various diurnal patterns
• 12 weeks
• Mice were fed either a high-fat or control diet in a time-of-day-dependent manner
Lipid
(dietary)
• Independent of feeding patterns, food intake and energy expenditure were spontaneously adjusted
• Altered dietary composition during the active period markedly influences metabolic health
Res et al.
2012 [116]
16 healthy, young, recreationally active men
Randomized, parallel group design
• Single RT bout at 2,000 h
• Post-RT recovery meal delivered 30 min before sleep (2,330 h) with 40 g protein or PLA
• Phenylalanine-labeled casein protein
• Protein ingested immediately before sleep is:
(1) effectively digested and absorbed, ↑ overnight plasma AA availability, (2) ↑ MPS leading to ↑ net balance
Minor et al. [117]
• Hypocaloric (−15 % kcal): n = 10 (2 M 8 F; 64 ± 2 y; 23.3 ± 0.8 kg/m2)
• Hypercaloric (+15 % kcal): n = 6 (2 M 4 F; 65 ± 2 y; 24.6 ± 1.5 kg/m2)
Each participant completed 2 consecutive, 3-day trials in a randomized crossover design
• 6 days total
• Each 3-day trial were reproduced and identical
• Immediately after exercise vs. earlier in day
Protein
• Anabolic effect of postexercise feeding was evident during positive energy balance but not negative energy balance
Jordan et al. [118]
• 9 healthy, sedentary adults
• 64.5 ± 2.0 y
Each participant completed 2 consecutive, 3-day trials in a randomized crossover design
• 6 days total
• Each 3-day trial were reproduced and identical
• Immediately after exercise vs. earlier in day
Protein
(chocolate milk, 15.3 g protein)
• Older individuals were better able to maintain nitrogen balance by simply changing when a portion of an identical amount of daily protein was consumed
Galloway et al. [18]
• 17 male active team-sports players
• 23.6 ± 4.8 years
Randomized, double-blind, crossover design
• 4 trials (cycling at 90 % of PPO)
• 7-d crossover
ingestion of a 6.4 % GES or PLA 30 or 120 min before exercise
• Glucose-electrolyte solution (6.4 % CHO)
• ↑ in HI ex capacity when 32 g of CHO taken 30 min before exercise (14–17 % increase) vs. 2 h preexercise
Greer et al. [119]
• 9 trained subjects (6 M, 3 W)
• 21.4 ± 1.0 year
Randomized, blinded, crossover design
• CHO + PRO vs. PLA before and after downhill run
• 7d b/w trials
• ~ 3wks total
72 g carbohydrate
+18 g protein (Accelerade®”)
• ND in the 1.5-mile time trial or soreness between trials, regardless of timing
• No impact of CHO + PRO beverage on next day running performance or soreness vs. placebo
Heesch et al. 120]
• 8 trained male cyclists
• 34.5 ± 8.3 years
Randomized, counterbalanced, crossover design
4 separate trials, each with 2-h cycling at 60 % VO2max then 10-km TT:
• No CHO
• Early CHO (1st hour)
• Late CHO (2nd hour)
• CHO throughout
≥3 day washout
• 250 mL of 6 % CHO (early and late conditions)
• 3 % CHO every 15 min
• ND in O2 uptake, HR, RPE, or substrate use between trials (p > 0.05)
• CHO ingestion throughout or late during a 2-h cycling bout can improve subsequent 10-km time trial performance
Trabelsi et al. [121]
16 male recreational bodybuilders (9 Ramadan fasters and 7 nonfasters)
• Anthropometric measurements
• Diet record
• Fasting blood and urine
• Samples taken before and after Ramadan
• 31days (29-d Ramadan + 2d)
• 4 d/wk RT; 4–6 exercises, w/ 4 sets at 10RM and 2–3 min b/w sets
• Progress to 10RM loads
Mixed
~15 h fasting
• Hypertrophic training through Ramadan had no effect on body mass and body composition of bodybuilders
• State of dehydration and reduced renal function were apparent
13.3 Micronutrient Timing
While much of the academic attention devoted to nutrient timing has centered exclusively on the macronutrients, especially protein and carbohydrates, there is emerging research suggesting that timing may also influence the impact of various micronutrients and non-nutrient dietary compounds. As can be expected, the number of studies available at this point in time is quite low, which only underscores the relative infancy of nutrient timing research, as well as the potential for further investigation in these areas. The next section will discuss the research of micronutrients, followed by a summary of timing considerations involving non-nutrients.
