Fig. 11.1
ASPEN interspinous fusion device
11.3.2 BacFuse (Pioneer)
This device is used alone or as an adjunct to interbody fusion and/or posterior fusion with decompression in treatment from T1 to S1. It has a spiked-plate design that provide spinous process fixation. The BacFuse decompresses the spinal canal while supporting the formation of interspinous fusion.
Recently those devices have gone through an evolution, with the creation of expansion devices and cardanic compression devices that allow the distraction and the compression of the segment during the surgical procedure. These new devices allow to model the orientation of the segment toward compression, increasing the pressure on the cage and assuring a better interbody fusion. These are advanced spinous process fixation systems that were developed to address some of the disadvantages of traditional stabilization devices. The implant has unique telescoping plates that allow surgeons to fixate and compress spinous processes to restore sagittal alignment and facilitate a reliable interbody fusion. The device’s large contact area provides a strong anchor point from which is possible to apply compression between the adjacent spinous processes during the surgical procedure, and it offers optimal stability during the fusion process. These systems are easy to use and the quick procedure offers minimal exposure, dissection, muscle trauma, and blood loss as well as protection of neural structures. The devices have a large bone graft window and are intended for use with bone graft material and are not intended for stand-alone use. These devices are designed to support the formation of fusion and decompression by fixation and interspinous process spacing, while renewing anatomic alignment. These implants have an adjustable, fenestrated core and adjustable-length plates which allow for expansion and compression. The in situ compressibility allows surgeons to control the lordosis at the treated level, while the adjustable sizing allows for an optimized anatomical fit. Bone graft material is then packed within the hollow post of the implant.
11.4 Surgical Technique
These devices could be implanted by a minimally invasive approach ; through a very short median skin incision (extended from the upper spinous process to the lower spinous process) of about 3–4 cm, the surgeon must expose the upper and lower lamina of one side of the metamere. Using a specific minimally invasive instrumentation (Fig. 11.2), the device must be implanted between spinous processes using a technique similar to the one used for the insertion of a classic interspinous spacers. After radiological intraoperative check, the device must be blocked in the desired position. After this, in the interspinous space, using a specific instrument, a cruentation of the bone must be performed and bone chips could be inserted (Fig. 11.3). The mean duration of the surgical intervention is 40 min, and the blood loss is extremely poor. It is important to underline that a TLIF technique could be added to this device with the aim of obtaining a 360° fusion with only a monolateral minimally invasive approach.



Fig. 11.2
Intraoperative view of low profile instruments for an IFD implant (a) minimally invasive instrument (b) device which has to be implanted

Fig. 11.3
Intraoperative view of the surgical technique: (a) exposure of the surgical field, (b) preparation and enlargement of the interspinous space, (c) cruentation and bone exposure of the interspinous space, (d) positioning of the interspinous fusion device in the interspinous space
11.5 Biomechanics of IFDs
If we consider that interspinous devices IPD can be implanted in stenosis of mild and moderate degree, being the stenosis central or foraminal, or in the low-grade spondylolisthesis without spondylolysis (with poor or at least controversial results), we give for granted that the degenerative lumbar cascade, as described by Kirkaldy-Willis, is in the active phase. The degenerative lumbar spondylosis in active phase has as a first step the damage of the intervertebral disk, whose degree of degeneration is related to the entity of the damage and to the persistency of the damage itself in time. Normally, the biomechanic behavior of the lumbar spine is subject to the rule of spine loading. According to this rule, the axial load of the body is discharged, and consequently neutralized, on the intervertebral disk and on the posterior structures (articulations, ligaments, and muscles) in proportions of 80% and 20%, respectively. Any disk degeneration transfers the axial load to the posterior elements of the spine, determining an inversion in the distribution of the axial load, related to the loss of viscoelastic and shock adsorber properties of the disk itself. This condition promotes the insurgence of a functional overload of the facet joints, determining a greater mechanical stress than the physiological one, with consequent hyperlaxity of the facet joints, reduced competence of the articular capsule and hypermobility of the lumbar segment. The hypermobility stimulates the inflammatory reaction in the adjacent tissues, activating chemokines (fractalkine in particular) in the ligamentum flavum, promoting the chemotaxis in the ligamentum itself. The inflammatory cells cause extracellular matrix degradation of the ligamentum, determining loss of elasticity and hypertrophy. It is well documented the role of fractalkine in the development of numerous inflammatory diseases (rheumatoid arthritis, dermatitis, etc.) and in ligaments and joints involved in inflammatory processes caused by instability (e.g., joint capsules, ligaments, and synovium). The inflammatory process involves these tissues so the fractalkine overexpression is activated; thus causing the recruitment of mononuclear cells within the LF feeding the inflammation and causing vascular injury and angiogenesis. Moreover such an increase in mononuclear activity causes a proliferation of fibroblasts (for overexpression of TGF beta mRNA resulting in increased collagen fibers) and