Class of nerve injury
Myelin
Axon
Endoneurium
Perineurium
Epineurium
Electrophysiology
MRN findings
Sensory nerve action potential
Compound motor action potential
Electromyography
I- Neurapraxia
Abnormal
Normal
Normal
Normal
Normal
Normal
Normal or conduction block
Normal but interference pattern decreased
Hyperintense nerve
II- Axonotmesis
Abnormal
Abnormal
Normal
Normal
Normal
Amplitude decreased
Amplitude decreased
interference pattern and spontaneous activity decreased
Hyperintense and thickened nerve with/without prominent fascicles
III
Abnormal
Abnormal
Abnormal
Normal
Normal
IV
Abnormal
Abnormal
Abnormal
Abnormal
Normal
Heterogeneous nerve signal with lateral or fusiform neuroma in continuity
V-Neurotmesis
Abnormal
Abnormal
Abnormal
Abnormal
Abnormal
Absent
Absent
No motor unit potentials
Complete nerve gap
Nerve Compression and Entrapment Neuropathies
Nerve compression typically occurs at specific anatomic sites leading to what is termed an entrapment neuropathy [9]. Typically, these are chronic conditions. Classic examples for nerve compression or entrapment syndromes are carpal tunnel syndrome and cubital tunnel syndrome. However, compression neuropathies may occur at many more sites or tunnels in the body including the pelvic floor region, the popliteal space, the tarsal tunnel, the dorsal foot, the thoracic outlet, the infraclavicular region, quadrilateral space, the elbow, the forearm, the canal of Guyon, etc. It is beyond the scope of this syllabus to explain these lesions in detail, but the most important upper extremity entrapment syndromes e.g. have been described in the 2013 IDKD syllabus book as well as other good review articles [10–13].
External nerve compression blocks the normal flow of endoneurium fluid. In addition, compression of the venous flow occurs which typically leads to vascular congestion and local hyperemia at the compression side and proximal to it. This is important to understand as abnormalities on magnetic resonance neurography images will show correspondingly signal changes of the nerve at the side of compression and proximal to it. The pathophysiology of entrapment syndromes is different from acute nerve pathologies, as the development of compressive neuropathy depends mostly on long-term or repetitive pressure within these tunnels and the fact that nerves typically can withstand only little external pressure. At more than 15 mmHg, the venous drainage of the nerves is increasingly hampered, and at over 40 mmHg the arterial blood supply is affected. Irreversible structural nerve damage begins at pressures of around 80 mmHg [3]. In patients where the external compression continues for a longer period, there is a loss of myelin with associated risk of permanent axonal damage, Wallerian degeneration, nerve infarction and nerve fibrosis [14].
Spontaneous nerve healing in chronic nerve compression or entrapment syndromes is very unlikely. However, with surgery, good results and nerve recovery can be achieved, i.e. when the diagnosis is made prior to chronic and often irreversible nerve changes [15]. The latter is characterized by fat and/or fibrous tissue proliferation around the affected axons.
Nerve Regeneration
Nerve regeneration usually proceeds slowly from the anterior horn cells of the spinal cord (the location of the main cell body of a peripheral nerve) to the neuromuscular endplate a rate of 1 mm per day or approximately 1 in. per month. Therefore, depending on the length of the nerve fibers, it is easy to calculate the likely recovery time. For “spontaneous” recovery of long extremity nerves, it may take up to 1–1½ years. As a practical example, it may take up to 2 years to return full motor function to the distal forearm after a brachial plexus injury [16].
It must be also noted that functional nerve recovery does not occur in all pathologies. There can be a persistent lack of full sensory and/or motor function; there is typically no guarantee that even with primarily successful surgical therapy that normal function will be restored. The latter can be seen especially in chronic pathologies or hereditary neuropathies such as Charcot-Marie-Tooth disease (CMT) where either formation of “axon-deficient myelin ovoid” in axonal degeneration (CMT II) or “repeated myelination-related onion-bulb formation” (CMT I) is observed.
Technical Considerations and Normal Appearance of Nerves
For successful magnetic resonance neurography, the most practical advice may be the following: “simply use the best equipment which you have available”. This includes the magnet, the radiofrequency coils, the pulse sequences and the software [17].
