Fig. 1
Treatment with minocycline and ebselen reduced endothelial permeability, plaque burden, and gadofosveset uptake. A1 through D1 and A2 through D2, Cross-sectional delayed enhancement magnetic resonance imaging (DE-MRI) fused with magnetic resonance angiography (MRA) images of the brachiocephalic artery. ApoE−/− mice on a high fat diet showed increased vessel wall enhancement corresponding to plaque progression, whereas minocycline- and ebselen-treated ApoE−/− mice showed less enhancement. A3 through D3, Corresponding R1 maps quantify the amount of gadofosveset within the vessel wall. Intense yellow signal indicates increased gadofosveset concentration
4 Iron Oxide-Based Contrast Agents
Iron oxide-based contrast agents include nano-sized, ultra-small super-paramagnetic particles of iron oxide (USPIO) (20–50 nm), super-paramagnetic particles of iron oxide (SPIO) (60–250 nm) and micron-sized microparticles of iron oxide (MPIO) (0.9–4.5 μm). These contrast agents create hypo-intense signal or “negative” contrast areas on MR images, by shortening T2 and T2 * relaxation times, providing greater MRI contrast sensitivity compared to Gd chelates [54, 55]. USPIO have become popular owing to their long blood half-life compared to Gd agents, which is a positive attribute for applications such as measurement of changes in cerebral perfusion. However, this property may cause high background contrast for an extended period, thus reducing its visibility. Furthermore, UPSIO contrast is manifest in T2*-weighted images as indistinct low-signal areas that may be difficult to distinguish from normal tissue heterogeneity and other susceptibility artifacts. To overcome this limitation, positive contrast techniques have been developed [56], which generate positive MRI signals and improves detection of USPIO accumulation in macrophages [57].
MPIO carry the highest payload of iron oxide (typically 0.1–1.6 pg iron/MPIO particle), which is orders of magnitude greater than nano-sized iron oxide particles [58]. MPIO produce a hypo-intense contrast “blooming effect” on MR images that extends up to 50 times the physical diameter of the microparticle. This produces strong contrast effects by in vivo MRI enabling detection of single cells with high sensitivity, using only small numbers of MPIO [59, 60]. The rapid clearance of MPIO from the circulation (within minutes) [61, 62] minimizes background contrast enabling MRI to commence immediately after MPIO administration [63] as opposed to USPIO, which require up to 48 h to clear from the circulation. MPIO are less susceptible to vascular egress or uptake by endothelial cells due to their size and incompressible nature. MPIO can be readily functionalized with single or multiple targeting ligands, such as monoclonal antibodies, peptides or sugar ligands, providing a versatile contrast agent for molecular MRI of endovascular targets such as endothelial adhesion molecules and activated platelets [64].
5 Macrophage imaging
USPIO are typically phagocytosed by plaque-resident or blood-born macrophages and have been shown to accumulate in macrophage-rich atheroma in rabbits [65]. Enhanced USPIO retention has been demonstrated when monocyte recruitment is promoted by cytokine injection in mice [66]. Clinical studies in symptomatic patients scheduled for carotid endarterectomy showed preferential USPIO uptake in macrophage-rich plaques by histology and electron microscopy. USPIO uptake was higher (75 %) in ruptured and rupture-prone lesions compared to stable lesions (7 %) [67]. Targeted MRI of macrophages in atherosclerotic plaques in apoE−/− mice has been achieved using paramagnetic Gd-containing micelles targeted against macrophage scavenger receptor-A (MSR-A) [35]. Bimodal MSR-A-targeted micelles containing Gd chelates and a fluorescent label (either rhodamine-conjugated lipid in the micelle or quantum dots in the micelle corona) have also been developed and applied for in vivo MRI of macrophages in mice. Pronounced MR signal enhancement of up to 200 % was observed in the abdominal aorta of apoE−/− mice injected with MSR-A-targeted micelles, with minimal SE in mice injected with nontargeted micelles [68]. Ex vivo imaging with UV illumination confirmed quantum dot accumulation in regions with high macrophage content.
Nanoparticles conjugated with radiolabeled elements (64Cu, 18F) and/or fluorescent dyes have also been developed for multimodal imaging of macrophages which may decrease the detection threshold and nanoparticle dose required compared to MRI. Nahrendorf and colleagues have synthesized a tri-reporting cross-linked iron oxide nanoparticles (CLIO), decorated with diethylene triamine pentaacetic acid (DTPA) to transchelate radiolabel 64Cu [69]. The 64Cu-CLIO detected macrophages in inflammatory atherosclerotic lesions by PET/SPECT imaging, using an iron dose that was ~100 times lower as compared to MRI. Jarrett et al. have also designed multimodal nanoparticle probes for PET and MRI containing either Gd or iron oxide conjugated to 64Cu which identified regions of macrophage accumulation in apoE−/− mice with vascular inflammation induced by ligation [70]. The disadvantage of 64Cu is that it is not widely available, whereas 18F is more widely available, and has higher sensitivity, shorter half-life, and the ability of irreversible covalent bonding [71]. PET-CT imaging using 18F-radiolabeled CLIO detected significantly higher PET signal in murine aortic aneurysms compared to normal aorta, due to enhanced uptake by macrophages within the aneurysm [72].
