4 Neurophysiology of the Joints and Muscles The past 30 to 50 years have witnessed constantly increasing interest by physicians in manual medicine approaches. At the same time there has been an equal amount of skepticism and overt criticism of the reported, primarily empirical studies, since definitive basic and clinical research was lacking. For a long time more hypothetical postulates than real science had been proposed in an attempt to explain the possible mechanisms of action that were thought to underlie the different manual medicine techniques. Despite recent notable progress and intensive international efforts with better scientific designs and implementation of solid research protocols, little is still known today about the specific neurophysiologic processes or specific biochemical or biomechanical mechanisms that are initiated with or follow manipulation or mobilization maneuvers applied to the joints of the spine and the limbs. One of the major initial steps was taken in 1975, when upon the recommendation of the National Institutes of Health, the National Institute of Neurological and Communicative Disorders and Stroke (Goldstein, 1975) held a conference on the topic “The Research Status of Spinal Manipulative Therapy.” References here are mainly to Sato’s (1975) work on the somatosympathetic reflexes, Perl’s (1975) work on pain and spinal and peripheral nerve factors, and White and Panjabi’s (1990) fundamental work on the biomechanics of the spine. In 1978, a symposium was held in Pisa, Italy on “Reflex Control of Posture and Movement” (Granit and Pompeiano, 1979). Even though manual medicine per se was not discussed, this symposium offered a broad basis of the theoretical research in this field up until that time. In 1982, the College of Osteopathic Medicine at Michigan State University held a symposium entitled “Empirical Approaches to the Validation of Manual Medicine” (Greenman and Buerger, 1984). Here, researchers and clinicians from the fields of biomechanics and neurophysiology discussed their basic and clinical research findings in relation to the field of manual medicine. Based on his basic experimental research with Freeman (Freeman and Wyke, 1967), Wyke coined the term “articular neurology.” Wyke’s publications from 1967, 1975 (together with Polacek), 1979, and 1980, and the information presented by Jayson (1980) expand on this term and thus provide a basis for a better understanding of the neurophysiologic processes that are potentially involved in manual medicine. These will now be described in more detail. The following presentation is based mainly on the fundamental work by Wyke and co-workers. Articular neurology can be defined as that branch of neurology that deals with the morphology, physiology, pathology, and clinical aspects of the innervation of the joints of the entire skeleton and the limbs. Within this chapter, we will concentrate primarily, but not exclusively, on the apophyseal joints, which are also referred to in the literature as the zygapophyseal (“Z-joints”) or facet joints; in this text the terms will be used interchangeably. Wyke’s major contribution to the field of articular neurology was the identification of the structures and innervation patterns of the four major joint receptor types, as well as their distribution and characteristic physiologic behavior. Furthermore, Wyke’s work has shown that these receptors can be found in all synovial joint capsules, and that they have an effect on static and dynamic reflex control mechanisms of the skeletal muscles in response to normal and pathologic conditions. It is now accepted that the human synovial joints contain three types of mechanoreceptors (nociceptive-free) and the free nerve endings—the nociceptors. Their organization was visualized in neurohistologic studies (Wyke and Polacek, 1973, 1975; Freeman and Wyke, 1967; Vrettos and Wyke, 1979). The mechanoreceptors are proprioceptors and can be further subdivided into three specific groups (types I, II, and III), while the nociceptors make up the fourth group (type IV). They are depicted in Figures 4.1 and 4.2 and are described in more detail below. The type I mechanoreceptors are found primarily in the outer layers of the fibrous joint capsule. These receptors consist of three to eight globular corpuscles measuring approximately 100 μm by 40 μm. Thinly encapsulated and thinly myelinated afferent nerve fibers (6–9 μm) connect these corpuscles with the corresponding articular branches of the dorsal rami of the spinal nerves. Fig. 4.1 Schematic representation of the four joint receptors. The type I, II, and III receptors are mechanoreceptors. Type IV receptors are nociceptors (Polacek, 1966). Fig. 4.2 Location of the various joint receptor types demonstrated for the knee joint. (After Freeman and Wyke, 1967.) • Slowly adapting receptors: they control tension of the outer layers of the joint capsule. • Transsynaptic inhibition of the centripetal flow of the activity of the nociceptive afferent receptors (type IV nociceptors); in other words, inhibition of the impulses arising from pain receptors. • Tonic reflexogenic effects on the motor neurons of the neck, limb, jaw, and eye muscles (Wyke 1975, 1977; Molina et al., 