Myopathies and Nerve Disorders
Ranjan Gupta, MD
Jacques H. Hacquebord, MD
Dr. Gupta or an immediate family member serves as a board member, owner, officer, or committee member of the American Shoulder and Elbow Surgeons and the American Society for Surgery of the Hand. Dr. Hacquebord or an immediate family member has received research or institutional support from Acumed, LLC.
ABSTRACT
Myopathies and nerve disorders continue to remain challenging diagnoses. The past several years, however, have seen significant progress in both elucidating the pathophysiology and advancing treatment particularly in muscle disorders. Investigation into muscle-derived stem cells (MDSCs), specifically MDSC transplantation, shows much potential for future use. Gene replacement therapy has also allowed for significant advancement in the treatment of muscular dystrophies along with medications such as eteplirsen and eculizumab, which have recently been approved for use in various muscle disorders. In the setting of nerve injury, understanding the difference in pathophysiology between acute and chronic nerve injuries is critical in directing treatment and further research.
Keywords: Becker muscular dystrophy; Duchenne muscular dystrophy; myopathy; nerve healing; nerve injury; neuropathy
Introduction
Myopathies and nerve disorders produce significant morbidity and remain challenging to treat. Advancements in our understanding of the pathophysiology have been made, but there remains significant need for a more complete understanding to develop meaningful therapies. This chapter includes our updated understanding of both the pathophysiology for common myopathies and nerve disorders and current therapeutic avenues.
Myopathies
Muscle Anatomy of Physiology
Human skeletal muscle develops force and allows for movement. Muscle comprises numerous muscle fibers arranged in a tubular structure, which function as the individual contractile units within muscles. Each muscle fiber consists of numerous tubular myofibrils. The myofibrils have repeating sections of sarcomeres. Sarcomeres give muscle its striated appearance and are the contractile subunit of muscle. Sarcomeres each contain rows of myosin overlapping with an actin framework. The length of the sarcomere where the myosin and actin framework overlap is directly related to the force capable to be generated by the muscle. This relationship between muscle length and active force is displayed in the Blix curve, which describes the relationships between the active force generated by muscle, passive force of muscle, and muscle length. The Blix curve identifies the sarcomere length(s) at which the muscle is biomechanically in the ideal position with optimal myosin and actin overlap to generate the maximum active force (Figure 1). Muscle phenotype is also controlled by several different signaling pathways. These include the Ras/MAP signaling pathway, calcineurin, Ca2+/calmodulin-dependent protein kinase, and peroxisome proliferator γ coactivator 1. These pathways are exercise-induced and important in determining the specialized characteristics of slow-twitch and fast-twitch muscles. Skeletal muscle is often divided into slow-twitch (type 1) and fast-twitch (type 2). This distinction originates from the fact that chemical energy converted to mechanical work occurs either through anaerobic glycolysis or from oxidation of pyruvate or free fatty acids. The oxidative enzymes are localized inside mitochondria, whereas the enzymes of anaerobic glycolysis are not membrane-bound. The efficiency of anaerobic glycolysis is comparatively low; however, it has the advantage of not being impaired by lack of blood supply. Most skeletal muscle has the ability to shift between both metabolic pathways, and most muscle fibers are specialized and differ in their content of enzymes and substrates of metabolism. The
muscles that are slower twitching tend to have high oxidative capacity, whereas the fast-twitch muscles rely on anaerobic glycolysis.
muscles that are slower twitching tend to have high oxidative capacity, whereas the fast-twitch muscles rely on anaerobic glycolysis.