Because certain endurance exercise modalities, particularly cycling, have been associated with a negative effect on bone mineral density, Barry et al. (2011) compared the impact of cycling exercise on calcium homeostasis following two different timing strategies for calcium supplementation [76]. Twenty trained male cyclists were asked to perform intense 35-km cycling time trials while consuming a fortified beverage containing one total gram of calcium either 20 min prior to exercise or in equal doses ingested at quarter-hour intervals during the 1-h cycling bout. For each trial, a placebo beverage was provided during the alternate consumption period, and the results were compared against a placebo-only session. These trials were performed in a double-blind, crossover fashion, with at least 48 h between bouts. The authors found that only calcium supplementation prior to exercise was able to significantly diminish (p = 0.04) the expected increase in parathyroid hormone provoked by exercise, though a similar trend (p = 0.07) was seen for supplementation during exercise. Because parathyroid hormone increases in response to even minor decrements in serum calcium levels, the blunted increases reported by this project indicate an improved maintenance of serum calcium as a result of calcium supplementation, and this effect appears to be at least partially moderated by the timing of supplement consumption [76].
These results were bolstered by another recent project presented at the 2013 Endocrine Society annual meeting in San Francisco, CA, which also looked at the impact of calcium supplement timing on cycling exercise-induced disruptions in calcium homeostasis [77]. This study worked with 52 competitive male cyclists, who were randomly assigned to groups consuming supplements containing 1 g calcium and 1,000 IU vitamin D either 30 min before or 1 h after a strenuous 35-km cycling time trial. These researchers found that the observed decrease in serum ionized calcium postexercise was significantly less for the group receiving supplements prior to exercise, and while parathyroid hormone increased after cycling for both groups, the increase was attenuated by preexercise supplementation [77].
In a similar study performed by Shea et al. (2014), researchers again compared the effects of disparate timing strategies for calcium supplementation on the disruptions to calcium homeostasis caused by endurance exercise [78]. This project carried out two separate double-blinded, crossover experiments using healthy, postmenopausal women aged 50–75 years performing 60 min of treadmill walking at 75–80 % VO2peak. The first experiment asked ten subjects to consume a calcium-fortified beverage or placebo in equal quantities every 15 min, beginning 1 h prior to exercise and continuing throughout the hour-long bout to deliver a total dose of 1 g of calcium. The second experiment asked a group of 23 subjects to consume an equivalent total measure of calcium or placebo, this time beginning just 15 min prior to exercise and once again continuing throughout the exercise session. This project found that the anticipated increase in parathyroid hormone following exercise was significantly reduced for only those subjects beginning calcium supplementation 60 min before activity. Additionally, all conditions saw a significant decrease in serum ionized calcium except the group receiving supplementation for a full hour prior to exercise [78].
Altogether, these projects provide firm support for a variable influence of calcium supplementation on exercise-induced disruptions to calcium homeostasis, which seems to be largely dependent on the timing of intake relative to exercise. While there is currently a paucity of research examining the influence of micronutrient timing on their subsequent effects, especially in relation to exercise, this is largely because many vitamins and minerals are present in durable stores built up over time throughout the physiological system, so that daily timing of intake seems less pivotal to the overall impact of these nutrients. The results of these projects investigating calcium dynamics help indicate that more research is necessary to provide a solid empirical foundation for that assumption on a nutrient-by-nutrient basis, though not every nutrient is likely to demonstrate a timing-dependent effect.
In a recent animal study conducted by Fujii, Matsuo, and Okamura (2011), the research team used rats with induced iron deficiency to examine the impact of resistance training and postexercise meal timing on iron status and heme synthesis [79]. In light of previous research demonstrating an increase in heme synthesis following resistance exercise in iron-deficient rats [80], these researchers hypothesized that earlier postexercise feeding may potentiate this effect. Two groups of 4-week-old male rats were then given similar, iron-deficient feed either immediately or 4 h after performing a climbing exercise three times per week over a 3-week period. While plasma iron was significantly elevated after climbing for only the group receiving immediate postexercise feeding, the hematocrit and hemoglobin levels were similar between groups pre- to postexercise [79]. As such, the researchers determined that postexercise meal timing had no effect on the hemoglobin concentration, and this project helps highlight that the complexity of intake timing, nutrient handling, and exercise interactions is such that repeated, comprehensive investigation may be required to tease out the underlying chain of effects.