1976; Biemond and DeJong, 1969; Igarashi et al., 1972; Hikosaka and Maeda, 1973; DeJong et al., 1977). The type II receptors consist of oblong, conical, thickly encapsulated corpuscles (about 280 μm by 100 μm) that most often appear singly in the deeper layers of the fibrous joint capsule. These receptors are connected to the articular rami by way of thickly myelinated nerve fibers. • Rapidly adapting mechanoreceptors with a low threshold reacting to changes in tension of the fibrous joint capsule (less than 0.5 seconds). • Phasic and reflexogenic effects on neck, limb, jaw, and eye muscles. • Transitory inhibition of the nociceptive activity of the joint capsule. Mechanoreceptors of the type III category play a significant role in joint function. The type III receptors are typically embedded in the ligaments and the terminal portion of the tendons close to the joint capsule. They are not found in the joint capsule itself. Morphologically, they consist of broad, fusiform corpuscles (600 μm by 100 μm) and usually appear singly. Occasionally, they occur in clusters of one to three and are found at the end of a ligament or tendon. The type III receptors are innervated by large myelinated (13–17 μm) fibers that are connected to the articular branches. The shape of the type III receptors resembles that of the Golgi tendon organs. It is postulated that the type III mechanoreceptors may have a function similar to that of the Golgi tendon organs. Furthermore, it is believed that these slowly adapting receptors have an inhibitory reflexogenic effect on motor neurons (Freeman and Wyke, 1967). • Inhibitory reflexogenic effects on motor neurons. • Function similar to the Golgi tendon organ. Nociceptive fibers are sensitive to noxious substances in the tissue. Nociceptors are free, very thinly myelinated or nonmyelinated plexiform nerve endings. They are ubiquitous in the fibrous portion of the joint capsule. This receptor system is activated by depolarization of the nerve fibers. For instance, depolarization occurs with constant pressure on the joint capsule as would be the case with nonphysiological position or as is seen with abrupt, jerky, uncontrolled movement. They are set off by such tissue alterations as disk narrowing or compression, acute vertebral fracture, or a dislocation of the apophyseal joints. In addition to mechanical factors, the nociceptors can also be stimulated by chemical irritation via such substances as potassium ions, lactic acid, 5-hydroxytryptamine, and histamine, among others. Interstitial edema of the joint capsule, often associated with acute or chronic inflammatory processes, is also known to activate the fibers, resulting in pain. • Pain generation. • Tonic reflexogenic effects on neck, limb, jaw, and eye muscles. • Respiratory and cardiovascular reflexogenic effects. The joint is innervated by the dorsal rami of the associated spinal nerves. It should be emphasized that the articular ramus of a nerve root contributes not only to one segmental joint capsule: its collateral rami above and below also supply the neighboring joints as well. Therefore, the joint capsules are innervated bisegmentally or even multisegmentally (Auteroche, 1983). The cross-sectional representation at the level of the thoracic spine is depicted in Fig. 4.3. This depiction demonstrates the course of the nerve as it supplies the corresponding joints, tendons, paravertebral muscles, and the periosteum. Fig. 4.3a, b The nerve supply of the joints, muscles, ligaments, and periosteum in the thoracic spine region. (After Wyke, 1967.) a Innervation of the vertebrae and disks, lateral view. Note that typically each facet joint receives its innervation of at least two neighboring branches. b Cross-sectional schema of the localized vertebral, disk and facet joint innervation as demonstrated in a thoracic vertebra. Note that typically the posterior primary ramus innervates the paravertebral muscles of the back, whereas the anterior primary ramus innervates the extremities. Note the relationship of the spinal ganglion with reference to the facet joint posteriorly and the costovertebral joint anterolaterally. 1 Apophyseal joint 2 Costovertebral joint 3 Costotransverse joint 4 Spinal ganglion 5 Anterior ramus of the spinal nerve (left) 6 Posterior ramus of the spinal nerve (left) 7 Anterior longitudinal ligament 8 Posterior longitudinal ligament 9 Paravertebral muscles 10 Interspinous ligament 11 Ventral nerve root of the spinal nerve 12 Dorsal nerve root of the spinal nerve Fig. 4.3c Innervation of the disk with emphasis on the sinuvertebral nerve (Haldemann et al., 2002). The nociceptive and mechanoreceptive fibers enter the posterior horn in the gray matter of the spinal cord via the dorsal root. Once in the spinal cord, the nociceptive fibers divide into a number of collateral rami. Some of these rami extend through the gray matter directly to the basal nuclei (basal spinal nucleus or laminae IV and V after Rexed). From there, they run through the lateral spinothalamic tract (anterolateral portion) to ultimately terminate in the limbic system, where the actual perception of pain occurs. Pain cannot be subjectively perceived unless depolarization of the synapse has occurred at the