Monoclonal gammopathy of undetermined significance (MGUS)a
(a) Serum monoclonal protein <3 g/dL
And
(b) Bone marrow clonal plasma cells <10 %
And
(c) Absence of end-organ damage attributable to the underlying plasma cell disorder (CRAB criteria, hyperCalcemia, Renal insufficiency, Anemia, and Bone lesions)
Smoldering multiple myeloma (SMM)
(a) Serum monoclonal protein (IgG or IgA)a ≥3 g/dL
And/or
(b) Bone marrow clonal plasma cells ≥10 %,
And
(c) Absence of end-organ damage attributable to the underlying plasma cell disorder (CRAB criteria, hyperCalcemia, Renal insufficiency, Anemia, and Bone lesions)
Multiple myeloma (MM)
(a) Bone marrow clonal plasma cells ≥10 % or biopsy proven plasmacytoma
And
(b) Evidence of end-organ damage attributable to the underlying plasma cell disorder specifically
Hypercalcemia (serum calcium >11.5 mg/dL)
OrRenal insufficiency (serum creatinine >2 mg/dL or estimated creatinine clearance less than 40 mL/min)
Or
Anemia (normochromic, normocytic anemia with hemoglobin >2 g/dL below the lower limit of normal or <10 g/dL)
Or
Bone lesions, lytic lesions, severe osteopenia, or pathologic fractures
Pathogenesis of Bone Disease in MGUS and MM
Altered Cortical Bone Architecture and Reduced Strength Increases Fracture Risk in MGUS
Although by definition patients with MGUS have no osteolytic lesions, population based studies demonstrate an increased fracture risk in MGUS patients compared to their matched controls [16, 17]. Low lumbar bone mineral density is a major risk factor associated with significantly high fracture risk in this patient population [18]. The exact pathophysiology of increased fracture risk in MGUS patients is not well established. Intriguingly, using a high-resolution peripheral quantitative computed tomography imaging of the distal radius, patients with MGUS were shown to have significantly increased cortical bone porosity and reduced bone strength [19, 20]. In addition, serum markers for both increased osteoclastic and decreased osteoblastic activities were significantly elevated in patients with MGUS. Dickkopf-related protein (DKK1), a secreted Wnt pathway inhibitor is a critical mediator of bone disease in myeloma through inhibition of Wnt-regulated osteoblastic differentiation [21]. Importantly, DKK1 is also implicated in increased osteoclastic activity through upregulation of RANKL and inhibition of OPG secretion [22]. Notably, one study showed significantly elevated serum DKK1 levels in MGUS patients compared to matched control subjects [20], although the increase was statistically insignificant in another study [23]. Finally, patients MGUS have significantly high serum level of osteoclast stimulating cytokine, macrophage inflammatory protein-1α (MPP-1α), when compared to matched healthy controls [20]. In conclusion, the altered bone microstructure and an imbalance between bone resorption and formation seem to be responsible for increased fracture risk even in patients with MGUS.
Altered Bone Remodeling Explains the Osteolytic Bone Disease in MM
Bone disease in MM is a major cause of morbidity leading to poor quality of life. Hypercalcemia and skeletal related events such as bone pain, vertebral compression fractures, and pathologic fractures occur due to osteolytic bone disease in a large proportion of patients. Notably, pathologic fractures negatively impact the survival with 20 % increase in the risk of death compared to patients without fractures [24, 25]. Under normal circumstances, continuous turn over and remodeling of adult skeletal occurs through a highly regulated network of interactions between bone microenvironment and the bone cells creating a balance between bone resorption by osteoclasts and formation by osteoblasts. Deregulated bone remodeling due to increased osteoclastic activity and decreased osteoblastic differentiation is the principle cause of excessive bone resorption in MM. The increased osteoclastic activity and the resultant bone resorption typically occur in the areas adjacent to the malignant plasma cells [26]. Moreover, histomorphometric studies also demonstrated an increase in the number of osteoclasts and osteoblasts early in the course of myeloma development [27]. Clonal plasma cells and other elements of bone marrow (BM) microenvironment cooperate with each other through a complex network of signaling factors and cytokines that ultimately result in uncoupling of osteoclastic and osteoblastic activities. A variety of cellular and non-cellular components of BM microenvironment including BM cells (clonal plasma cells, stromal cells, immune cells); extracellular matrix containing collagen, laminin, fibronectin, and extracellular fluid rich in cytokines and other growth factors contribute to the pathogenesis of osteolytic bone disease [28].