Muscle Injury Pathophysiology
Injuries to skeletal muscle involving degradation of myofibers and fibrosis and fatty cell infiltration of muscle can occur through (1) mechanical weakening of the sarcolemma, (2) inappropriate calcium influx, (3) aberrant cell signaling, (4) increased oxidative stress, and (5) recurrent muscle ischemia. The exact mechanisms by which this happens are quite complex but involve the dystrophin-glycoprotein complex. It was previously believed that there was one unifying mechanism, but it is now believed that the dystrophin-glycoprotein complex is involved in multiple different pathological pathways that result in skeletal muscle damage. Injury of skeletal muscle can also involve conversion of the muscle to bone. This can occur in myositis ossificans secondary to significant trauma or in fibrodysplasia ossificans secondary to a genetic mutation. The mechanism by which this occurs is debated; however, it is believed that most heterotopic bone originates through endothelial mesenchymal transition1 and that smooth muscle cells do not contribute to any stage of heterotopic ossification.2
Another common degradation pathway for muscle is the loss of tendon attachment to bone, that is,, the enthesis with the ensuing changes in muscle physiology and structure. A common clinical scenario for these injuries includes rotator cuff tears. Tendon tears result in loss of muscle tensile forces, leading to decrease in muscle strength and atrophy both radially and longitudinally.3 There is decreased sarcomere number and length, which results in an overall appearance of myofiber disorganization.4,5 These changes result in increased fat content and fibrosis. The exact cause for fatty infiltration remains poorly understood.6 The two primary progenitor cells that appear to be the source of fatty infiltration are adipose-derived mesenchymal stem cells (MSCs) and muscle-derived stem cells (MDSCs).7,8,9 Muscular atrophy can recover, but it has been well demonstrated that fatty infiltration does not. Some studies support the theory that massive tear results in a neurologic injury through which fatty degeneration occurs as a result of denervation of the affected muscle. Other studies have postulated that the chronic unloading of the muscle-tendon unit leads to atrophy and fatty degeneration through infiltration of adipocytes. A final hypothesis is that the loss of tensile strength in muscle directly leads to phenotypic changes. This theory has been supported by molecular studies using animal models where loss of mechanical stretch in skeletal muscle results in the upregulation of specific adipogenic (PPARγ and C/EBPβ) and myogenic (Myf-5) transcription factors and the suppression of specific pathways (Wnt signaling) that then result in the upregulation of adipogenic transcription factors.10,11
Muscle-Derived
Stem Cells MDSCs were first discovered in 1961 when Alexander Mauro detected a clump of mononucleated cells in adult myofiber using electron microscopy.12 Physically, MDSCs reside between the basal lamina and sarcolemma of adult muscle fibers.13 Like typical adult stem cells, they possess the ability to proliferate and self-renew. The unique marker profile of MDSCs include Pax7/pax3, Myf5, CD56, and CD29.14,15 Pax3 and Pax7 are transcription factors, which help to regulate myogenic differentiation by activating the expression of myogenic differentiation genes Myf5 and MyoD. CD56 is also expressed by myoblasts and neuronal-derived tissues, among others. CD29, also known as β1-integrin functions mainly as a collagen receptor but has been shown to be upregulated in MDSCs. Partly because of the ability to differentiate into adipocytes, osteocytes, myocytes, and chondrocytes, MDSCs have a high regenerative capacity in musculoskeletal tissue healing.16 Transplanted MDSCs can vigorously self-renew, expanding and repopulating the host muscle. Collins et al confirmed that as few as seven MDSCs associated with one transplanted myofiber can generate over 100 new myofibers containing thousands of myonuclei. Injury or muscle damage is the most common stimulus for MDSCs to differentiate. They play a role in musculoskeletal tissue regeneration and can fuse with injured
myofibers.17 The fact that they are reversibly quiescent and are capable of self-renewal and differentiation after transplantation makes MDSCs very unique.18 There is still much to learn regarding the role of MDSCs in muscle injury, but future avenues for the use of MDSCs include therapeutic treatment of neuromuscular diseases via transplantation and/or forced differentiation. Specifically, MDSC protein activators could be manipulated to aid in myofiber regeneration following massive rotator cuff tears. Although an alternative strategy is MDSC transplantation to improve postsurgical rotator cuff retear rates, there are currently no human data about what actually happens to MDSCs secondary to injury or aging.