level of the basal nuclei following the initial nociceptive impulses. Similarly to the nociceptive afferent fibers, the mechanoreceptive afferent fibers enter the posterior horn of the gray matter of the spinal cord through the dorsal root (Fig. 4.4). It is important for this theory that some of the many divided rami form synapses at the level of the apical spinal nucleus or lamina II (after Rexed). The apical spinal interneurons arising from this level conduct the activity of the mechanoreceptive afferent stimuli to the basal spinal nucleus, where they are thought to inhibit presynaptically the pain-inducing nociceptive neurons. Thus, they interrupt conduction to the spinothalamic tract and the limbic system (Wyke, 1979a,b; Bonica and Albe-Fessard, 1980). Experiments on adult cats demonstrate that a portion of the thinly myelinated afferent fibers end in the substantia gelatinosa of the dorsal horns. The transmission of nociceptive impulses—as initiated by peripheral stimulation of the nociceptors, for instance—can be blocked by application of opioids to the substantia gelatinosa. Transmission of other sensory modalities, such as that of light touch, remains intact, however (Yoshimura and North, 1983). It is possible that opioids inhibit those cells of the substantia gelatinosa that function as interneurons in the transmission of pain. Electron-microscopic studies showed an endogenous enkephalin in the substantia gelatinosa (Benett et al., 1982), which again would support the idea of the interneurons performing an inhibitory function. The enkephalins have been surmised to function as inhibiting neurotransmitters. It also appears that strong stimulation of the type II mechanoreceptors can lead to a depotlike increase of the enkephalins in the dorsal horns (Wyke, personal communication, 1983). As outlined earlier in this chapter, one of the major contributions to the current understanding and the basis for further research of the clinically observed nonradicular pain syndromes, and the spondylogenic reflex syndrome (SRS: see Chapter6) inparticular, came from Wyke and his co-workers. This experimental work is of particular relevance for clinical practice and for our current understanding of the various proposed mechanisms. The joint capsule and the articular nerve of C3–C4 were microsurgically exposed in anesthetized cats. The joint capsule was irritated using a probe and a stimulator. A substantially lower voltage was necessary to stimulate the mechanoreceptors (2 V) than to stimulate the nociceptors (8 V). Wyke’s results, as reproduced here, very clearly show the reflex relationships between the receptors of the fibrous joint capsule, the apophyseal (facet) joints, and the peripheral musculature (Figs. 4.5–4.8). Fig. 4.4 Arrangement of the fibers of the mechanoreceptors and nociceptors in dorsal horn of the spinal cord. (After Wyke, 1979a.) Fig. 4.5 The reflexogenic mechanoreceptor effects of a cervical apophyseal joint on the muscles of the neck. At the location indicated by the arrow, a single articular nerve of the left C3–C4 apophyseal joint was stimulated for 3 seconds with an electrical impulse (2 V, 5 Hz, 5 ms) that selectively excited the mechanoreceptive afferent fibers in the exposed nerve. The simultaneous electromyographic tracings show a long-lasting effect on the homologous pairs of the neck muscles. (After Wyke, 1979a.) Akio Sato (1975) made similar observations in his investigation of the somatosympathetic reflexes. A reflex type of relationship between soft-tissue changes or segmental (i. e., somatic) dysfunctions and diseases of internal organs has been suggested on the basis of clinical observations (Beal, 1984; Beal and Dvořák, 1984; Larson, 1976). Perl (1975) partially explains the phenomenon of referred pain by this reflex mechanism. Wyke (1979b) suspects that multisynaptic intraspinal tracts may be involved, which both ascend to the brainstem and descend to the basal nuclei of the lower levels of the spinal cord. The free nerve endings associated with the nociceptorsare found in the joint capsules, muscles (Mense and Meyer, 1985), the intervertebral disks (Bodgduk, 1993), and the dorsal nerve root ganglia. They are all sensitive to pressure. A sensory volley up to 25 minutes after compression of the ganglion was detected. The ganglion may be a significant source of a patient’s pain, especially when considering its close proximity to the disk and in particular when there is a significant disk herniation (e. g., prolapse, protrusion). The dorsal nerve root itself does not appear to be particularly pressure sensitive unless there is concomitant inflammation or irritation. In a rabbit model, sensory discharge lasting up to several minutes after induced compression of the nerve root by the autologous nucleus pulposus was observed. Fig. 4.6 The same experimental arrangement as in Fig. 4.5, except with a stimulus of 8 volts, 5 hertz, 5 milliseconds, in which both the afferent fibers of the mechanoreceptors and nociceptors were stimulated. (After Wyke 1979a.) Fig. 4.7 Reflex effects of the mechanoreceptors of the cervical apophyseal joints on limb muscles. At the signal (S), a single, exposed branch of the articular nerve was repetitively stimulated