6 Vascular Endothelial Dysfunction in Atherosclerosis
Vascular endothelial dysfunction promotes atherosclerotic lesion development through endothelial activation and increased endothelial permeability, leukocyte and monocyte arterial infiltration, platelet activation, and smooth muscle cell proliferation [73]. The deposition of oxidized low-density lipoprotein in the vascular wall leads to localized endothelial expression of pro-inflammatory cytokines, such as interleukin-1β and tumor necrosis factor-α [74]. The localized increase in cytokine levels results in up-regulation of cell adhesion molecules, such as vascular cell adhesion molecule-1 (VCAM-1), and intercellular adhesion molecule-1 (ICAM-1), as well as P-selectin and E-selectin, expressed on the activated endothelial surface. This in turn promotes monocyte recruitment to the vessel wall, with initial monocyte rolling along activated endothelium mediated by interactions between endothelial P-selectin and its ligand, P-selectin glycoprotein ligand-1 (PSGL-1) integrin, expressed on monocytes. Firm adhesion of monocytes is mediated by VCAM-1 (CD106) and it ligand, very late antigen-4 (VLA-4, also known as α4β1 integrin) [75]. In the following sections, we examine targeted molecular imaging approaches, which harness these adhesion molecule signals used for leukocyte recruitment during atherosclerotic lesion development.
7 Molecular MRI Using VCAM-1-Targeted Nanoparticles
VCAM-1 has been at the center of attention for molecular imaging of vascular inflammation since it is not constitutively expressed on the normal vascular endothelium but is rapidly up-regulated upon endothelial activation. Its endothelial location makes it readily accessible for targeted blood borne contrast agents [76]. VCAM-1 is expressed by vascular endothelial cells in both early and advanced atherosclerotic lesions [77] and is also up-regulated by macrophages and smooth muscle cells in atherosclerotic plaques. The expression of VCAM-1 is confined to predisposed arterial regions such as the inner curvature of the aortic arch and its bifurcations, which are exposed to disturbed blood flow and low shear stress [78, 79]. Several generations of targeted nanoparticles have been developed for molecular imaging of VCAM-1. The first VCAM-1 targeted nanoparticles were based on CLIO conjugated to either VCAM-1 monoclonal antibody [80] or VCAM-1-targeting peptides, identified by phage display, with homology to VLA-4 [81, 82]. In vivo MRI studies confirmed detection of VCAM-1 expression using VCAM-1-targeted CLIO in a murine ear inflammation model and in the aortic root of atherosclerotic apoE−/− mice fed a high-fat diet [82]. However, these nanoparticles were also susceptible to non-specific uptake by macrophages, due to their small size and long blood clearance time (48 h). USPIO conjugated to a VCAM-1 targeting cyclic peptide, termed P03011, have recently been reported to detect VCAM-1 expression in early and advanced atherosclerotic lesions using in vivo ultrahigh-field strength MRI (17.6 T), with minimal nonspecific macrophage uptake of non-targeted USPIO [83]. The lack of nonspecific uptake of nontargeted USPIO may be due to the shorter blood clearance time or differences in their surface properties, size, or dose compared to previous USPIO studies. VCAM-1-targeted USPIO were observed to co-localize with macrophages, luminal endothelial-like cells and medial smooth muscle cells using high-resolution Stokes Raman scattering (SECARS) microscopy.
USPIO targeted to novel peptides targeting VCAM-1 (USPIO-R832) have also been reported to enable rapid in vivo MRI (4.7 T) detection of aortic VCAM-1 expression in advanced atherosclerotic plaques in apoE−/− mice at ~30 min, which was maintained until 90 min [84]. The use of USPIO (27 nm) covalently conjugated to PEG as opposed to previously reported dextran coated USPIO formulations was reported to act as a stealth coating to reduce nonspecific uptake into atherosclerotic plaques and enable rapid blood clearance. Co-localization of the USPIO-R832 agent was primarily to macrophage-rich areas of advanced atherosclerotic plaques, which is a downstream target compared to endothelial-specific imaging provided by targeted MPIO.