myofibers.17 The fact that they are reversibly quiescent and are capable of self-renewal and differentiation after transplantation makes MDSCs very unique.18 There is still much to learn regarding the role of MDSCs in muscle injury, but future avenues for the use of MDSCs include therapeutic treatment of neuromuscular diseases via transplantation and/or forced differentiation. Specifically, MDSC protein activators could be manipulated to aid in myofiber regeneration following massive rotator cuff tears. Although an alternative strategy is MDSC transplantation to improve postsurgical rotator cuff retear rates, there are currently no human data about what actually happens to MDSCs secondary to injury or aging.
Muscle Disorders
Duchenne and Becker Muscular Dystrophy
Duchenne and Becker muscular dystrophy (DMD and BMD) both occur because of a mutation affecting the DMD gene that encodes for the dystrophin protein. DMD is more severe and also three times more common than BMD. Because this gene is X-linked, it nearly always affects boys. The dystrophin protein has a unique three-dimensional structure that allows for binding to other proteins such as dystrobrevin and syntrophin and as such plays a critical role as a structural linker for other proteins. Because the deformational forces generated by muscle contraction are significant, dystrophin is essential to maintain the integrity of the muscle membrane. However, with translation of a dysfunctional dystrophin protein in the settings of both DMD and BMD, the muscle membrane is damaged, leading to elevations of creatine kinase in the serum and to calcium influx within the muscle fiber, which activates calcium-dependent proteases. In BMD, dystrophin protein is still partially functional due to “in-frame” mutations that allow for continuation of the open reading frame and translation of the protein. However, DMD causes an “out of frame” mutation that leads to ablation of the open reading frame and translation termination. Muscle biopsy was the mainstay of diagnosis for both DMD and BMD. However, molecular mutation analysis has improved and has increasingly become the primary means of diagnosis. Molecular therapies that allow for continued translation of the dystrophin gene are currently in clinical trials.
Many therapeutic interventions focus on modifying dystrophin DNA. Gene editing has potential as a more permanent treatment as permanent chromosomal DNA corrections can be made. Gene replacement therapy primarily utilizes a viral vector delivery system. Successful gene-replacement therapy for DMD requires widespread and efficient delivery of the therapeutic genes to all muscles. Because of the enormous size of the dystrophin gene (˜11.5 kb) and the requirement to replace the gene distributed throughout the body, this has presented significant challenges for viral vector packaging. The adeno-associated virus (AAV) vectors have been found most appropriate to achieve this goal.19,20 However, the dystrophin gene is too large to fit into a recombinant AAV (rAAV), which typically has packaging capacity of 5 kb. Therefore, a reduced-size dystrophin version that leads to a mild phenotype has been developed. One of the challenges has been to identify the appropriate microgene configurations along with AAV serotypes. Preclinical data show significant promise of intravascular AAV microdystrophin delivery in significantly ameliorating the pathology. Although underpowered to reliably evaluate efficacy, several early clinical trials evaluating the safety and tolerability of the gene therapy have shown significant clinical promise.21,22 The continued advancements in animal trials to improve intravascular delivery have led to several current trials in the United States and Europe investigating specific AAV mircodystrophin therapy for DMD and spinal muscular atrophy patients. This is a very exciting development in the field.
Other than gene therapy, other therapies focus on transcription and translation of RNA. More specifically, these include nonsense-suppressing agents that induce translational machinery to “read-through” the premature stop codon, antisense oligonucleotides that “skip” over mutation-containing exons, and upregulation of proteins homologous to dystrophin. In 2016, eteplirsen was approved by the FDA for use in DMD. This medication treats DMD by splicing out one of the mutations, which then allows for correct downstream reading frame and translation. Based on the success of these trials, trials for other congenital muscle disorders including MTM and Pompe are under way.