Osteoclasts are hematopoietic in origin derived from differentiation and fusion of the monocyte-macrophage lineage precursor cells to form inactive osteoclasts. Activated osteoclasts are responsible for bone resorption and eventually undergo apoptosis [29]. In contrast, osteoblasts arise from mesenchymal stem cells and factors such as platelet-derived growth factor, fibroblast growth factor, and transforming growth factor B enhance their growth and differentiation. Bone microenvironment plays a critical role in the formation of osteoclasts through macrophage colony-stimulating factor and receptor activator of nuclear factor-kB (RANK) ligand (RANKL) produced by the osteoblasts and stromal cells [30]. Osteoblasts and stromal cells have surface expression of RANKL, which bind to the RANK receptor on the osteoclast precursor cells and induce the osteoclast formation [31]. In contrast, osteoprotegerin (OPG), a decoy soluble receptor of RANKL, normally present in the bone marrow binds to the RANKL inhibiting the RANK-RANKL interactions, thereby limiting osteoclastogenesis [32]. The ratio of OPG/RANKL regulates the osteoclast development, and the unbalance of this ratio is associated with bone disease in MM.
The other unique feature of bone disease in MM is the absence of new bone formation in the areas of osteolysis unlike any other tumor metastasis. Importantly, lack of new bone formation due to decreased osteoblastic differentiation and activity explain the pure lytic bone lesions, a typical finding in MM unlike other solid tumor metastases [31]. The key factor involved in this mechanism is DKK1 produced by clonal plasma cells which inhibits Wnt regulated osteoblastic differentiation [21]. This would also explain the persistence of lytic lesions in MM patients in remission despite the absence of increased plasma cells in the bone marrow. In addition to RANKL and DKK-1, several other key factors are involved in uncoupling of osteoclastic and osteoblastic activities including interleukin-3 (IL-3), Interleukin-6 (IL-6), MIP-1a, MIP-1B, BAFF, and activin A, and a detailed discussion is beyond the scope of this chapter. In conclusion, uncoupling of osteoclastic and osteoblastic activities is responsible for the formation of bone lytic lesions that are typical of MM. Understanding the mechanisms involved in this process has led to the development of several therapeutic targets for myeloma bone disease.
Clinical Presentation and Diagnostic Work Up
The clinical manifestations of MM occur due to bone marrow infiltration by clonal plasma cells, and the related monoclonal paraprotein resulting in end organ damage. It is important to distinguish MM from its preceding disorders such as MGUS or SMM. The hallmark of MM and other plasma cell dyscrasias is the presence of monoclonal protein (M-protein) produced by the clonal plasma cells. The key distinguishing feature of MM from MGUS and SMM, is the presence of end organ damage, commonly referred to as CRAB, which include hyperCalcemia, Renal insufficiency, Anemia and Bone lesions [12] (Table 9.1). Most commonly MM patients present with fatigue due to anemia, bone pain, elevated creatinine, symptoms related to hypercalcemia, and weight loss [6]. Recurrent infections due to impaired cellular immunity and hypogammaglobulinemia are a common feature. Extramedullary plasmacytomas (EMP) can be seen in 7 % of patients at diagnosis and an additional 6 % during the course of the disease, and are associated with overall poor survival [33].