The targeted nanoparticles developed for molecular imaging of VCAM-1 are generally spherical in shape. However, non-spherical nanoparticles may enable improved margination to the vessel wall and therefore enhance presentation of targeting ligands. Bruckman et al. have exploited the elongated tubular structure of the tobacco mosaic plant virus (TMV) (300 × 18 nm) to engineer a novel Gd-based, near-infrared fluorescent nanoparticle, conjugated with VCAM-1-targeting peptides for dual-MRI-optical imaging of VCAM-1 expression in the aorta of atherosclerotic ApoE−/− mice (Fig. 2) [85]. VCAM-TMV were rapidly cleared from the circulation within minutes and reached rapid steady-state accumulation at the target site, with maximal signal-to-noise ratio (SNR) achieved 90 min post-VCAM-TMV injection. The Gd dose of VCAM-TMV was 400 times lower than the Gd dose used for clinical MR angiography, demonstrating the utility of targeted nanoparticle delivery for improved Gd detection. Non-targeted PEG-TMV showed negligible passive accumulation in atherosclerotic aortas by MRI and immunofluorescence imaging confirmed significantly greater VCAM-TMV accumulation at the intima-media interface. However, while these VCAM-1-targeted nanoparticle approaches can give insights into the vascular inflammatory processes in atherosclerosis, they lack specificity for specific cell types and their cellular uptake has potential for toxicity.
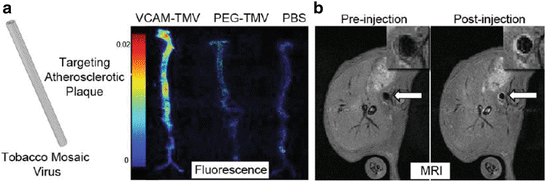
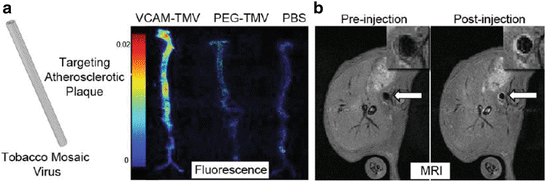
Fig. 2
(a) Ex vivo fluorescence of aortas from ApoE−/− mice injected with (from left to right) VCAM-TMV, PEG-TMV, PBS. (b) Pre- and post-injection MRI scans of VCAM-TMV in ApoE−/− mouse. The inset is a magnified image of the abdominal aorta region of interest [85]
8 Molecular Imaging of Endothelial Adhesion Molecules Using Targeted MPIO
Targeted MPIO, due to their large micron size and conspicuous MRI contrast effects, offer a tool for specific targeting of endothelial molecular biomarkers, such as endothelial adhesion molecules [86, 87]. We have developed VCAM-1-targeted MPIO (1 μm diameter) (VCAM-MPIO) and applied them for in vivo MRI of acute cerebral endothelial activation in mice [88]. In vivo T2*-weighted MR images showed potent, hypo-intense contrast effects in the activated cerebral hemisphere, corresponding to VCAM-MPIO binding, with no contrast effects observed in the non-activated cerebral hemisphere or in animals injected with VCAM-1 blocking antibody. Importantly, this technique also enabled 3-D volumetric mapping of VCAM-MPIO binding throughout the brain at a time when conventional, clinically used MRI techniques do not reveal abnormalities (Fig. 3a) [88]. In addition, inflammatory foci of leukocytes around vessels were shown to be VCAM-MPIO positive but Gd-DTPA negative [89]. Targeted VCAM-MPIO have also been applied for in vivo molecular MRI of endothelial activation following recent ischemia, in mouse models of unilateral renal ischemia-reperfusion injury (IRI) [90], experimental stroke [91], and myocardial IRI [92]. VCAM-MPIO have also demonstrated efficacy for discriminating reductions in endothelial activation following ischemic pre-conditioning [91] and for monitoring anti-interleukin 17A (IL-17A) therapeutic treatment in a chronic relapsing EAE mouse model [93]. Anti-IL-17A treatment was shown to delay relapse, and improve functional scores, and was associated with reduced VCAM-MPIO binding on the cerebral vasculature during remission compared to IgG treated animals (Fig. 3b). No significant differences in levels of Gd-DTPA- or VCAM-MPIO-positive lesions were observed during relapse. Therefore, VCAM-MPIO may be a more sensitive marker of disease activity than Gd-DTPA.