inflammatory cells in LF. This inflammatory cell activity in the LF causes rupture of the extracellular matrix (for activation of metalloproteinase MMP2) due to the elastin degradation, resulting in loss of elasticity of the ligament and subsequent hypertrophy. The collapse of the intervertebral disk causes ligamentum flavum redundancy, and its prominence in the vertebral canal reduces the diameter of the canal itself, determining spinal stenosis. Only in this phase, the articular hypertrophy generates foraminal stenosis, the collapse of the disk generates ligamentous stenosis, and the stenosis becomes symptomatic, but the main pathological substrate remains the hypermobility. The treatment of a hard or soft stenosis has necessarily to be strictly linked to the concept of vertebral instability as basic pathological condition. The introduction in the market of fusion interspinous devices is relatively recent, so there are less studies regarding the biomechanical effects of these devices mainly focused on the ROM reduction compared to pedicle screw constructs. Kettler et al. performed a biomechanical in vitro study on a different version of the Coflex interspinous implant, called Coflex Rivet , in which the device is screw-fixed to the spinous processes. The new device was tested for flexibility and load transfer, and, unlike the original Coflex implant, it is shown to increase stability only in extension as described in other biomechanical studies. Compared to the defect condition (bilateral hemifacetectomy with resection of the flaval ligaments), both implants had a strong stabilizing effect in extension. Also Coflex Rivet strongly stabilized in flexion and was able to compensate the destabilizing effect of the defect in axial rotation and lateral bending. The authors believed that the biomechanical characteristics of this new implant might even make it suitable as an adjunct to fusion, which would be a new indication for this type of device. Wang et al. conducted a biomechanical study on the CD HORIZON SPIRE fixation system. The authors compared the stability provided by the SPIRE with unilateral and bilateral pedicle screw system in destabilized spines with or without anterior allograft support. Used alone, or in conjunction with an interbody cage, the SPIRE provided a great stability in flexion and extension and the limitation of motion appears to be equal to bilateral pedicle screw system. In lateral bending and axial rotation, the SPIRE had a less stabilizing effect, and it reduced motion equal to unilateral pedicle screw system. In the recent biomechanical study conducted by Karahalios et al. [7], the ASPEN device was compared with other devices standing alone and in conjunction with anterior lumbar interbody fusion (ALIF) procedure . The authors found that the stand-alone ASPEN device decreased significantly the ROM in extension and flexion with less effects on the ROM in lateral bending and axial rotation. The use of ASPEN device and ALIF had a stabilization effect immobilized equal to ALIF and pedicle screw system and superior to ALIF and anterior plate system. The authors concluded that ASPEN device could be an alternative implant to pedicle screw system and anterior plate system when used in conjunction with ALIF. The use of the ASPEN device resulted in flexion at the index level, with a resultant increase in foraminal height. Compensatory extension at the adjacent levels prevented any significant change in overall sagittal balance. Kaibara et al. [8] conducted a biomechanical study on ASPEN interspinous fixation device in combination with transforaminal lumbar interbody fusion (TLIF) and other posterior fixations in human cadaver spines. The use of the stand-alone ASPEN device significantly reduced motion in flexion and extension, and the outcomes were similar to the effects obtained with the use of TLIF and bilateral pedicle screw system. In lateral bending and axial rotation, ASPEN device with and without TLIF showed inferior stability to bilateral pedicel screw. TLIF supplemented with ASPEN device and unilateral screw system provided equal stability as in TLIF with bilateral pedicle screws. The authors suggested the ASPEN device as a possible alternative to pedicle screw systems. In 2013, Techy et al. conducted a biomechanical study to evaluate the effect of the use ASPEN device as augmentation of an interbody cage or a pedicular screw fixation. After implantation of the ASPEN device to augment the interbody cage, there was a significant decrease in the ROM of 74% in flexion-extension (FE), but there was no significant change in lateral bending (LB) and axial rotation (AR). The construct with unilateral pedicle screws showed a significant reduction of FE by 77%, LB by 55%, and AR by 42% compared with control spine. The bilateral pedicle screw construct reduced FE by 77%, LB by 77%, and AR by 65% when compared with the control spine. The authors concluded that ASPEN device, which is used to augment an interbody cage, was able to provide FE stability comparable with the bilateral pedicle screw fixation. However, it provided minimal stability in LB and AR unless further augmented with pedicle screws [9–14]. Similar results were obtained by the study published by Gonzalez-Blohm et al. in 2014. In this study, the authors evaluated the biomechanical performance of the ASPEN as a stand-alone device after lumbar decompression surgery and as supplemental fixation in a posterior lumbar interbody fusion (PLIF ) construct. They suggested that the ASPEN device may be a suitable device to provide a flexion-extension balance after a unilateral laminotomy. PLIF constructs with ASPEN device and pedicle screw fixation performed equivalently in flexion-extension and axial rotation, but the PLIF-bilateral pedicle screw construct was more resistant to lateral bending. The authors recommended further biomechanical and clinical evidence to strongly support the use of this interspinous fusion device as stand-alone or as supplemental fixation to expandable posterior interbody cages.