Scanner
Magnetic resonance neurography can be performed on 1.5 and 3.0 Tesla clinical scanners. When available, 3.0 Tesla is preferred due to the increased signal-to-noise ratio and superior contrast-to-noise ratio at higher spatial resolution. Especially 3-dimensional (3D) isotropic imaging benefits from higher field strength and shorter acquisition times. With high-end field strength, some drawbacks may arise such as increased B0 inhomogeneities that might cause poor fat suppression when spectral saturation pulses are used. Therefore, the use of STIR (short tau inversion recovery) or Dixon type fat suppression may be beneficial at 3.0 Tesla [17]. Another concern at 3.0 Tesla is the increased specific absorption rate, which might cause longer examination times. Of course, technical tricks exist to address these latter limitations such as reducing the refocusing flip angle to shortened acquisition time (e.g. 130° instead of 150°). However, despite some drawbacks, most experts favor the use of 3.0 Tesla scanners.
Coils
Whenever possible, the use dedicated multichannel surface coils is advised. These should be—as always in magnetic resonance imaging—be placed as close as possible to the anatomy of interest. While seemingly simple, this can be quite challenging when imaging several anatomic regions needing different coils or if a larger field-of-view needs to be covered. Some scanners allow combined use of multiple coils, whereas with other scanners, coils need to be changed during the study.. Dedicated joint coils can also be used in combination with flex or surface coils in some scanners which, for example, allows the use of high-resolution wrist coils to examine the median nerve in the wrist in combination with a flex coil that covers the forearm. This combination allows seamless examination of the median nerve in the forearm, wrist and hand at the same time. If a specific field-of-view is still too large to be covered even with the use of coil combination, then a stepwise-approach, first using nerve ultrasound to narrow down the possible site of injury and with subsequent dedicated magnetic resonance imaging of that remaining nerve section may be helpful. Along these lines, it is important that referring physicians understand the tradeoffs between spatial resolution and anatomic coverage in magnetic resonance neurography. It is almost always preferable to scan a small region in high detail than to try to survey a large amount of anatomy for possible nerve pathology.
Training of the technician/technologist is an often forgotten but nevertheless extremely important issue. Technicians should also be taught to identify target anatomic structures (they should be able to identify the nerve-of-interest); to correctly position patients (e.g. the region-of-interest should be placed in the scanners isocenter whenever possible); and to correctly plan pulse sequences (e.g. to avoid wraparound artifacts).
Sequences
Much has been written about pulse sequence developments for magnetic resonance neurography and several new techniques have been published including variations of 3D FSE (fast spin echo) acquisitions. The latter are based on technical advancements and are available as product sequences (e.g. Siemens; SPACE; Philips: VISTA, Views; and GE: Cube) [4]. The basic principle of the sequences when used and optimized for magnetic resonance neurography is to reduce the refocusing flip angles to decrease the sensitivity to flow. When assessing images, it is obvious that these sequences adequately suppress the arterial signal, however, venous signal contamination remains a frequent problem due to slow flow. Hence, these 3D FSE sequences are often combined with either a weak gradient pulse to reduce venous signal or with motion sensitizing driven equilibrium (MSDE) for further reduction of the venous flow signal. For example, SHINKEI (nerve-SHeath signal increased with INKed rest-tissue RARE Imaging) is a 3D FSE sequence variant with SPAIR for fat suppression and MSDE for blood suppression [18]. These 3D sequences are especially helpful for brachial and/or lumbosacral plexus imaging [19].