Myasthenia Gravis
Myasthenia gravis (MG) is the most frequently acquired disorder affecting neuromuscular transmission. The most common etiology includes pathogenic antibodies against components of the postsynaptic muscle end plate membrane. MG is usually divided into subgroups based on clinical features and serum autoantibodies. Most patients have autoantibodies against the nicotinic acetylcholine receptor (AChR). Other common autoantibodies are against muscle-specific kinase and low-density lipoprotein-related protein 4. Knowing the serum autoantibodies is relevant to determine treatment. Traditionally, therapies have been divided into
(1) short-term symptom control (acetylcholinesterase inhibitors, ephedrine, and/or 3,4-diaminopyridine, which work to increase the amount of acetylcholine present at the neuromuscular junction [NMJ]) (2) immunosuppressive, and (3) immunomodulating and supportive, including thymectomy. More specific antigenic targets are emerging in MG but require greater study. Increasingly, immunological subclassification of the disease is important because future therapies will target cell-surface antigens with specific monoclonal antibodies. These targeted monoclonal antibody agents remain some of the more exciting potential emerging therapies. A recent phase 3 randomized double-blinded trial with eculizumab, a recombinant humanized monoclonal antibody to complement, has shown some promise. Although the MG-ADL score was not statistically different between the two groups, the eculizumab group had nearly a third of MG exacerbations as compared with the placebo group.23 Eculizumab has recently been approved by the US FDA as an approved therapy for MG. Along with acetylcholinesterase inhibitor, they are the only therapies for MG that are not considered “off-label” by the US FDA.24