for 3 seconds, selectively exciting the mechanoreceptor afferent fibers in the corresponding nerve. The simultaneous electromyographic tracings of the upper and lower limb muscles display the reflexogenic effects of varying duration. They demonstrate that such stimulation has an affect not only on the muscles of the neck but also on the muscles of the extremities. (see also Fig. 4.5). Interestingly, stimulation that generates a nociceptive impulse produces a different muscle potential response. (After Wyke 1979a.) Fig. 4.8 The reflex effects of an induced manipulative procedure directed at the C3–C4 articulation. At the signal, brisk vertical cervical traction procedure was applied to the isolated joint. The simultaneous electromyographic tracings of the homologous pairs of the upper and lower limb muscles display the reflex effects of such cervical manipulation on the peripheral musculature. (After Wyke, 1979a.) It has been postulated that the nucleus pulposus may contain chemical substances that, when released from the disk after a tear, for instance, may induce inflammatory neurodegenerative reactions. Furthermore, it was thought that in the acute phase these chemical irritants are excitatory rather than inhibitory. Olmarker and Rydevik (1993) believe that the immunoglobulin G or stromolysin may be one of the chemical irritants. These observations may, at least in part, explain the unabated pain reported by a significant number of patients, even when decompressive spinal surgery had been deemed successful after nerve root compression, for instance. Human skeletal muscle is characterized by a high degree of plasticity. This plasticity is accomplished by a wide array of different muscle fiber types, which allows the various muscles to respond to the many different functional requirements placed upon them (Pette, 1990). This explains, at least in part, the muscles’ abilities to respond to different demands. On the one hand, a person is able to perform high-speed activities of short duration such as a hundred-meter dash, while on the other the same person is able to run at slower speeds over long duration as is required in a marathon. The ability to develop sufficient strength for activities such as lifting a 200-kilogram weight is yet another aspect of the muscles’ functional plasticities to respond to different demands. Flück and Hoppeler (2003) present a state-of-the-art review of muscle structure and function in which they describe a muscle’s plasticity in response to various stimuli as well as developing a paradigm for studying gene regulatory phenomena in humans. The following section on muscle fibers is based on the excellent review presented by Weber (1995), which is reproduced here with kind permission. Muscle fibers are categorized according to their biochemical, histochemical, mechanical, and metabolic characteristics (Barnard et al., 1971; Dubowitz, 1985; Cumming et al., 1994). Until quite recently, striated muscles had simply been classified according to their primary metabolic activity as either aerobic or anaerobic muscle, or red and white muscle, respectively. Recent research, however, indicated that further subclassifications was necessary, especially for the “white” fibers, which are more accurately known as “type II” fibers. While the literature had reported “intermediate” muscle fibers that appear to share properties of both the aerobic and anaerobic fiber types, specific staining techniques have allowed separation of the various fibers according to their particular metabolic and contractile properties. Despite the advent of more detailed histochemical profiles, this has also led to a confusing nomenclature for the different types of muscle fibers (Cumming et al., 1994). The salient characteristics are listed in Table 4.1. The primary categorization distinguishes between the slow-twitch, oxidative, fatigue-resistant type I fibers, and the fast-twitch, glycolytic type II fibers, which are further subdivided according to their fatigability. The type IIA fibers are the fast-twitch, fatigue-resistant, glycolytic fibers, while the type IIB fibers are also fast-twitch and glycolytic but fast-fatiguing fibers. Recent studies seem to indicate that human type IIB fibers may correspond to the II-x fibers described for animals (Ennion et al., 1994; Smerdu et al., 1994), and the nomenclature has recently changed accordingly. • Aerobic type I muscle fibers:
Neuropathophysiology of the Apophyseal Joints
Articular Neurology
Receptors of the Joint Capsule
Type I Receptors (Mechanoreceptors)
Function
Type ll Receptors (Mechanoreceptors)
Function
Type III Receptors (Mechanoreceptors)
Function
Type IV Receptors (Nociceptors)
Function
Innervation of the Joint Capsule
Central Interactions of the Mechanoreceptors and Nociceptive Impulses
Mechanoreceptors and Nociceptive Reflexes
Possible Neural Mechanisms Involved in Back Pain
Functional Pathology of Muscle
Morphology and Function of the Slow-Twitch (Type I) and Fast-Twitch Muscle Fibers (Type II)
Human Skeletal Muscle Fiber Types
General Properties
Stay updated, free articles. Join our Telegram channel

Full access? Get Clinical Tree
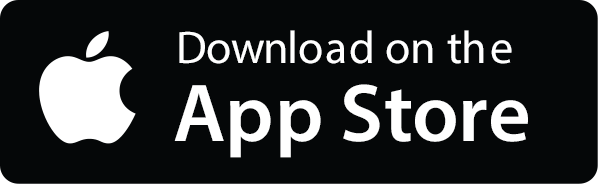
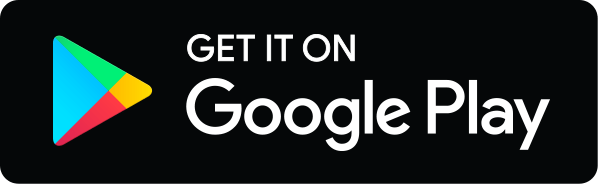