Musculoskeletal Infection Associated with Skeletal Trauma
Infection is a dreaded complication of musculoskeletal trauma. It occurs most commonly after open fractures but has been reported to occur in as many as 5% of cases following the surgical treatment of closed fractures. In open fractures, the reported incidence ranges from 10 per 1,000 (0.1%) for Gustilo type I open fractures to 25 to 50% of Gustilo type III open fractures. When infection occurs, treatment becomes more difficult and prolonged, management options are restricted, and patient outcomes are compromised. If infection complicates the treatment of a long-bone fracture, the cost of care is increased by an average of 20.5% per patient, and the length of hospital stay is increased by an average of 36.2%. Because of increasing numbers of revision surgeries for infection, analysis of NIS (Nationwide Inpatient Sample) data has shown that there is a greater economic burden on the healthcare system for infected revisions, even though the average estimated hospital cost per procedure remained relatively stable in the 2000s. Costs associated with the management of musculo-skeletal infections vary widely but are higher than those associated with preceding interventions, such as elective joint arthroplasty or fracture treatment.1
The estimated total hospital cost in the United States incurred for care of infected primary total joint replacements grew from $365 million in 2001 to $771 million in 2011. This is projected to surpass $1.1 billion by 2015 and will cost US hospitals $1.63 billion (CI, $1.57 billion to $1.69 billion) by 2020. This is in addition to the cost of treating infected fractures, the care which is equally as costly. For example, treatment of necrotizing fasciitis associated with a fracture can be particularly costly, with charges ranging from $20,000 to $866,000 and an overall mortality rate >10%.2
Prevention of Infection
Because of the difficulty in treating musculoskeletal infection, prevention of infection is of paramount importance. Measures should be taken to optimize the host′s defensive capabilities. Nutrition should be assessed and supported. Malnutrition is common in injured or hospitalized orthopaedic patients, and it leads to immune compromise.3,4 Protein malnutrition adversely affects both humoral and cell-mediated immune mechanisms. Information gathered in the history or from the physical examination that is suggestive of malnutrition can be evaluated with laboratory tests such as serum albumin and total lymphocyte count, which should be above 3.5 mg/mL and 1,500 cells/mL, respectively. In cases of obvious malnutrition or diagnostic laboratory values, nutritional support and supplementation should be instituted. Tobacco use can lead to wound-healing failure, with resultant infection. Medical conditions that impair immune function should be aggressively treated. Patients with multiple comorbidities, including diabetes, connective tissue disease, peripheral vascular disease, or any medical condition that might impair the function of their immune system, are especially prone to incur a wound infection following surgery.
Prevention of infection in open fractures has been discussed in Chapter 2. Some of the factors that contribute to the development of infection in open fractures are under at least partial control of the surgeon. Of these, the most important are (1) the adequacy of the initial debridement and irrigation, and (2) antibiotic treatment. The initial debridement procedure must be thorough, careful, and systematic. Traumatic wounds should be extended longitudinally. The ends of the bone should be exposed and examined in high-energy open fractures. All foreign material as well as obviously devascularized tissue must be identified and removed, using sharp dissection. The tourniquet should be used sparingly and briefly because tissue bleeding is one of the best indicators of viability, and marginal tissue should not be subjected to additional ischemia. Exploration and debridement should be done using a systematic layer-by-layer approach. Skin that has questionable viability but is in an essential area (hands, feet, pretibial area) should be preserved if possible. Repeat debridement at 24- to 48-hour intervals is performed until a clean, viable wound is obtained.
Irrigation with high volumes (8 to 10 L) of fluid should be performed. Use of higher irrigation pressure increases the effectiveness of particle removal, but animal studies have suggested it could delay bone healing. Furthermore, the use of high-pressure irrigation may actually push any contamination even further into the wound bed. This occurrence, combined with the infusion of air and fluid into the tissues, may serve to inhibit the body′s ability to clear any infective foci that may develop. Thus, there are both theoretical advantages and disadvantages to the use of high-pressure wound irrigation, and researchers have tried to use animal models to determine which aspects are more clinically relevant: the benefit of more thorough mechanical cleansing or the potentially greater biological insult. In one such study, pulsed lavage was contrasted with simple bulb irrigation using various irrigation solutions in a contaminated bioluminescent wound model. Pulsed lavage decreased the amount of relative luminescent units by 52%, 64%, and 70% when 3, 6, and 9 L, respectively, of irrigant were used. The bulb syringe irrigation method reduced the amount of relative luminescent units by 33%, 44%, and 51% at these same time points.5 However, wound evaluations were obtained at routine intervals, and by 48 hours the bacterial counts in the pulsed lavage groups had rebounded to within 94% of pre-irrigation levels. This was compared to a rebound of only 48% in the bulb syringe group.6
The use of antibiotic additives is of no proven value in open fractures, and antiseptics such as Betadine, hydrogen peroxide, and chlorhexidine should be avoided because they are potentially harmful to host defenses.7 Detergent irrigation additives have shown some promise in laboratory studies.8 The effectiveness of additives was evaluated in the same bioluminescent animal fracture model.6 Normal saline, bacitracin, castile soap, and benzalkonium chloride were all analyzed in terms of effectiveness and post-irrigation rebound of bacterial counts. The greatest reduction in bacterial counts was with castile soap, followed by benzalkonium chloride, bacitracin, and saline. The highest rebound was measured in the castile soap group, which rebounded to 120% of the pretreatment bacterial levels. Normal saline solution demonstrated the lowest rebound at (68%). A similar study by the same authors noted that potable water was also effective in removing bacterial loads and also demonstrated relatively low rebound of 71%.9
It appears that many of the common approaches used to remove bacteria from wounds, such as irrigants (other than saline solution) or high-pressure devices, may not result the best clinical outcome. A newer technology, focused fluid jet lavage, uses a suction effect to draw the devitalized tissue into a high-pressure jet of normal saline, which then cuts the devitalized tissue free from the surrounding tissues and is removed from the wound via a suction apparatus. Clinical studies evaluating this “fluid friendly” technology have documented an overall decrease in the time necessary to complete an adequate debridement, a decrease in the amount of irrigant used, and most importantly a decrease in the number of procedures necessary to achieve definitive wound closure.10,11 When they treated chronic lower extremity stasis ulcers, Caputo et al12 noted debridement times that were 39% shorter than those with standard debridement techniques, with a significant reduction in the use of pulsed lavage and saline (p < 0.001). The median time to wound closure was 71 days with focused fluid jet lavage versus 77 days with conventional therapy (p = 0.733). Potential cost savings were identified as well due to the shorter debridement time and time to closure.