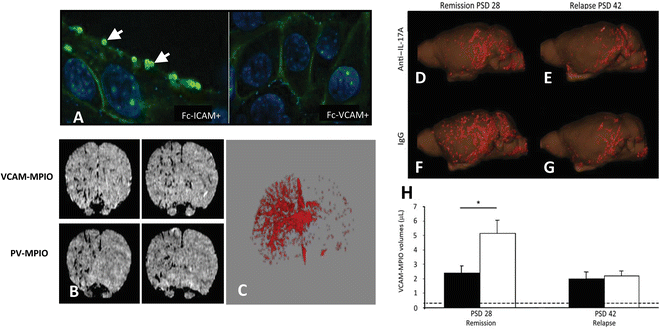
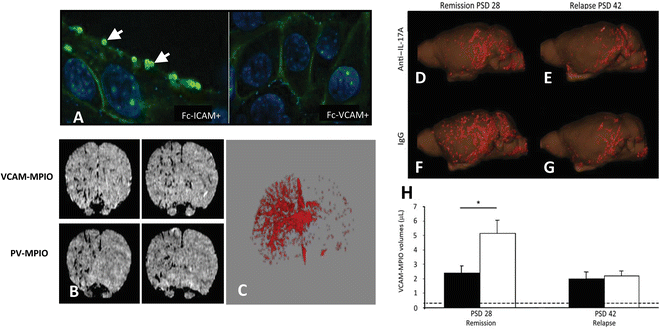
Fig. 3
(a) VCAM-MPIO binding (autofluorescent spheres (see arrows)) on the surface of sEND-1 cells, stimulated with TNF-α co-localizes with VCAM-1 immunofluorescence. Preincubation of VCAM-MPIO with Fc-VCAM-1 abolished VCAM-MPIO binding, despite cell surface expression of VCAM-1, as confirmed by immunofluorescence while pre-incubation with FcICAM-1 did not inhibit VCAM-MPIO binding. (b) T2*-weighted 3-D gradient echo MR image showing intense low-signal areas on the left IL-1β-stimulated brain hemisphere reflecting specific VCAM-MPIO with virtually absent contrast effects in the contralateral hemisphere. Similar, unilateral contrast effects were observed in mice administered dual-targeted VCAM-1 and P-selectin MPIO (PV-MPIO). (c) 3-D volumetric map of segmented VCAM-MPIO contrast delineating the architecture of inflamed cerebral vasculature in the left IL-1β-stimulated hemisphere with absence of binding on the contralateral side [88]. Three-dimensional reconstruction showing that anti-IL-17A treatment of mice with chronic relapsing experimental autoimmune encephalitis (CR-EAE) mice (d) exhibited less MPIO binding during remission (post-sensitization day (PSD) 28) than IgG-treated mice (f). On PSD 42, no significant differences in MPIO binding were observed (e and g, respectively). (h) A significant reduction in VCAM-MPIO binding in anti-IL-17A-treated animals (black bars) was found compared with IgG-treated controls (white bars) on PSD 28, but no significant difference was observed on PSD 42. Data are shown as means SEM. *P < 0.05. Dashed lines represented baseline VCAM-MPIO binding in naive animals
9 Molecular MRI of adhesion molecules in atherosclerosis
Targeted endovascular imaging of large arteries, such as the aorta, is more challenging since the contrast agent must bind to the endothelial monolayer in sufficient quantity and with high affinity and specificity, under conditions of high shear stress [94]. To address these challenges, we have developed dual-targeted MPIO (4.5 μm diameter), conjugated to VCAM-1 and P-selectin monoclonal antibodies (50:50 ratio) to mimic in vivo leukocyte-binding pathways [95]. Dual-targeted MPIO enabled high-resolution ex vivo MRI (9.4 T) detection of endothelial inflammation in advanced atherosclerotic plaque in the aortic root of apoE−/− mice fed a high-fat diet. However, the numbers of MPIO retained on the aortic root were thought to be insufficient for in vivo molecular MRI using acceptable iron doses. We therefore constructed a second generation of smaller 1 μm diameter, dual-targeted MPIO which we hypothesized would be less buoyant under high shear stress enabling more effective ligand presentation and improved MPIO binding, using lower iron doses [63]. In vitro flow chamber studies confirmed that dual VCAM-1 and P-selectin antibody-conjugated MPIO enabled greater binding affinity and specificity to activated endothelial cells compared to VLA-4 or PSGL-1 integrin-targeted MPIO. Dual-PV-MPIO produced strong signal enhancement post-contrast compared to pre-contrast images. Rapid dual PV-MPIO binding to the aortic root endothelium in chow-fed apoE−/− mice was demonstrated by in vivo MRI (11.7 T), with steady state accumulation reached within 30 min of MPIO injection, and maintained at 60 min (Fig. 4). Dual-PV-MPIO tracked closely with the burden and distribution of plaque macrophages, not merely plaque size, at different stages of lesion development. Flow cytometry of aortas confirmed that dual-ligand MPIO binding was predominantly to VCAM-1-positive endothelial cells. En face immunofluorescence microscopy confirmed co-localization of dual-PV-MPIO binding to VCAM-1 positive endothelial cells in the atherosclerosis-susceptible inner curvature of the aortic arch with no MPIO binding observed in the atherosclerosis-resistant outer curvature or descending aorta. MPIO have been shown be sequestered by the liver and spleen, with no evidence of tissue infarction, inflammation, or hemorrhage [95, 96]. Therefore, targeted MPIO offer potential as leukocyte-mimetic agents to track atherosclerotic disease.