(1) short-term symptom control (acetylcholinesterase inhibitors, ephedrine, and/or 3,4-diaminopyridine, which work to increase the amount of acetylcholine present at the neuromuscular junction [NMJ]) (2) immunosuppressive, and (3) immunomodulating and supportive, including thymectomy. More specific antigenic targets are emerging in MG but require greater study. Increasingly, immunological subclassification of the disease is important because future therapies will target cell-surface antigens with specific monoclonal antibodies. These targeted monoclonal antibody agents remain some of the more exciting potential emerging therapies. A recent phase 3 randomized double-blinded trial with eculizumab, a recombinant humanized monoclonal antibody to complement, has shown some promise. Although the MG-ADL score was not statistically different between the two groups, the eculizumab group had nearly a third of MG exacerbations as compared with the placebo group.23 Eculizumab has recently been approved by the US FDA as an approved therapy for MG. Along with acetylcholinesterase inhibitor, they are the only therapies for MG that are not considered “off-label” by the US FDA.24
Nerve Disorders
Anatomy
Peripheral nerves are heterogeneous composite structures comprising multiple different components including neurons, Schwann cells, fibroblasts, and macrophages. The neuron forms the foundation of the nerve and is a polarized structure consisting of an axon, cell body, and dendrites. The axon projects toward the site of innervation to form a synapse with the target end-organ. The Schwann cells are glial cells that produce myelin to encapsulate the axon and allow for faster and more efficient conduction of the action potential down the axon. Neural blood supply is intricate and forms from anastomoses of epineural, perineural, and endoneural plexuses along with segmental supply from nutrient arteries.25 This blood supply is fragile and is easily injured in the setting of trauma, compression, or with inappropriate repair.26
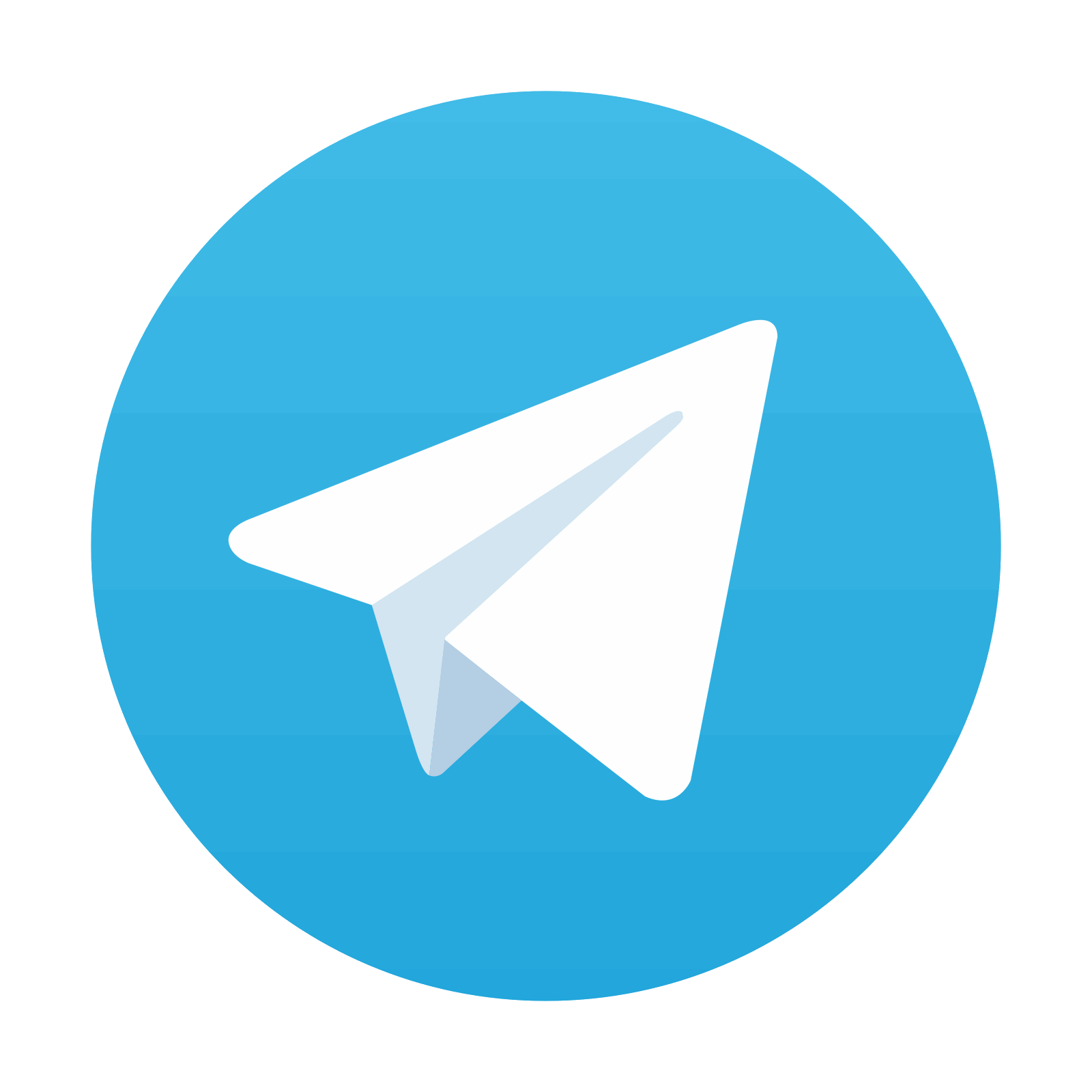
Stay updated, free articles. Join our Telegram channel

Full access? Get Clinical Tree
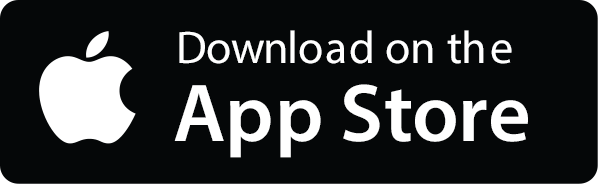
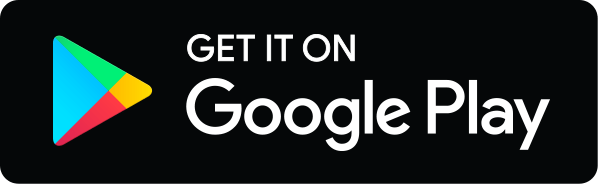