In addition to the routine laboratory studies including complete blood count, differential count, evaluation of blood smear, and comprehensive metabolic profile, initial evaluation for suspected MM should include serum and urine protein electrophoresis and immunofixation (Fig. 9.1). Urine 24-h collection is necessary for identification and quantification of monoclonal immunoglobulin or free light chains, referred to as Bence Jones proteins. A small percentage (~3 %) of patients does not have a detectable M-protein either in the serum or urine, and are referred to as non-secretory myeloma. However, the majority of these patients have elevated serum free kappa or lambda light chains that can be measured with serum free light chain assays [34]. Therefore, the incidence of truly non-secretory myeloma, as defined by the absence of M-protein in the serum, and urine with normal serum free light chain ratio, is quite low.


Fig. 9.1
(a) Normal serum protein electrophoresis. (b) Immunofixation electrophoresis shows polyclonal immunoglobulins with and monoclonal paraprotein. (c) Serum protein electrophoresis from a patient with multiple myeloma shows a monoclonal spike in the gamma region (dashed area). (d) Immunofixation electrophoresis identified the monoclonal protein as immunoglobulin G, lambda type. (G immunoglobulin G, A immunoglobulin A, M Immunoglobulin M, K kappa light chain, L lambda light chain)
Bone marrow evaluation is indicated in all patients with suspected MM including immunophenotyping, karyotype, and fluorescent in situ hybridization (FISH) using probes for chromosomal abnormalities of prognostic significance. A gene expression profile testing is also of prognostic significance and may identify high-risk patients [35].
The current International Myeloma Working Group (IMWG) guidelines recommend whole body X-ray skeletal survey as the gold standard test for evaluation of MM bone disease [36]. However, PET/CT and MRI scans are much more sensitive in detection of the number of bone lesions in MM with an ability to identify extramedullary plasmacytomas, the identification of which has a significant prognostic impact [37]. Advanced imaging studies should be considered in all patients with bone pain and normal skeletal survey or neurological symptoms suggestive of cord compression (Table 9.2). Routine use of PET/CT and MRI scan in MM is not currently recommended, although these advanced imaging modalities are being incorporated in management of MM.
Table 9.2
Initial diagnostic work up for suspected plasma cell dyscrasia
• Complete blood count with differential count |
• Peripheral blood smear evaluation |
• Complete metabolic profile |
• Serum protein electrophoresis with immunofixation |
• Urine protein electrophoresis with immunofixation |
• Serum free kappa and lambda light chainsa |
• Serum beta-2 microglobulin |
• Bone marrow aspirate and biopsy |
• Chromosome analysis (karyotyping), bone marrow |
• Fluorescent in situ hybridization (FISH), bone marrow |
• Plain X-ray skeletal survey |
Staging and Risk Stratification
The clinical course of MM is widely heterogeneous with a long indolent course in some patients while others have a rapidly progressive fatal disease. A variety of host factors, including the age, disease burden, severity of end organ damage and biological characteristics, influence the clinical course of MM. There are two staging systems that are widely accepted for staging and assessment of tumor burden in MM (Table 9.3). The Durie–Salmon staging system (DSS) , developed many years ago based on mathematical calculation of plasma cell tumor mass in association with the severity of the end organ damage (Calcium level, Renal insufficiency, Anemia and Bone lesions) and immunoglobulin level is still widely used [38]. The main limitation of this system is the potential for subjective errors in evaluating lytic bone lesions and also the lack of prognostic significance. The International staging system (ISS) , a simple and more practical system was developed based on retrospective analysis of a large number of previously untreated MM patients utilizing two widely available laboratory tests, serum β-2 microglobulin and albumin levels. The ISS has prognostic significance with a median survival of 62, 44, 29 months in patients with stages I, II, and III respectively [39]. Although the ISS is more easily reproducible in clinical trials, it does not effectively reflect the tumor burden as the level of albumin and β-2 microglobulin can be affected by the renal function and other comorbidities. In addition, the prognostic role of ISS has not been validated in the era of novel biological therapeutic agents [40]. Neither staging systems are currently utilized in treatment decision-making.