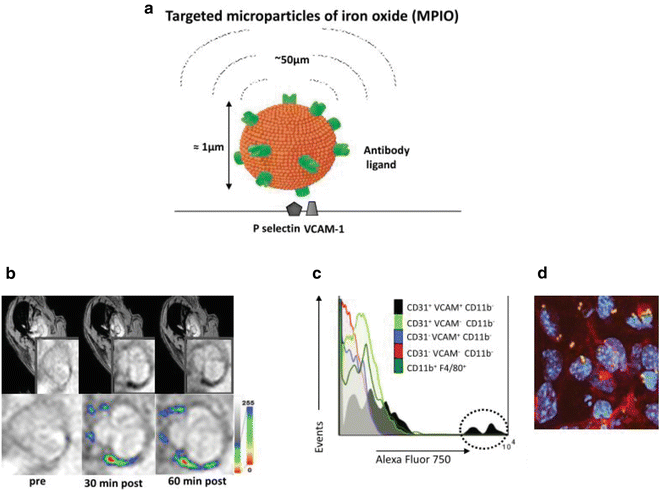
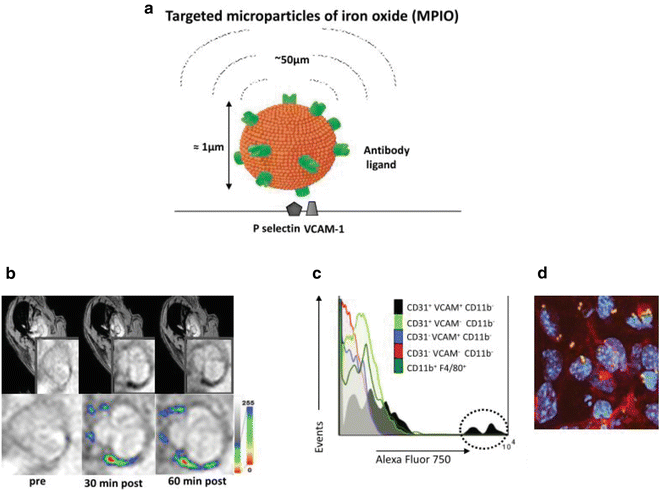
Fig. 4
(a) Schematic of dual-targeted MPIO conjugated with P-selectin and VCAM-1 monoclonal antibodies (PV-MPIO, 1 μm diameter). MPIO produce strong MRI contrast effects which can extend a distance of up to 50 times the diameter of the particle, known as the “MRI contrast blooming effect”. (b) In vivo MRI shows rapid low MR signal intensity areas in the aortic root of chow-fed apoE−/− mice 30 min after PV-MPIO injection, which are maintained at 60 min. (c) Flow cytometry shows predominant binding of near-infrared fluorescently labeled dual-ligand MPIO to activated aortic endothelial cells (CD31+ VCAM+ CD11b−) with minimal signal in macrophages (CD11b+ F4/80+) or other cell types. (d) Dual-ligand MPIO binding localizes to activated endothelial cells in atherosclerosis-susceptible sites of the aortic arch [63]
A similar approach targeting ultrasound microbubbles to vascular endothelium used a combination of sLeX and ICAM-1 antibody, though binding efficiency was increased only marginally, possibly due to stearic limitation from size mismatch between the two ligands [97]. Dual-targeted approaches have also been applied to enhance the detection of vascular inflammation using optical imaging with fluorescent microspheres conjugated to recombinant PSGL-1 and ICAM-1 antibody [98] and photoacoustic imaging with E-selectin and ICAM-1 antibody conjugated gold nanorods [99]. In order to attenuate immunogenicity of monoclonal antibodies, Van Kasteren et al. developed iron oxide glyconanoparticles functionalized with a glycan ligand (sLeX) that binds with high affinity to E and P-selectin [100]. This agent enabled molecular MRI of early endothelial inflammation in mouse models of acute cerebral vascular inflammation, EAE, and focal cerebral stroke. The sLeX sugar ligand has the advantage of transferable cross-species utility that is difficult to achieve with antibodies.