13.4 Non-nutrient Timing
In addition to micronutrients, the timing component of other dietary constituents or non-nutritive supplements, particularly those intended to provide an ergogenic benefit, is rarely included in nutrient timing considerations. Nonetheless, timing is often a crucial and underexplored component of many supplemental strategies for ergogenic aid. Table 13.2 outlines the study design of the small number of studies examining timing of non-nutrients.
Table 13.2
Summary table of selected studies examining timing of non-nutrients
Reference | Subjects | Study design | Duration | Nutrient | Outcomes/comments |
---|---|---|---|---|---|
Ryan et al. 2013 [82] | • 8 college-aged (25 ± 5 years) male cyclists • 50 ± 5 ml∙kg−1∙min−1) | A within-subject, repeated measure, placebo-controlled design | • 4 sessions w/in 1 month • 3 sessions with caffeine given a different time point each • 1 PLA trial | Caffeine | • Performance ↑ when gum was given immediately before • ND when offered 1 or 2 h before |
Candow et al. [90] | • 22 adults (9 M, 13 F) • 50–64y • No RT for 6 months prior | Randomized, double-blind, repeated measure design | • RT 3 d/wk × 12 wks • CRE (0.1 g/kg) before PLA after • PLA before CRE after | Creatine (0.1 g/kg) | • Both groups ↑ strength and muscle mass • ND between groups |
Antonio et al. 2013 [89] | • 19 healthy males • 23.1 ± 2.9 years • 80.2 ± 10.4 kg | • Randomized trial • Ingest 5 g before or after training | ~60-min RT 5 d/wk for 4 weeks | 5-g/d creatine | • Postexercise group had significantly greater increase in FFM and bench press strength |
Siegler et al. [94] | • 8 active and healthy males • 22 ± 2 years • 76.2 ± 9.1 kg | • Randomized (counterbalanced)• Repeated measure design • Subjects ingested 0.3 g∙kg−1NaHCO3 at 60, 120, or 180 min before exercise | 3 × 10s sprints separated by 50s of active recovery (1:5 work-to-rest) on a nonmotorized treadmill | NaHCO3 (0.3 g∙kg−1) | • ND b/w groups in blood buffering or ergogenic potential of NaHCO3 • 180-min group reported significantly less GI upset |
Caffeine is one of the most commonly consumed psychoactive substances in the world and has been widely demonstrated to have ergogenic properties when applied to a variety of exercise modalities [81]. Unlike many other ergogenic supplements, the widespread social consumption of caffeine means that it is often both available and acceptable for athletes to use during training and even competition. Despite this pervasive popularity and established ergogenic efficacy, a recent project conducted by Ryan et al. (2013) was one of the first to examine the differential impact on performance ensuing from a range of timing strategies for preexercise caffeine consumption [82]. In a randomized, double-blinded, crossover fashion, these researchers provided eight male cyclists with 300 mg of caffeine in a chewing gum at 2 h, 1 h, or 5 min prior to a standardized warm-up and cycling time trial. This method of administration was chosen largely because it has been demonstrated to provide faster absorption than pill consumption, and a matched placebo was supplied at all other time points. The authors found that a significant improvement in time trial performance was only observed when caffeine was dispensed to athletes immediately prior to exercise [82]. Overall, these results were the first to illustrate the impact of timing of caffeine on cycling performance while also employing an administration method that may offer benefits over capsule or liquid delivery systems.
Outside of the recent work by Ryan, a number of studies using both endurance and resistance exercise have provided varying dosages of caffeine prior to beginning a workout or competition and have reported widespread improvements in performance. In endurance-style exercise, caffeine prior to exercise is known to favorably impact substrate utilization, reduce perceptions of fatigue, increase drive to exercise, and enhance overall performance [81, 83, 84]. Similarly, a number of studies published in the last 5 years have reported favorable outcomes for caffeine ingestion prior to resistance exercise, leading to improvements in maximal strength, maximal repetitions completed, and drive to exercise, as well as reductions in soreness and muscle pain [85–87]. Collectively, supplementation with caffeine leads to significant reductions in muscle pain, improvements in mood, improvements in maximal strength, and improvements in the maximal number of repetitions performed. Outside of the Ryan study, a key discussion point for all research highlighted involving caffeine is that none of the studies were developed to examine a question of timing. These studies are included because the nature in which caffeine operates results in a situation where preexercise administration is needed to fully take advantage of its stimulatory effects, particularly as they would work toward enhancing performance. In this respect, providing caffeine after a bout of resistance exercise would not make any pharmacological, physiological, or theoretical sense. For these reasons, caffeine use prior to both endurance and resistance exercise has a strong potential to exert favorable performance and metabolic outcomes. One final thought on caffeine and timing relates to the work of Pedersen et al., whereby the authors reported that postexercise ingestion of caffeine at a dose of 8 mg per kilogram of body mass, when combined with carbohydrate feedings, resulted in the fastest published rates of glycogen resynthesis that have ever been reported [88]. Again, this study did not examine caffeine timing per se, but demonstrated that providing it in a postexercise window with carbohydrate feedings can result in enhanced rates of muscle glycogen resynthesis. The efficacy of doing so prior to exercise and its impact on performance, glycogen storage, or utilization remains to be seen.