Table 9.3
Staging for multiple myeloma
Durie–Salmon staging [38] | Stage I: Low cell mass: <0.6 × 1012 cells/m2 and all of the following: (a) Hemoglobin >10 g/dL (b) Serum IgG <5 g/dL and (c) Serum IgA <3 g/dL (d) Normal serum calcium (e) Urine monoclonal protein excretion <4 g/day (f) No generalized lytic bone lesions Stage II: Intermediate cell mass: neither stage I nor stage III Stage III: High cell mass: >1.2 × 1012 cells/m2 and one or more of the following: (a) Hemoglobin <8.5 g/dL (b) Serum IgG >7 g/dL and (c) Serum IgA >5 g/dL (d) Serum calcium >12 mg/dL (e) Urine monoclonal protein excretion >12 g/day (f) Advanced lytic bone lesion a Stage IIIA Serum creatinine <2 mg/dL and IIIB with serum creatinine ≥2 mg/dL |
Stage I: B2M <3.5 mg/L and serum albumin ≥3 g/dL Stage II: neither stage I nor stage III Stage III: B2M ≥5.5 mg/L |
In addition to the tumor burden as indicated by ISS and the DSS systems, host factors such as advanced age, ECOG performance status of 3 or 4 and renal failure (serum creatinine >2 mg/dL) are associated with adverse prognosis and less intensive treatment is recommended for those patients [41]. Finally disease biological characteristics play a critical role in determining patient prognosis and therapeutic strategies. Foremost are chromosomal abnormalities identified by conventional cytogenetics and by FISH, which allow risk stratification into standard-risk, intermediate risk and high-risk disease according to the Mayo stratification for myeloma and risk-adapted therapy classification (mSMART) [42]. Chromosomal translocations, t(14;16), t(14;20), and 17p deletion, typically identified by FISH, impart poor prognosis and are considered high-risk. The adverse prognostic impact of t(4;14) can be overcome by incorporating Bortezomib in the frontline treatment regimen, and therefore considered as intermediate risk category. All other chromosomal abnormalities, including hyperdiploidy and translocations t(11;14), t(6;14), are considered standard risk. The median overall survival in high risk MM is approximately 3 years in comparison to 8–10 years in standard risk patients. Treatment should be tailored to the individual risk category to minimize the toxicity and improve the overall clinical outcomes.
Risk-Adapted Therapy for Newly Diagnosed Patients
The first question to consider for newly diagnosed is whether patients are candidates or not for intensive chemotherapy followed by autologous stem cell transplantation (ASCT). Age and concomitant comorbidities should be taken in to account in determining transplant eligibility. In the USA, patients with physiologic age <70 years are considered ASCT eligible [42]. Active agents in MM available for treatment of frontline and relapsed myeloma patients belong to five different classes: corticosteroids (dexamethasone and prednisone), alkylating agents (Melphalan, and Cyclophosphamide), anthracyclines (Doxorubicin, and liposomal Doxorubicin), immunomodulatory derivatives or IMiDs (Thalidomide, Lenalidomide, and Pomalidomide), and proteasome inhibitors (Bortezomib and Carfilzomib), which are administered in multiple combinations.
Initial Therapy for Transplantation Eligible Patients
Patients eligible for ASCT are typically treated with two to four cycles of an induction regimen prior to stem cell harvest. Many treatment options are available and the choice of the regimen is based on risk stratification, on patient comorbidities and on physician preference. Stem cell damaging agents such as Melphalan should be avoided in patients eligible for ASCT. Standard risk patients can be treated with an oral combination of Lenalidomide and low dose Dexamethasone [43]. Lenalidomide is currently preferred to Thalidomide due to superior activity and better toxicity profile [44]. Importantly, patients treated with Lenalidomide and Thalidomide should receive aspirin for thromboprophylaxis with consideration to warfarin and low molecular weight heparin in certain high-risk patients [45]. Bortezomib-containing regimens are given to intermediate and high-risk patients in combination with two additional agents, in so-called triplets. Popular combinations include VCD or CyBorD (Cyclophosphamide, Bortezomib, and Dexamethasone), VRD (Bortezomib, Lenalidomide, and Dexamethasone) and VTD (Bortezomib, Thalidomide, and Dexamethasone) [46–48]. Although VCD and VRD have not been compared in a randomized trial, the EVOLUTION trial has shown no difference between them in a small-size phase 2 study [49]. VRD is the preferred combination in patients with high-risk disease. Cisplatin and Etoposide combinations are a part of more aggressive treatment regimens (e.g., DT-PACE), typically reserved for patients with plasma cell leukemia and extramedullary disease at diagnosis [50].
