Modifiable risk factors
Non-modifiable risk factors
Body mass index
Gender
Muscle strength
Age
Pretraining fitness level
Ethnicity
Nutrition factors
Lower extremity morphology
Menstrual dysfunctions
Genetics
Muscle fatigue
Previous injury history
Flexibility
Training errors
Training surfaces
Worn-out/inappropriate footwear
Excessive training intensity
Environment
Researchers have found several non-modifiable risk factors for stress fracture in trainees. One factor that increases risk is gender with multiple studies indicating that female recruits are more susceptible to stress injuries when compared to males in the same training environment [17–23], with females experiencing an incidence of stress fracture that is 2–10 times higher than males [17, 18, 20, 24–29]. This can be related to predisposition for the female athlete triad [30, 31] as well as females general decreased muscular strength compared to males. Increasing age is more commonly associated with an increase in stress injuries [17, 32], but in one study by Milgrom et al. [33] it was reported that the younger the trainee, the more susceptible to fracture due to lack of fully developed bony strength. Risk of stress fracture decreased by 28 % for each additional year in age between 17 and 26 years. It is also reported that narrow bones in relation to cross-sectional width have a higher incidence of stress injuries [34–37]. This may also predispose females to stress fracture injury risk. White recruits have shown increased incidence of stress fractures when compared to African-American, Hispanic, and Asian recruits [17, 32, 33].
Many of the risk factors relating to stress fractures are closely related to the recruit’s fitness and nutritional habits prior to enlistment. One societal factor that may contribute to increased risk of stress fracture is the fitness level of American youth. Some research has suggested that aerobic fitness levels of American youth are declining [38]. However, there is conflicting research as Knapik et al. found no change in male fitness levels VO2max from 1975 to 1998 and slightly improved aerobic fitness in females during the same timeframe [39]. In more recent Army research, the failure rate for the initial fitness assessment has increased in males from 4 % in 2003 to 34 % in 2009 with female failures increasing from 10 to 47 % during the same timeframe [39]. As previous fitness, nutrition, and muscle strength are lower or decreased, incidence of stress fracture increases. When high body mass index (BMI) is related to poor physical conditioning, stress fractures increase [18]. Similarly, recruits (particularly, females) in the lower quartile for BMI are also at increased risk for stress fracture suggesting a bimodal effect of BMI on stress fracture risk [40]. Multiple studies of US Army recruits have consistently shown that trainees, both men and women, with less lean mass in the lower leg and thus smaller calf girth measurement are more likely to incur stress injuries [41–44]. Trainees in these studies performed fewer sit-ups during a timed test, correlating to lower muscle strength and endurance, and also ran slower [45]. Soldiers who participated in low-impact sports such as swimming prior to reporting to training had an increased stress fracture risk versus trainees who participated in basketball [46]. Those who did not participate in any physical activity before training, although not statistically significant, were also highly susceptible to stress injuries. There have been many recent studies that utilize statistical shape modeling, but they are very labor intensive and would likely not be feasible for large-scale screening in a military training environment [47–51]. A recent study by Yanovich et al. [23] attempted to link anemia and iron deficiency to stress fractures. They found a 6.6 % prevalence of stress fractures among female soldiers at the end of training, of which 28.6 % were anemic and 23.6 % were iron deficient on recruitment day .
Modifiable risk factors may include training errors, training surfaces, footwear, and environmental conditions. These risk factors can essentially be controlled and should be decreased as much as possible in order to help prevent stress injuries. Training errors that are typically associated with increased stress injuries are increasing mileage or intensity (e.g., hill running) too rapidly [14, 20]. The type of running and marching surface is also an important activity on pavement that leads to increased impact when compared to running on a rubber track or grassy surface [52]. In an Israeli Defense Force (IDF) study, stress fracture incidence increased from the usual report of 3.5 to 11.4 % when the only change in training was a switch to marching on hilly, rocky terrain instead of flat, predictable terrain [53]. When the marching returned to flat terrain, the incidence of injuries returned to 2.5 %.
The discussion of training footwear includes both boots and running shoes. Although there is no evidence to show that specific types of running shoes for specific foot types decrease the overall incidence of stress injuries, much research has investigated the use of insoles and types of boots. A Cochrane database review that suggests that insoles might reduce stress fracture rates [54] is in agreement with Milgrom et al. [9] who noted a reduction in stress fractures with the use of specifically shock absorbing orthoses as opposed to other types of orthoses. The IDF uses specific Zohar boots that have been shown to reduce tibial strain contributing to less stress fractures [55, 56].