Another popular non-nutritive dietary supplement with demonstrated ergogenic benefit, creatine monohydrate, has also been shown through several research projects to merit involvement in the nutrient timing discussion. In one of the seminal studies on supplement timing and performance, Cribb and Hayes (2006) provided matched groups of resistance-trained males with a supplement containing protein, carbohydrate, and creatine monohydrate throughout a structured 10-week training period [61]. Subjects consumed the supplement either immediately before and after four weekly resistance training workouts or in the early morning and late evening of training days in a randomized, single-blind fashion. A number of timing-specific effects were observed by the researchers, including significantly greater improvements in lean body mass and muscular strength for the group receiving supplementation immediately before and after training. Most importantly for creatine users, after the training period, researchers found significantly greater phosphocreatine and creatine concentrations in muscle biopsies from the group supplementing before and after exercise, indicating an enhanced effect of creatine use dependent on intake timing.
This conclusion was refined in a subsequent project performed by Antonio and Ciccone (2013). These researchers randomly assigned a group of 19 recreational male bodybuilders to receive 5 g of creatine monohydrate either immediately before or after a structured, 4-week resistance training regimen [89]. Though trends in the resulting data failed to reach significance, perhaps due to the small sample size utilized and the relatively short duration of the resistance training program, the authors used a magnitude-based inference analysis approach to conclude that postexercise supplementation was potentially superior in producing beneficial changes to fat-free mass, fat mass, and 1-RM bench press.
These somewhat tenuous results were then confounded by another recent study conducted by Candow et al. (2014). This project also compared pre- versus postexercise creatine supplementation, this time in a group of 22 untrained older adults of mixed gender. In a double-blind design, these subjects were randomized into groups receiving 0.1 g of creatine per kilogram body weight, supplied either immediately before or after structured resistance training sessions performed three times per week over 12 weeks. The authors found no significant difference between groups in the ensuing adaptations to resistance training, with both groups experiencing similar significant increases in lean tissue mass, muscle thickness, and muscle strength [90].
While the authors of these two projects reached disparate conclusions, there are a variety of important physiological considerations impacting these studies that can help highlight the level of nuance able to effect research of this nature. First and foremost, earlier work on creatine estimates that approximately 20–30 % of individuals fail to respond to creatine supplementation [91]. In light of the relatively small subject samples employed by both of these projects, any imbalance in the grouping of nonresponders during randomization could have significantly affected the subsequent results. Moreover, previous studies have reported male subjects as responding more favorably to creatine supplementation than females [92], so that the use of a mixed-sex sample by Candow et al. (2014) may have been nontrivial. Other inherent differences between these two projects, including absolute versus relative creatine dosage strategies, as well as subject age, training status, and the duration of the training program, could each have plausibly differentiated the eventual results. Altogether, the potential impact of these minor disparities points to the need for carefully conducted future research in order to distinguish any potential timing effect for creatine and other dietary supplements hitherto unconsidered.
Indeed, beyond augmenting the ergogenic benefit of these compounds, intake timing may also play a role in moderating any negative side effects accompanying these products. A straightforward and effective timing strategy is often used to mitigate the paresthesia associated with effective doses of beta-alanine, in simply breaking the dosage into smaller quantities consumed throughout the day [93]. Similarly, a recent project conducted by Siegler, Marshall, Bray, and Towlson (2012) exploring timing strategies for sodium bicarbonate supplementation found that intake timing impacted subsequent reports of gastrointestinal upset. Using a randomized, counterbalanced design, these researchers provided a group of eight male sprinters with 0.3 g of sodium bicarbonate per kilogram body weight at 60, 120, or 180 min prior to repeated bouts of sprinting. The authors found that the subjects performed similarly under all supplemental conditions, yet reports of gastrointestinal discomfort were significantly elevated for only those subjects receiving sodium bicarbonate at 60 and 120 min prior to exercise [94].