Initial Therapy for Transplantation Ineligible Patients
The initial treatment regimens for newly diagnosed myeloma patients not eligible for ASCT due to age or comorbidities are the same as discussed for transplantation eligible patients. However, they are given for a total of 12–18 months. Addition of novel agents to the Melphalan-Prednisone (MP) backbone is associated to good clinical outcome, but the popularity of these regimens is limited in the USA in favor of the Lenalidomide–Dexamethasone combination. A meta-analysis of six randomized clinical trials showed that addition of thalidomide to MP (MPT) resulted in improvement in PFS and overall survival at the expense of increased toxicity, particularly peripheral neuropathy, and thrombosis [51]. A recent trial by the IFM group showed that a Lenalidomide–Dexamethasone combination, with Lenalidomide given continuously until progression, was superior to MPT [52]. Velcade-based regimens should also be considered in elderly patients, especially in high-risk group [46, 53].
High Dose Chemotherapy/Autologous Stem Cell Transplantation (HDT/ASCT)
High dose chemotherapy with autologous stem cell transplantation remains the mainstay of MM with improvement in CR rates, event free survival (EFS) and median overall survival (OS) by approximately 12 months [54, 55]. The transplant related mortality is less than 3 % [56]. Age and concomitant comorbidities should be taken in to account in determining transplant eligibility. In the USA, patients with physiologic age >70 years are considered ASCT eligible [42]. Melphalan 200 mg/m2 is the standard preparatory regimen followed by stem cell rescue. However, a reduced intensity regimen with Melphalan 100 mg/m2 may be considered in older patients or with comorbidities [57]. The benefit of HDT/ASCT in the current era of novel biologic agents, which induce deeper and sustained responses, is unclear. Another important question is the timing of HDT/ASCT, early with frontline therapy or delayed at the time relapse. Delayed ASCT at the time relapse is acceptable for some patients as randomized controlled trials showed no difference in overall survival between early versus delayed transplantation strategies [58, 59]. However, most clinicians prefer to use early HDT/ASCT in all eligible patients due to improved quality of life. If delayed ASCT strategy is adopted, patients should have their stem cells collected and cryopreserved typically after four cycles of induction therapy, which should be continued until disease progression or relapse. Finally, the attempts to improve outcomes of ASCT in MM have been largely been unsuccessful so far, except that double or tandem transplantation may offer some benefit in a subset of patients who fail to achieve a complete response or very good partial response after the first transplant [2, 60]. Therefore many centers prefer to collect stem cells adequate for two transplants. In conclusion, HDT/ASCT remains a key modality of therapy for MM until further data is available from prospective randomized trials incorporating novel agents up front.