The ideal infantry trainee would be male, African-American, should have wide bones, a low range of hip external rotation, a low-normal foot arch, be beyond teens in age, and have played basketball regularly for more than 2 years prior to enlisting. As the above stated ideal trainee is often not attainable, clinicians would greatly benefit from a prediction rule for stress fractures to use as a screening device for those trainees who do not fit the perfect mold. Moran et al. [49] published a study specifically analyzing female trainees and factors that lead to developing stress fractures. They collected data on body mass, aerobic capacity, nutrition, and hematological values. They concluded that a young female is at greater risk if she is tall, lean, feels “burnout,” has iron deficiency, and is at the end of the normal ferritin range. A follow-on study was then performed for male infantry recruits. They collected data on anthropometric variables, fitness variables, bone quality, a hematology profile, and questionnaires on activity level prior to training, a psychological assessment, nutrition assessment, and health history for a total of 77 variables. The prediction model was constructed of three variables: aerobic training (times per week), aerobic training duration (minutes per week), and waist circumference [50]. They concluded that a young male recruit is at a greater risk of developing a stress fracture if, before entering training, he ran less than 2 times per week and each training was greater than 40 min and if his waist circumference was less than 75 cm. This model was found to be able to correctly predict the presence or absence of stress fractures in 85 and 76 % of the two sample populations. With these easily screenable metrics for male and female trainees, injury prevention techniques by cadre and clinicians can be implemented with little lost training time or additional cost .
Bone Stress Injury Assessment and Diagnosis
If stress injuries cannot be predicted then they must be evaluated and treated as quickly as possible in order to ensure proper healing and reduce the possibility of comorbidities. A good clinical exam is the first step to identifying a trainee with a stress injury . Both the soldier’s history and objective exam can lead you to suspicion of injury, but in many cases it is necessary to order radiological imaging to confirm the diagnosis. Radiological imaging includes plain film radiographs, scintigraphy (bone scan), magnetic resonance imaging (MRI), and computed tomography (CT). Although plain films are commonly used as the initial standard, they have limited usefulness during the early stages of development of a stress fracture [57–67]. If positive, radiographs are diagnostic. Radiographs will usually be positive approximately 1–3 weeks after initial report of injury [68]. While standard radiographs are very specific for stress fracture, they lack adequate sensitivity. Currently, the gold standard for diagnosis is either triple-phase technetium-99m bone scan [64–66] or MRI [69, 70]. Bone scan has been reported as having a sensitivity of 100 % and specificity of 76 % [58, 66]. It is important to realize that a bone scan may pick up increased uptake of the radioisotope due to normal bone remodeling from increased training stress [21]. Therefore, results from a bone scan cannot be used to diagnose a stress injury in an area that is non-symptomatic. A study of asymptomatic Army trainees found that 98.4 % of pain-free trainees had positive bone scans during the 7th week of training [71, 72]. Bone scans may also remain positive for up to a year after the initial injury and should not be used as a tool for evaluation of healing. A negative bone scan does not always eliminate the possibility of a stress fracture. When treating soldiers with suspicion of femoral neck or pelvic stress injuries, it is important to note that studies have documented false-negative bone scans in these areas. If symptoms continue, further imaging may be needed. MRI has been shown to have comparable sensitivity to bone scan [57, 66] and superior specificity [66]. MRI is not commonly used for diagnosis due to its cost [57, 67] and even with high sensitivity and specificity, it cannot be used without limitation. Early tumors, osteomyelitis, and bone bruises also produce stress-like findings [73]. Previous studies have shown that for an accurate diagnosis of bone stress injuries in the pelvic and femoral area, MRI should be used [7, 74]. CT is not commonly used in assessment of stress fractures but can be used as an adjunct to the further assessment of known stress injuries, particularly in the navicular bone of the foot and the sacrum [73, 75].