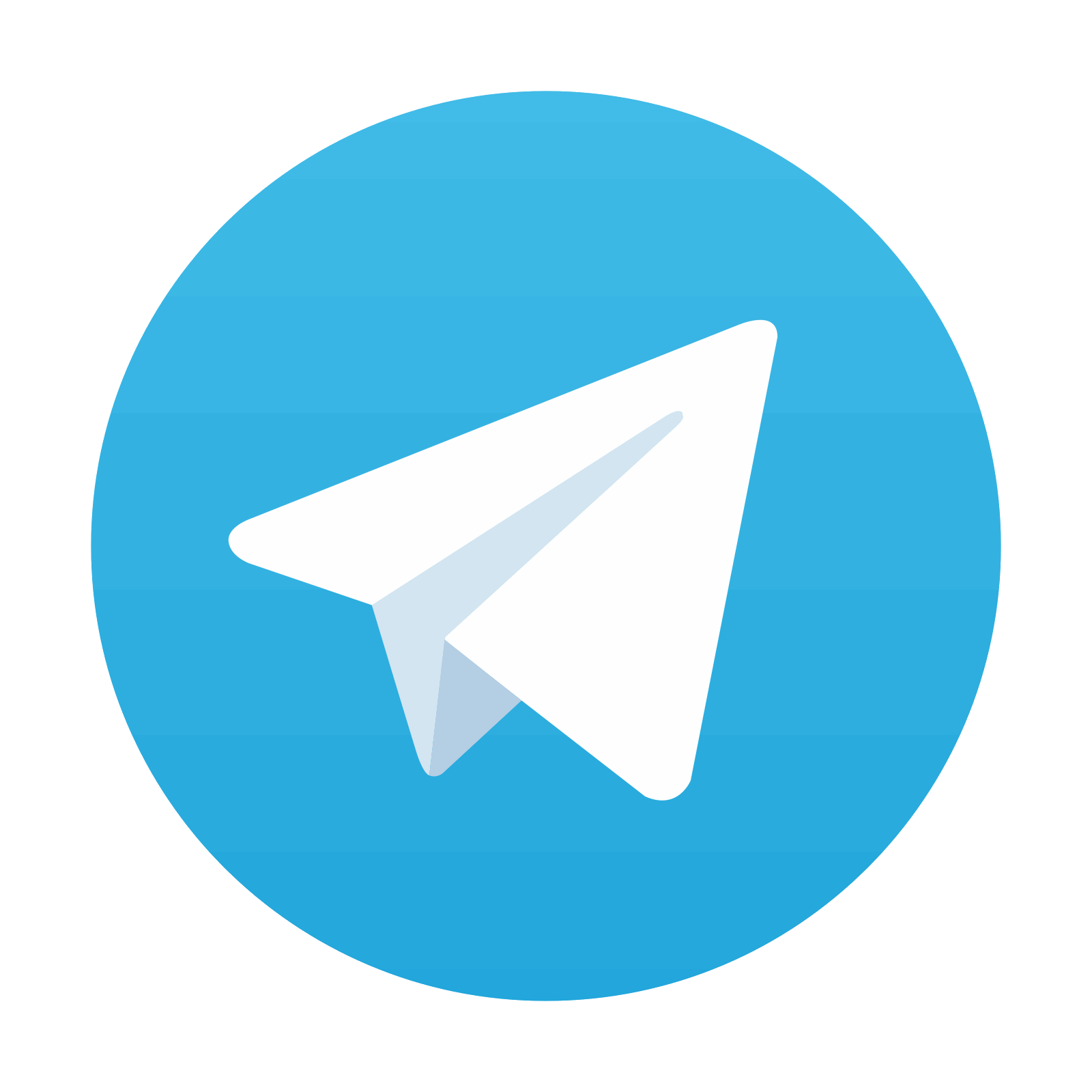
Stay updated, free articles. Join our Telegram channel

Full access? Get Clinical Tree
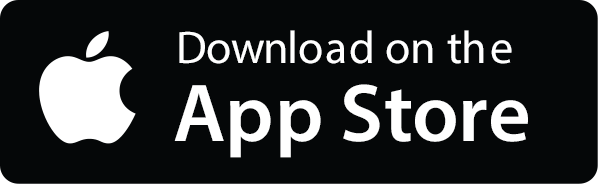
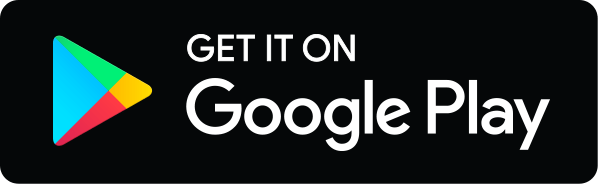