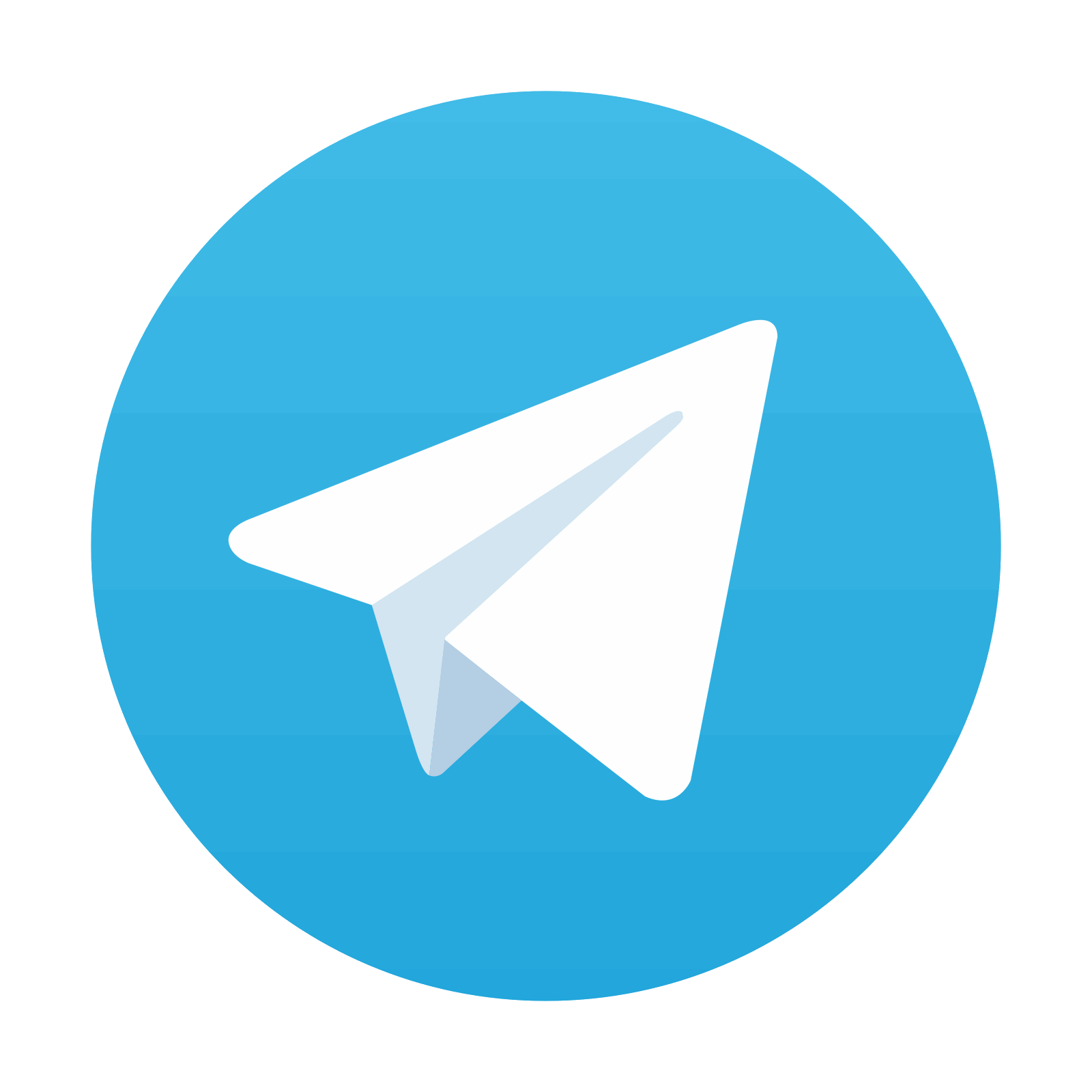
Stay updated, free articles. Join our Telegram channel

Full access? Get Clinical Tree
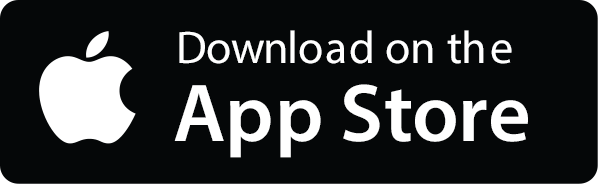
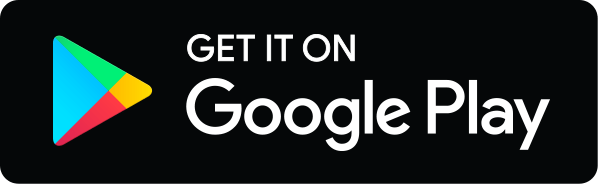