If radiological imaging is unavailable, history and clinical exam should be used instead of other, unproven, or unreliable clinical assessments such as therapeutic ultrasound or tuning forks. Previous studies have suggested the use of ultrasound to elicit pain when applied over the fracture site as a diagnostic tool [11, 58, 64]. The results of the meta-analysis by Schneider et al. in 2012 shows ultrasound to have a sensitivity of 64 % and specificity of 63 % [76]. Though this shows a low to moderate performance, the likelihood ratios are small. Tuning forks have also been suggested as effective diagnostic tools for stress injuries. The tuning fork is applied over the suspected fracture site looking for a pain response due to irritation of the damaged periosteum [66, 77]. A poorly conducted study by Wilder et al. [70] compared the ability of 128, 256, and 512-Hz tuning forks to MRI and bone scan in 45 males. The 256-Hz tuning fork had 90 % sensitivity for detecting tibia stress fracture; however, the specificity was only 20 %. The 512-Hz tuning fork showed 83 % sensitivity and 50 % specificity for detecting tibia stress fractures. With these results, it is recommended that radiological imaging be used for the confirmation of stress fractures .
Hip, Pelvis, and Upper Leg Stress Injuries
Soldiers who report to medical treatment facilities complaining of hip pain should be screened for bone stress injuries in the areas of the femoral neck, pelvis, and femur. Femoral shaft and pelvic stress injuries, including the inferior pubic ramus (IPR), superior pubic ramus (SPR), and sacrum, are generally considered low risk and tend to heal without continued symptoms allowing the soldier to return to and complete training. Femoral neck stress injuries (FNSIs) are much less common, but high risk, and both providers and company staff should be aware of their signs and symptoms. Differential diagnosis of hip/pelvic stress injuries includes acetabular impingement, Iliotibial band (ITB) friction syndrome, greater trochanteric bursitis, sacroiliac joint pain, radicular low back pain, or muscle strain.
Femoral Neck Stress Injuries
FNSIs represent 5–10 % of injuries that occur during IET, [13, 78], but are by far the most costly than most training injuries with an estimated cost of nearly $100,000 per injured trainee. There are two classifications of FNSIs: compression-side injuries and tension-side injuries. Compression-side injuries are the most common and most are treated nonoperatively [79]. Tension-side injuries are of much higher risk due to the risk of displacement and normally require operative fixation. Risks that are associated with displaced FNSIs include avascular necrosis and severe osteoarthritis [80]. Recruits who sustained FNSIs during training were found to be at a 25 % higher risk to sustain stress fractures in future training [46].
Clinical signs and symptoms for FNSIs are very general, which often make diagnosis challenging. Most soldiers will not complain of pain until about 4–7 weeks of training [81] or 13–16 weeks [82]. The typical presentation is poorly localized groin or thigh pain including intermittent, tight sensation in the groin after activity, and increased pain while climbing upstairs or taking downstairs [59, 83]. Clinical exam may reveal pain at end ranges of hip motion testing, especially internal rotation, and pain with a single leg stance or hop; however, the single leg hop test has been discontinued in the US Army IET medical facilities when a suspected FNSI is considered a differential diagnosis due to the possibility of causing displacement of the stress fracture. Risk factors include coxa vara, female gender, nutritional deficiencies, and decreased bone mineral density [84–88]. Higher age, poor muscle strength, and a poor result in the 12-min run test are significant risk factors for FNSIs reported from Finnish military training [89].
If a FNSI is suspected from the clinical exam, X-ray is ordered to rule out grade 4 fracture (Fig. 5.1). If the plain films show no fracture, bone scan is ordered. Bone scan has been shown to have very high sensitivity, but moderate specificity, so results from a bone scan cannot be interpreted independent of a physical exam. Even if the bone scan returns negative, if the patient is not progressing through normal treatment with physiological signs of healing, an MRI can be ordered. MRI is the gold standard for identifying femoral neck stress fractures due to its high sensitivity and specificity, but due to cost and availability, X-ray and bone scan are generally performed prior to MRI as a fracture can be seen utilizing these imaging techniques as well .