13.5 Meal Patterns and Time-of-Day Considerations
While it is true that the entire concept of nutrient timing, particularly as it relates to sports and exercise, has overwhelmingly involved timing considerations closely surrounding some form of workout or competition, the strategy itself and the potential advantages it may offer can also apply to timing strategies for other scenarios such as health and weight loss. An early study published in 1997 by Keim and investigators required study participants to complete two 6-week diet periods. Similar diet compositions and caloric contents (~1,950 kcals) were consumed, but in one pattern, approximately 70 % of the prescribed kcals were consumed during the morning, while in the other group 70 % of the prescribed kcals were consumed with evening meals. Changes in weight loss and body composition were compared, and slightly greater weight loss occurred when the majority of kcals were consumed in the morning. While this is a seemingly positive outcome for consuming more kcals in the morning, greater amounts of fat-free mass were lost as well, leading to questions surrounding the long-term efficacy of this strategy regarding weight management and metabolic activity [95]. Observational research by investigators at the University of Texas-El Paso examined the food intake of 867 free-living individuals (375 males and 492 females) and reported that the timing of food consumption is correlated to the overall amount of kcals consumed each day, with consumption early in the day being negatively correlated with total food intake (r = −0.13, p < 0.01), while late in the day consumption was positively correlated (r = 0.14, p < 0.01) [96]. A more detailed, follow-up analysis from the same study revealed that when carbohydrates, fats, and proteins were consumed in greater amounts earlier in the day, then reduced amounts were consumed later in the day. Conversely, when proportionally greater amounts were consumed later in the day, overall energy intakes tended to be higher [68].
In addition to changes in food intake, research in both animals and humans shows that the timing of food consumption may impact changes in body fat accumulation and production. Wu and investigators reported in laboratory animals that the first daily meal helps to program the circadian phase of peripheral clocks, while the last meal leads to increased rates of lipogenesis and adipose tissue accumulation [97]. Human research supports these findings, in that people who skip breakfast have been shown to display a delayed activation of lipolysis along with an increase in the production of adipose tissue [98, 99].
A more recent study conducted by Jakubowicz et al. in 2013 required overweight and obese women to consume a weight loss diet that provided approximately 1,400 kcals each day for a 12-week period. In this study, a portion of the study participants consumed 700 kcals during breakfast, 500 kcals during lunch, and 200 kcals during dinner, while the other portion of study participants consumed the exact opposite distribution (200 kcals for breakfast, 500 kcals for lunch, and 700 kcals for dinner) [100]. Approximately 2.5 times more weight was lost when the majority of kcals were consumed at breakfast, as compared to the opposing feeding pattern. In addition, significantly greater changes in waist circumference and overall body mass index were observed when the majority of kcals were consumed at the breakfast meal. Equally impressive findings from this study were that average triglyceride levels decreased by 34 % in the breakfast consumption group, as compared to a 15 % change in the dinner group, while greater improvements in serum glucose and insulin dynamics as well as improved feelings of satiety were also found in the group that consumed the majority of their kcals at breakfast [100].
Overall, the results of the Jakubowicz study provide additional empirical support for a number of other previously published reports. In both human and animal research, the practice of skipping breakfast and altering the macronutrient content of certain meals has been repeatedly shown to impact appetite, feelings of fullness, and overall food intake. For example, Leidy and colleagues in two separate published studies concluded that increasing the protein content of the breakfast meal can improve appetite control, food intake, and feelings of fullness [101, 102]. In closing, the Jakubowicz study offers an excellent extension of these findings and helps to illustrate that meal timing and the temporal distribution of kcal consumption may indeed impact weight loss, body composition, and various parameters of metabolic and cardiovascular health [100].