10 Targeted Radioisotope Imaging of Adhesion Molecules
A number of radiolabeled peptides have been developed for molecular PET and SPECT imaging of endothelial adhesion molecule expression in atherosclerosis. Radiolabeled cyclic linear peptide, 18F-4V, has been reported to detect in vivo VCAM-1 expression in atherosclerotic plaques in mice using hybrid PET-CT imaging [101]. Reduced atherosclerotic lesion uptake of 18F-4V was detected in mice treated with atorvastatin, which corresponded to reduced aortic VCAM-1 mRNA expression. The rapid clearance of 18F-4V from the circulation allowed imaging to commence 1 h after 18F-4V administration, with minimal background signal. Radiolabeled nanobodies have also been investigated as potential targeted agents for imaging of atherosclerosis [102, 103]. Nanobodies are single-domain antibody fragments that occur naturally in sharks and camelids. Noninvasive SPECT–CT imaging using 99mTc-radiolabeled VCAM-1 nanobodies enabled detection of VCAM-1 expression in aortic arch atherosclerosis in apoE−/− mice [102]. Fucoidan, a naturally occurring polysaccharide mimetic of sLex derived from brown seaweed, has also been investigated as a targeting ligand for P-selectin. Radiolabeled 99mTc-labeled fucoidan was shown to detect endothelial activation in ischemic myocardium 2 h after reperfusion in rats [104]. However, PET tracers are limited in specificity since they also produce high background signal which may be difficult to distinguish specific contrast.
11 Molecular Imaging of Activated Platelets in Atherosclerois
In addition to endothelial cell inflammation, platelet-endothelial interactions play an important role in the pathogenesis of atherosclerosis. For example, platelet-endothelial interactions mediated by P-selectin have been shown to facilitate the initiation of atherosclerotic lesion formation in mice [105], platelets are involved in the advanced stages of atherosclerotic plaque rupture and microembolism [106], and ruptured atherosclerotic plaques and vulnerable rupture-prone plaques on the vessel wall are lined with activated platelets [107]. Glycoprotein GP IIb/IIIa receptors (CD41/CD61; also known as αIIbβ3 integrin) on activated platelets mediate the final common pathway of platelet aggregation via fibrinogen and are key to thrombus formation [108]. A single-chain antibody that specifically recognizes ligand-induced binding sites (LIBS) on GP IIb/IIIa receptors, which become exposed only upon activation by receptor-ligand binding, has been developed offering the opportunity to target activated platelet adhesion [109, 110]. The LIBS single-chain antibody binds specifically to GP IIb/IIIa on activated platelet aggregates or platelets adherent to damaged endothelium, and does not bind to circulating platelets. However, in contrast to fibrin-rich thrombi, which form complex 3D reticular structures with high abundance of epitope, activated platelet thrombi may be only partially occlusive and localized at the surface of the ruptured atherosclerotic plaque, presenting a challenge to targeted contrast delivery. MPIO (1 μm diameter), conjugated to the histidine tag of LIBS single-chain antibodies (LIBS-MPIO) have been shown to overcome this challenge, enabling detection of activated platelets in a mouse model of endovascular platelet aggregation by ex vivo MRI (11.7 T) [96]. LIBS-MPIO have also allowed in vivo MRI detection of small, platelet-rich thrombi in a mouse model of wall-adherent carotid thrombosis, and reliably detected reductions in thrombus size in response to thrombolysis treatment with urokinase [111, 112]. The potential of LIBS-MPIO for human use has also been investigated in explanted symptomatic carotid artery plaque specimens by ex vivo MRI (9.4 T) [111] and human platelet-rich clots in vitro using a 3 T clinical MRI scanner [112]. MPIO targeting platelet-rich thrombi may also overcome the limitation of a previous in vivo MRI study using USPIO coupled to a cyclic arginine-glycine-aspartic acid (RGD) peptide, in which the in vivo spatial resolution (0.2 × 0.2 × 1 mm) and sensitivity were seen as potentially limiting for clinical application [113]. The use of single-chain antibodies as targeting ligands is attractive for human application since they have low immunogenicity due to a lack of Fc regions. Single-chain antibodies can also be tailored in size and produced in large quantities at low cost [114].
12 Molecular Imaging of Extracellular Matrix Proteins
ECM proteins, such as collagen and elastin, are major components of atherosclerotic lesions [23]. A high abundance of elastin during plaque development and changes in elastin content can provide a quantitative marker of plaque burden and plaque stability. In vivo molecular MRI of elastin was recently confirmed in apoE−/− mice using an elastin-specific small molecular weight Gd-based MR agent (ESMA) [115]. ESMA uptake by atherosclerotic plaques produced strong MRI signal intensity changes, which allowed accurate assessment of plaque burden and characterization by quantification of intra-plaque elastin content. Successful translation into the coronary arteries has been demonstrated in a swine model of coronary injury [116], offering potential for non-invasive assessment of coronary artery wall remodeling and plaque burden in patients with suspected coronary artery disease or in-stent restenosis. Imaging of plaque collagen has also been demonstrated using paramagnetic liposomes functionalized with the collagen adhesion protein CNA35, both in vitro [117] and in vivo in a mouse model of aortic aneurysm [118].