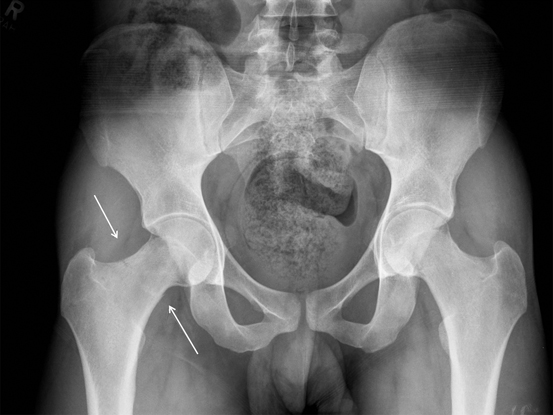
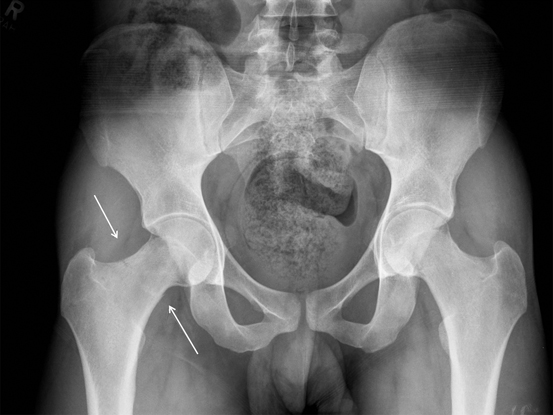
Fig. 5.1
Grade 4 stress fracture of the femoral neck
Treatment for FNSIs is very patient and symptom-dependent and can involve both operative and nonoperative treatment, depending on severity and location of injury. Nonoperative treatment generally consists of crutch ambulation with partial or weight bearing as tolerated restrictions on the affected hip during the first 4–6 weeks after diagnosis of injury. This is followed by 4–6 weeks of nonimpact cardiovascular training with light strengthening and stretching in a pain-free range of motion. This progresses to 4–6 weeks of return to impact and running, with the first 3–4 weeks of run training performed on a treadmill, later returning to running outside. At this point, depending on residual symptoms and the MOS per week of training, the soldier may be reintroduced back to mainstream training.
Operative treatment is indicated for compression-sided fractures measuring 50 % or greater of the femoral neck width and any tension-sided fracture, or completed fractures. Surgical intervention includes pinning of the femoral neck (Fig. 5.2). There is risk of further complication after surgical intervention including avascular necrosis of the femoral head, malunion, or nonunion.
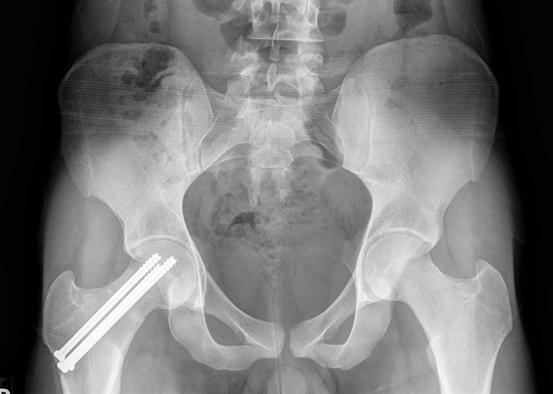
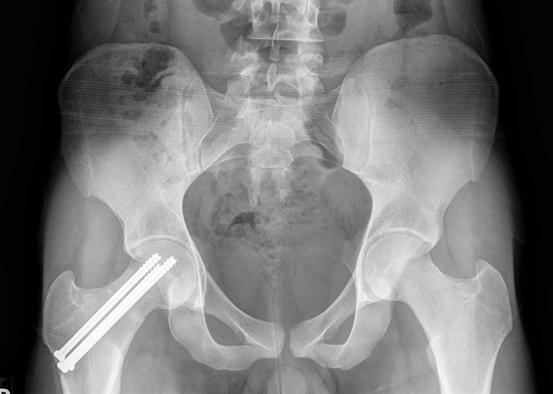
Fig. 5.2
Open reduction and internal fixation of femoral neck stress fracture
Outcomes for trainees in a stressful IET environment are very subjective and difficult to standardize. In a study by Weistroffer et al., despite appropriate treatment for FNSIs as evidenced by the absence of additional complications, many of their patients still complained of pain in their hips 5–7 years after surgery [90]. Issues of secondary gain cannot be ruled out. In the military setting, if a soldier is not recommended to return to training due to continued complaint of pain or inability to rehabilitate their injury fully, they are sent to the Medical Evaluation Board. This can be a lengthy process, even for a soldier who has been in the military for less than 6 months, and can end up creating other negative consequences.
Time lost from training due to injury varies dependending on the significance of the injury. If detected early, a femoral neck compression-side stress injury of less than 50 % width can rehabilitate normally in 3–4 months. If an injury has progressed to requiring surgery, the length of rehabilitation time is increased and the likelihood of successfully returning to training is decreased .