On the basis of these comparative studies, some observations are mandatory:
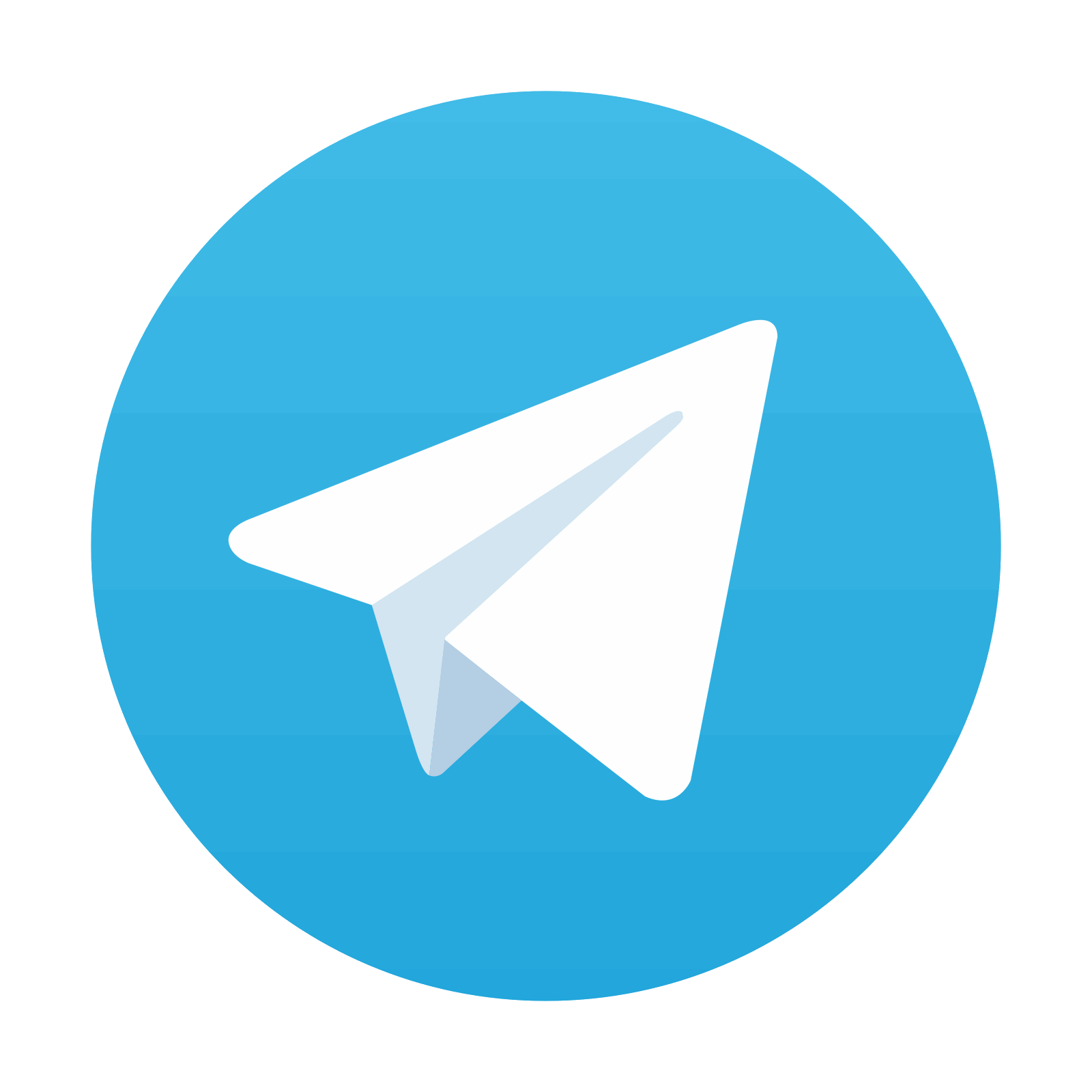
Stay updated, free articles. Join our Telegram channel

Full access? Get Clinical Tree
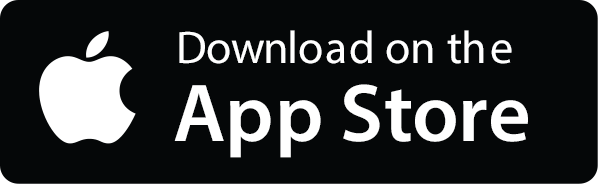
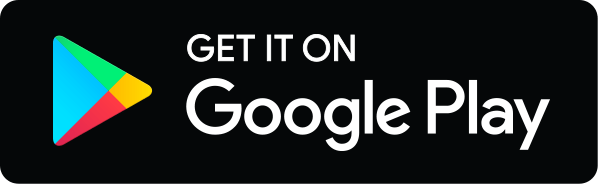