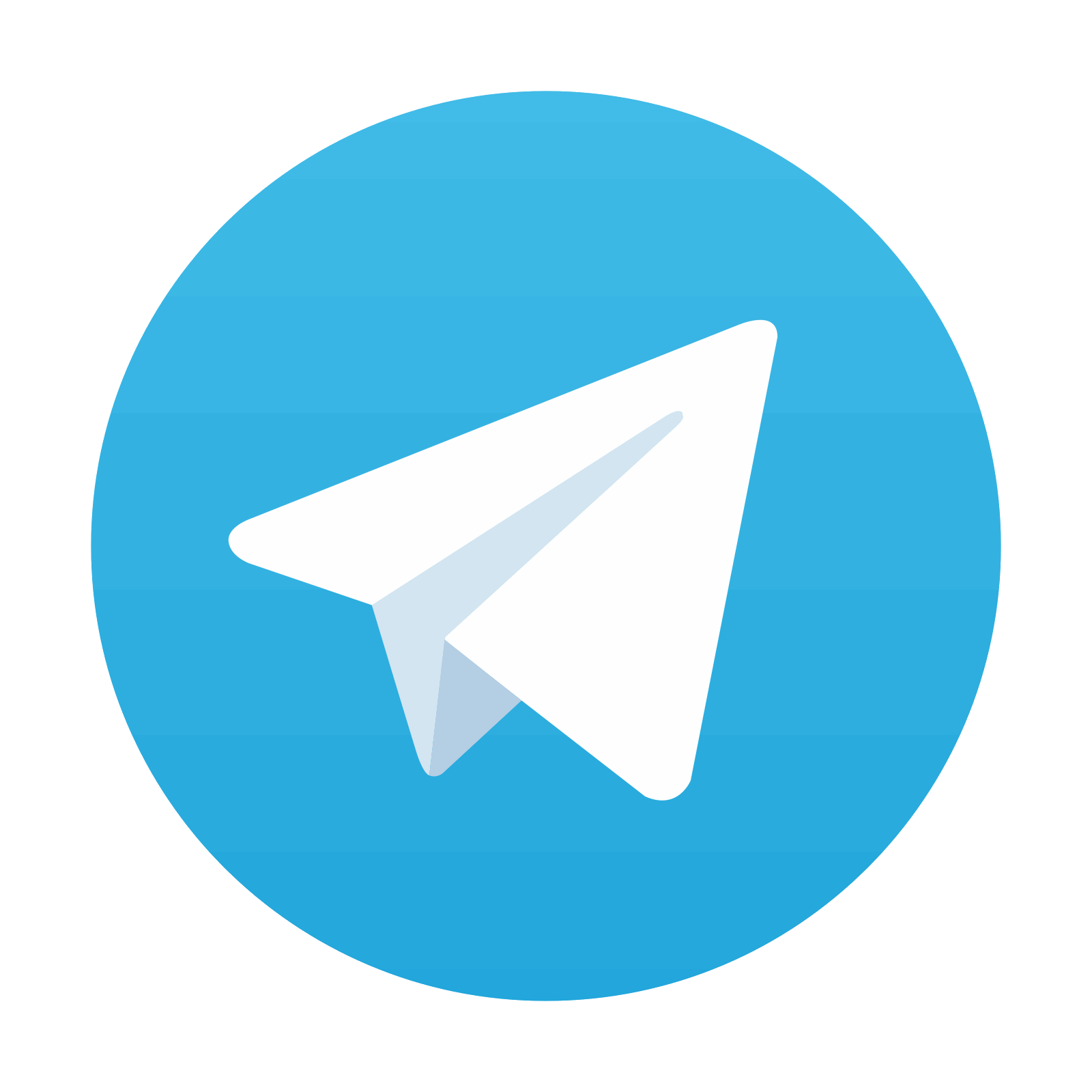
Stay updated, free articles. Join our Telegram channel

Full access? Get Clinical Tree
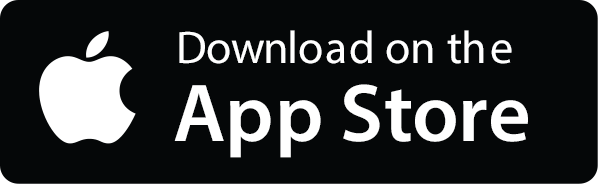
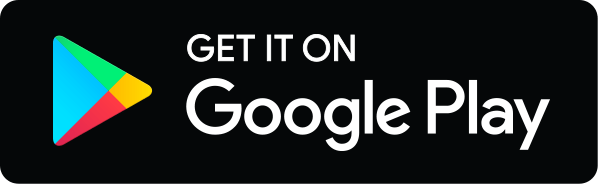