Pelvic Stress Injuries
The most commonly treated pelvic stress injury seen in IET is the inferior pubic ramus (IPR) stress injury. If not treated early, these injuries can progress to include the superior pubic ramus (SPR) and sacrum. IPR/SPR stress injuries are caused by the repetitive stress on the adductor muscle group attachments on the inferior or superior pubic ramus (adductor magnus, brevis, gracilis, pectinius, and obturator externus). When soldiers are made to “stride out” during marching movements, this muscle group is on a repetitive stretch and contract motion that can be outside of soldiers’ comfortable range and strength limits. This repetitive overstretching and activation, coupled with fatigue in hip stabilizing muscles from the marching activity, can lead to increased stress on that bony area, promoting stress injury [15, 91].
Clinical signs and symptoms include pain with palpation over the IPR, pain with resisted adductor testing, and pain with hip flexion. The soldier may complain of pain with running and performing sit-ups. The current practice guidelines at all US Army IET medical facilities are to immediately place the soldier on crutches and order imaging. Regular radiographs are ordered first, in order to rule out a fully progressed stress fracture. If radiographs are normal and the soldier is still complaining of pain, bone scan or MRI is ordered as deemed fit. There are not many researched intrinsic or extrinsic risk factors specifically linked to pelvic stress injuries. They are more commonly reported in females when compared to males in the same training units. In 1991–1992, pelvic stress fracture incidence of 11.2 % was reported in an Australian study of female Army recruits. In the following year, 0.1 % incidence was recorded in the same training unit for males [91].
Treatment for pelvic stress injuries is similar to that of FNSIs. Treatment starts with crutch ambulation until walking without the assistive device is pain free and then progresses to light nonimpact cardiovascular exercises and stretching. Once clinically and radiographically cleared for signs of healing, slow return to activity, specifically running and ruck marching is introduced. Hip, gluteal, and general lower body strength training is used to help prevent this injury from recurring when placed back in training.
It is difficult to state time lost from training due to injury as this is dependent on timely diagnosis and the extent of injury, as well as the recruit’s motivation to return to training. In general, trainees with pelvic stress injuries that have progressed to stress fracture are removed from training, rehabilitated for 2–4 months before returning to training. Also dependent on training time lost is how far the soldier made it in training and the training requirements for specific units.
Femoral Shaft Stress Injuries
Femur stress injuries often present as quadriceps muscle strains or knee pain. Clinical signs and symptoms include low level of pain with impact activities, a positive fulcrum test [92], and/or pain over the injury site. Lacking the protection of significant pain in the presence of femoral stress fracture, these injuries are more likely to progress to full fractures [93]. The most common location for a femoral shaft injury is on the medial (compression) side of the femur, which is the attachment site for the adductor and vastus medialis muscle groups. Rarely are condylar stress injuries seen, but they are reported both medially and laterally [30, 94, 95]. Femur stress injuries tend to heal faster than other stress injuries with activity modification and slow return to impact [30]. Depending on the severity of the injury, some femur stress injuries can be left in training if they are allowed a decrease in running and activity level .
Lower Leg Stress Injuries
Leg stress injuries include the areas of the medial tibial plateau, tibial shaft, medial malleolus, and fibula. Exercise-induced stress fractures are common in the lower extremities, with 75 % occurring in the tibia [96]. Occurrence of this injury in the general athletic population is less than 3.7 % [92, 97, 98], but is reported anywhere from 0.9 to 64 % in military recruits [17, 18, 28, 36, 95, 99, 100]. In a study by the IDF, data on 392 trainees showed that greater static valgus alignment of the knee was a significant risk factor for tibial stress injuries, but additional research is still needed [99].
Tibial Stress Injuries
Tibial shaft stress fractures are the most common anatomical region of involvement in trainees and athletes (Fig. 5.3) [25, 101]. Other diagnoses that must be ruled out are shin splints, compartment syndrome, or other lower leg muscular overuse symptoms. Symptoms include increased pain with impact activities, pain with palpation over the injury site, and sometimes pain with resisted ankle muscle testing. Tibial stress fractures are often characterized by a high level of pain even in the early stages of stress injury [93]. Batt et al. reported that positive findings on a bone scan read as shin splints can be considered as the beginning of stress-related changes to the tibia and should be treated as such to prevent progression to stress fracture [102]. When the tibia is divided into proximal, mid, and distal 1/3, most injuries that are diagnosed by clinical exam and radiographs are reported in the middle third of the shaft, versus when MRI is used as the imaging technique and injuries in the distal 1/3 of the tibia are more frequent [9, 103–106]. When MRI was used, lower level stress injuries (grades 1–2) to the distal 1/3 of the tibia were seen more frequently. Treatment for tibial stress injuries includes reduction of impact activity and slow return to training as symptoms subside. Midshaft stress fractures have reports of the poorest outcome with regard to returning to military training whereas proximal tibial stress fractures have reports of good potential for return to training [107]. Because of the increased report of pain with these injuries, most trainees are pulled from training before they develop into actual fracture, leading to reduced healing time and return to activity .