13 Proteolytic Enzymes
Molecular imaging of macrophage products has been demonstrated by targeted imaging of extracellular released enzymes, matrix metalloproteinase (MMP) or myeloperoxidases (MPO). Matrix metalloproteinases (MMPs) produced by macrophages and smooth muscle cells are thought to mediate the progression of stable atherosclerotic lesions to an unstable phenotype that is prone to rupture through the destruction of strength-giving ECM [119–121]. Activatable fluorescent probes, radiolabeled inhibitors, and nanoparticles are being investigated for molecular imaging of protease activity [122]. Recently, a Gd-based agent conjugated to the small MMP targeting peptide P947 has been shown to facilitate discrimination of MMP-rich atherosclerotic plaques compared to MMP-poor plaques by in vivo MRI in apoE−/− mice [123, 124], in hyperlipidemic rabbits [125] and by histologically evaluation of human carotid artery endarterectomy specimens [123]. The plasma half-life of this small peptide was only 30 min and the concentrations in artery specimens were three times as high as those achieved after injection of Gd-DOTA, with prolonged retention at least until 22 h after injection [123]. An MPO targeted Gd-based contrast agent has also enabled targeted imaging of MPO in the aortic wall of New Zealand White rabbits [126] and mice [127, 128]. Focal areas of increased signal intensity due to MPO (Gd) binding, co-localized with myeloperoxidase-rich areas infiltrated by macrophages [126]. MPO-Gd have also been applied for dual PET/MRI imaging of MPO-rich inflammatory leukocyte recruitment following nanoparticle-facilitated, siRNA therapy in atherosclerosis-prone apoE−/− mice after MI. A 75 % decrease in MPO-Gd signal following monocyte subset-targeted siRNA therapy was observed by PET-MRI [129].
14 Summary and Future Directions
Molecular imaging of atherosclerosis promises to provide improved diagnosis and prognosis of atherosclerotic plaques as well as monitoring of therapeutic efficacy. The development of imaging agents with potential for clinical translation is already under way. Small-molecular-weight Gd-based contrast agents can mainly image high abundance targets such as fibrin, elastin, collagen and albumin, while nano-sized particles and radiolabels have shown potential for imaging low-abundance targets or cells such as adhesion molecules and macrophages. MPIO offer a versatile platform for MRI of endothelial cell-specific biomarkers, such as VCAM-1, due to their size, exceptional potent T2* contrast effects, as well as their rapid blood clearance and rapid binding to target. The MPIO used in our studies are non-biodegradable due to their polyurethane coat. However, the basic iron contrast mechanism is potentially transferable to humans with suitable adaptation of the surface coat. Iron-dextran nanoparticles are already in clinical use and the synthesis of biodegradable MPIO is under development [130–133]. In particular, biocompatible MPIO based on a multiple iron oxide nanoparticle platform covalently coupled through a peptide linker and cleavable by intracellular macrophage proteases have shown promise for in vivo MRI of endothelial-specific inflammation [134, 135]. Extensive toxicological profiling and synthesis of sterile, clinical-grade targeted MPIO according to good manufacturing practise (GMP) will be required before clinical translation is feasible. Although targeted nano-sized agents, such as VCAM-1 targeted nanomaterials, lack cellular specificity and bind not only to endothelial cells but also smooth muscle cells and macrophages, these agents may still reveal important biological processes in atherosclerosis, through enhanced detection of inflammatory imaging signals [136]. However, more studies are required to comprehensively investigate the in vivo biological behavior and cellular processing of nano- and micro-materials as well as organ clearance of the core material and its coating.
With the recent approval of gadofosveset, a promising albumin-binding agent for detection of vascular endothelial permeability, and ferumoxytol, a super-paramagnetic ultra small iron oxide particle, and promising phase II results of EP-2104R, a fibrin-binding contrast agent, molecular MRI has entered the clinical arena [137]. The use of high MRI field strengths together with the introduction of multifunctional and multimodal nanoparticles offers great potential for future integration of diagnosis, treatment, and tracking of atherosclerotic plaque responses to therapy.
References
1.
Virmani R, Burke AP, Farb A et al (2006) Pathology of the vulnerable plaque. J Am Coll Cardiol 47:C13–C18PubMed
2.