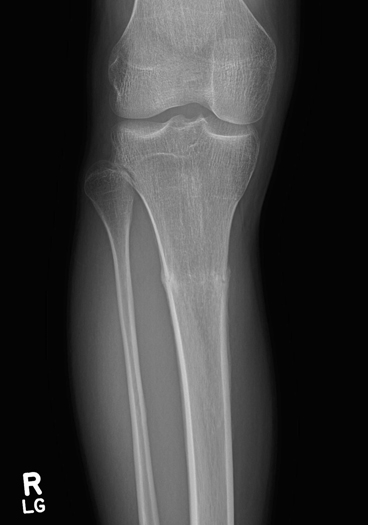
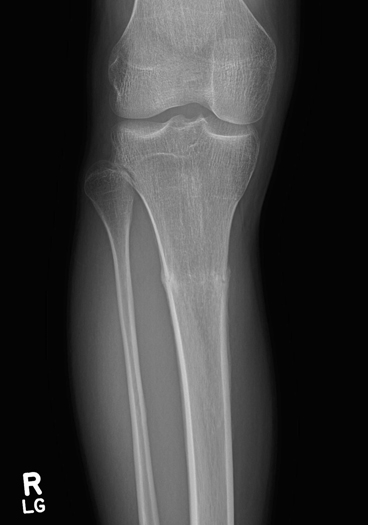
Fig. 5.3
Tibial shaft stress fracture
Fibular Stress Injuries
Fibular stress injuries are less common as the fibula is not a significant weight-bearing bone. These types of stress fractures are attributed to muscle traction and torsional injuries. A soldier who primarily runs or marches on the outside of their feet may have an increased likelihood of developing this type of injury. Differential diagnosis includes muscle strain and compartment syndrome. Only 0–1 % of fractures reported among Israeli military recruits were reported as fibular stress injuries [9, 99].
Foot Stress Injuries
Foot stress injuries commonly diagnosed during military training frequently impact the metatarsals, tarsals, and calcaneus. Some research suggests that foot arch height may influence the risk of stress fracture with associated intense physical training, but more research is needed to determine the association between arch height and type of stress fracture [15, 108]. Pes planus has been found to be a protective factor for stress fractures in basic training with a report of only 10 % incidence of stress fractures in trainees with this foot type versus 39.6 % incidence among trainees with high arches and 31.3 % incidence of stress fractures in trainees with average arches [109].
Metatarsal Stress Injuries
Metatarsal stress injuries , also known as fatigue or march fractures, have been documented as a medical condition in the military since 1855 [110]. The most common locations of stress fractures of the metatarsals are in the second and third bones. A stress fracture of the base of the fifth metatarsal is less common but of a higher risk. Clinical signs and symptoms include pain with palpation, most commonly on the dorsum of the foot, pain with axial loading, and fulcrum testing. Differential diagnosis includes metatarsalgia, Morton’s neuroma, and tendon strains. Frank metatarsal stress fractures are often completely asymptomatic until a complete fracture occurs. Milgrom et al. reported that soldiers who were completely asymptomatic prior to a long ruck march finished with a fracture of one or two of the metatarsal cortices [37]. When comparing incidence of stress injuries and body part, tibial and femoral shaft often outnumber metatarsals. But when examined over the course of a year of training, both tibial and femoral stress injuries did not occur after week 28, whereas metatarsal stress injuries continued to occur [93]. By measuring in vivo strains at both the second metatarsal and tibia, it was found that simultaneous strains in the metatarsals were four times higher than those of the tibia during both treadmill walking and running. With this information, it can be deduced that metatarsal stress fractures can be caused by cyclic overloading alone, without the addition of a remodeling response to increased activity [93]. Treatment includes decreased weight-bearing status until pain free with gradual return to impact activities. It is important to allow the trainee to start putting stress (weight) on the affected area once they are pain free in order for the remodeling process to occur [111]. In a case report written by a military physical therapist stationed at Fort Sam Houston, a trainee was treated very conservatively with crutches, a walking boot, and 1 month of convalescent leave. The trainee was not given any exercise program while on convalescent leave and once she returned, she was started in an aquatics program. Her healing time was most likely significantly delayed due to the lack of stress on the bone while on leave. Depending on how quickly the metatarsal stress injury is diagnosed, the length of the training time lost will be determined. If the symptoms are caught early, the soldier may be allowed to stay in training if they can limit impact activities. If the injury progresses to full fracture, normal treatment is 4–6 weeks of no running or significant impact with slow return to activity, which is dependent on the symptoms .
Calcaneal Stress Injuries
Calcaneal stress injuries are considered to be fairly common in athletes and military recruits [23, 112–114]. Previous research on calcaneal stress injuries found the posterior part of the bone to be the most frequently involved, but these studies had been performed over 30 years ago when MRI and bone scan were not readily available [114–116]. Clinical symptoms include pain with palpation or squeezing of the calcaneus, pain with impact activity, and sometimes foot swelling. Differential diagnosis includes retrocalcaneal bursitis, Achilles tendonitis, plantar nerve entrapment, radiculopathy, and posterior ankle impingement [117, 118]. In one Finnish study by Sormaala et al., the trainees were evaluated by an orthopedic surgeon for stress injury symptoms and plain radiographs were taken of the most symptomatic area [119]. The patients were then evaluated with MRI. Of the injuries, 56 % were located in the posterior part of the bone, which is much less than the previously reported 95–100 % [114–116]. Another 26 % were located in the anterior portion and 18 % in the middle portion of the bone. With a clearer diagnosis of area of stress injury with the use of MRI, injuries to the anterior calcaneus were also associated with injuries of the cuboid and talus. When performing the clinical exam and follow-up exams, it is important to check other areas of the foot for concomitant stress injuries. Of all the stress injuries of the calcaneus that were detected with MRI, only 15 % were detected on radiographs. Patients are managed with reduced activity and very rarely is casting necessary. Recovery and likelihood of return to duty status is good following calcaneal stress injury .
Tarsal Stress Injuries
Tarsal stress fractures most commonly include stress injuries to the talus and navicular, but may involve the cuboid and cuneiforms. Matheson et al. reported longer recovery times for injuries to these areas when compared to stress injuries in the lower extremity due to delayed diagnosis [101]. The clinical exam should consist of complete palpation of the foot to get a clearer idea of point of pain origin and followed with diagnostic imaging. Stress fractures of the talus have been associated with excessive subtalar joint pronation [120, 121]. Stress fractures of the navicular typically present as vague dorsal foot pain. Squeezing the midfoot will elicit symptoms. Differential diagnosis includes tendonitis or a symptomatic accessory navicular bone. Patients are managed with reduced activity and the likelihood of return to full duty status is good .
Anterior Knee Pain
One of the most common problems among trainees is anterior knee pain, which has been called the “black hole of orthopedics” [8]. Anterior knee pain can be a chronic and disabling condition. Also known as patellofemoral pain, or more colloquially as “runner’s knee,” this condition represents a challenging problem for clinicians which is extremely difficult to treat. Its causes are not clearly established, although it may be related to poor physical fitness, poor bony alignment, or abnormal lower extremity movement patterns [122]. Because its causes are not clearly understood, definitive prevention and treatment options remain elusive as well. Primary treatment of anterior knee pain usually consists of physical therapy focused on restoring normal lower extremity movement patterns and strengthening and stretching lower extremity musculature. Evidence to support exercise therapy for anterior knee pain is lacking though as few trials have compared physical therapy treatment to a placebo. Like many other conditions that affect trainees, one of the best treatment options for the trainee with anterior knee pain is rest. However, it is usually difficult to provide adequate rest to the trainee with anterior knee pain while still enabling him or her to complete the physical requirements of IET. Anterior knee pain is particularly concerning because of the strong likelihood of prolonged pain and disability. In one study, nearly half of Israeli recruits who developed anterior knee pain during training continued to have symptoms 6 years after the completion of training [55].