Falk E, Shah PK, Fuster V (1995) Coronary plaque disruption. Circulation 92:657–671PubMed
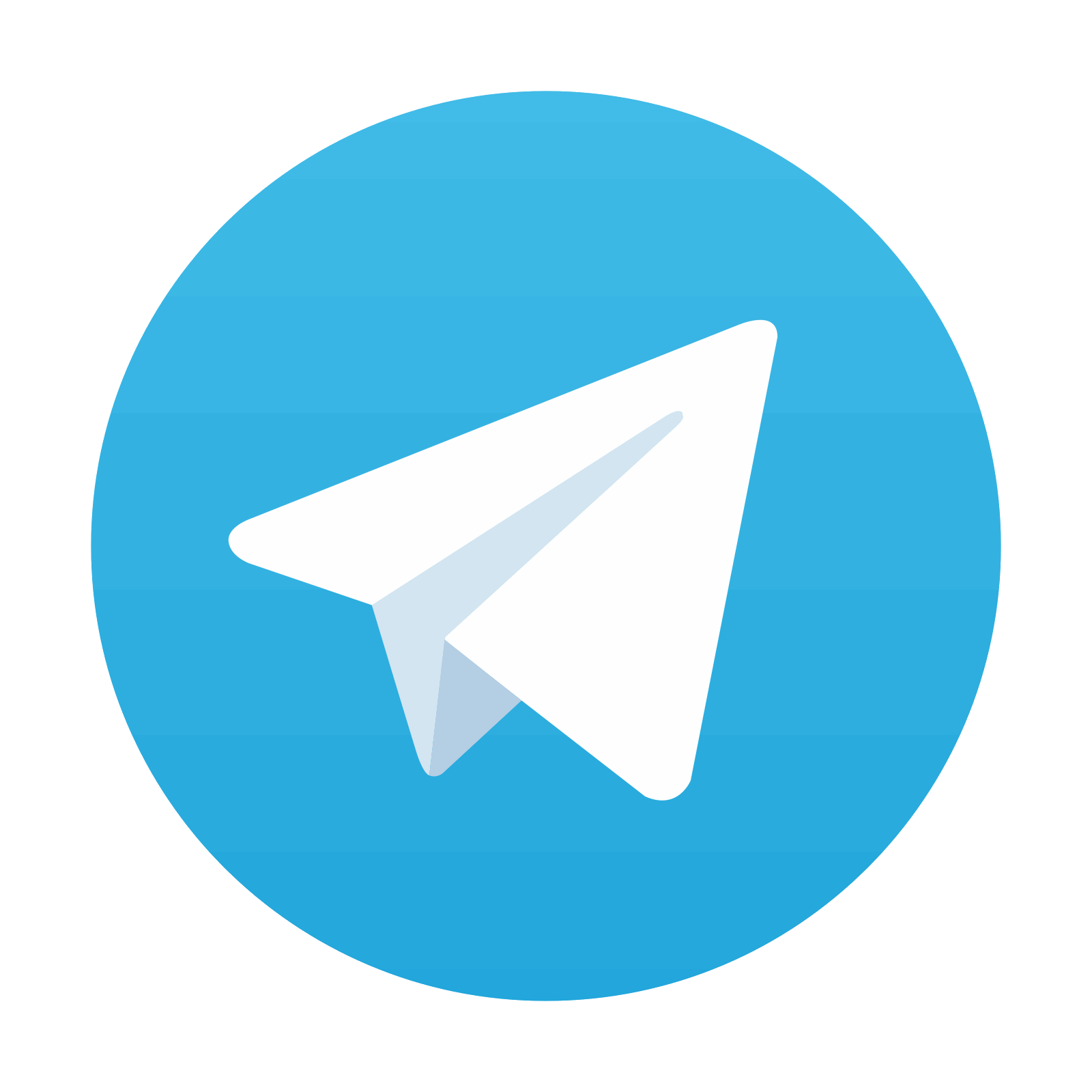
Stay updated, free articles. Join our Telegram channel

Full access? Get Clinical Tree
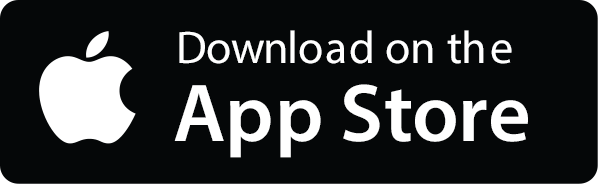
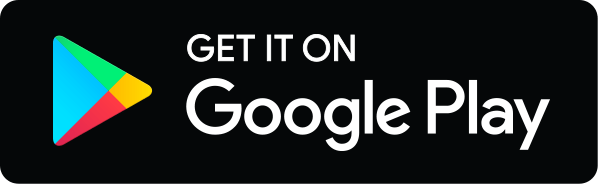