< div class='tao-gold-member'>
Only gold members can continue reading. Log In or Register a > to continue
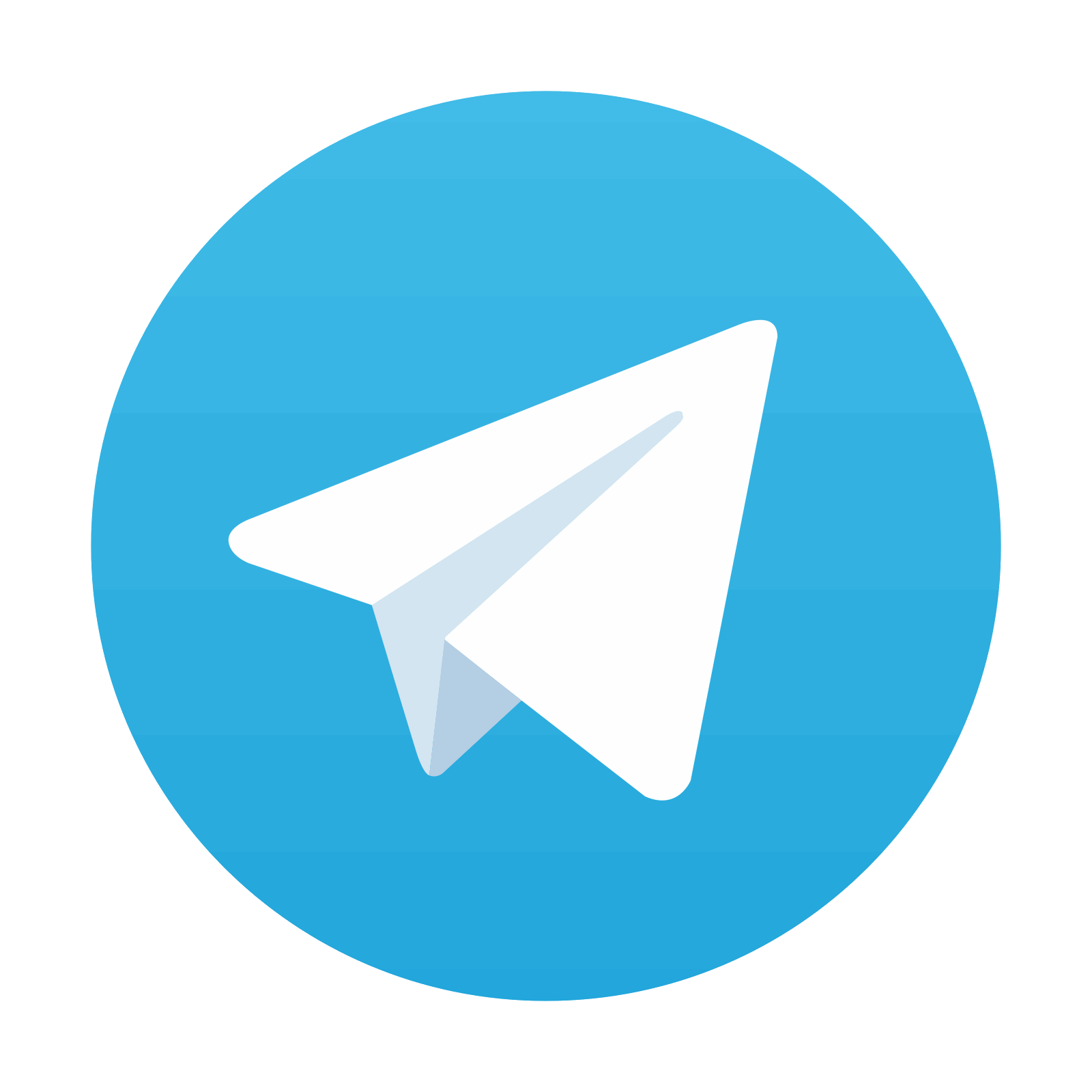
Stay updated, free articles. Join our Telegram channel

Full access? Get Clinical Tree
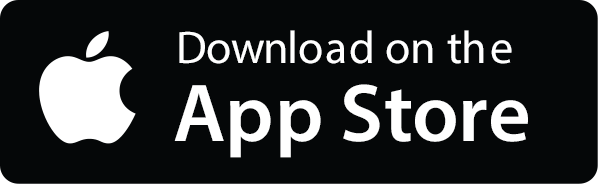
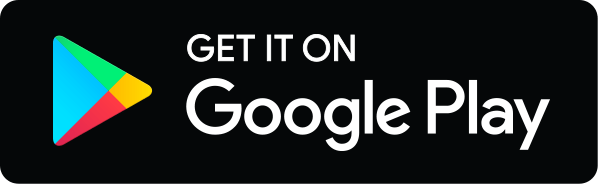