Clearly, surgical debridement is the most important factor in the early phases of wound management and in avoiding a postoperative wound infection. Between debridement procedures, prior to definitive wound closure or coverage, the wound should be prevented from desiccating by the use of appropriate dressing techniques (e.g., an antibiotic bead pouch13) or by the use of negative-pressure wound therapy (NPWT), such as the vacuum-assisted closure (VAC) system available from Kinetic Concepts Inc. (San Antonio, TX).14 NPWT has been shown to be effective in the treatment of open fractures when utilized for interim dressings between each debridement and prior to definitive wound closure. Compared with a similar group treated with fine mesh gauze, there was a significant difference between the groups in the incidence of total infections. Patients treated with NPWT were only one-fifth as likely to have an infection compared with patients randomized to the control group.15
Systemic antibiotic treatment for open fractures is considered the standard of care, although the details of the selection of agents and the duration of use are controversial. First-generation cephalosporin coverage for 2 to 3 days is adequate for low-grade open fractures, whereas higher grade open fractures (such as grade III) may benefit from the addition of an aminoglycoside and coverage for a longer period (5 days). Specific injury settings, such as agricultural or aquatic settings, may dictate additional antibiotic coverage. Penicillin should be added for soil contamination to cover anaerobic organisms. Studies show significant reduction in the incidence of infection if given early, irrespective of the timing of debridement.16
Stabilizing the fracture is a key component of preventing infection. For unstable fractures, this may be done with internal or external fixation. The use of internal fixation is acceptable in open fractures once the wound is adequately debrided and irrigated.17 It is critical to avoid additional surgical trauma to the vascularity of the bone or soft tissues by use of gentle, minimally invasive techniques.
Prevention of infection in the surgical treatment of closed fractures follows the same principles as other orthopaedic operations, except that these surgeries should be considered high risk due to the implantation of hardware and the immune compromise that trauma entails. Strict adherence to “aseptic technique” has been shown historically to dramatically reduce infection rates.3 This includes limitation of traffic through the operating room (OR), shaving the affected area at the time of surgery (not before), careful and thorough preparation of the skin with antiseptic, gentle handling of tissues, and awareness of sterile technique on the part of everyone involved in the operation. Preoperative antibiotics have been shown to reduce infection rates in the surgery of closed fractures in a prospective randomized, blinded, and placebo-controlled trial.18 Prophylactic antibiotics should be given within the 2 hours preceding incision, at least 10 minutes before tourniquet inflation.18 There is no documented benefit to continuing antibiotics beyond 24 hours postoperatively, and one study showed successful prophylaxis with a single preoperative dose of ceftriaxone.17 Cefazolin has been commonly chosen because of its activity against the most common pathogens (gram-positive skin flora), coverage of common gram-negative aerobes, high peak concentrations, long half-life, and a tendency to concentrate in the hematoma.19
The timing of wound closure for open fractures has always been a highly subjective judgment call, with few definitive guidelines as to how soon the open fracture can be closed primarily or via secondary wound coverage procedures. DeLong and Born′s group20 performed a retrospective review of open fractures and found that immediate closure of wounds after thorough debridement by an experienced fracture surgeon appeared to cause no significant increase in infection or need for secondary procedures. The fractures in this study were primarily lower grade injuries, and concern exists for immediate closure in those higher energy fractures with much more devitalization and contamination.
A large retrospective study evaluated the efficacy of using post-irrigation and debridement cultures as a basis for indicating overall wound health and as objective information to help determine the timing of wound closure in the management of open fractures.21 If the post-irrigation and debridement cultures were negative 48 hours after the initial irrigation and debridement, the wound could be successfully closed at the next operative intervention. However, if the initial post-debridement cultures were positive, the patient was returned to the OR for an additional debridement as well as additional post-debridement cultures. This process was repeated until the cultures were negative, at which time the wounds were closed either primarily or via a soft tissue procedure. Utilizing this protocol in over 600 open fractures, the authors demonstrated an overall rate of infection of 4.3% for all open fractures. Specifically for Gustilo type IIIB fractures, their reported rate of infection was only 4.2%. This very low rate of infection for these severe open fractures demanded strict adherence to this debridement and culture protocol. The authors found that closure did not require extra time for higher energy fractures, even with an average of five debridements prior to final closure. They felt that this number of debridements was necessary in these severe injuries in order to avoid infection by delineating fully the zone of injury prior to wound closure.
One key aspect of avoiding infection in trauma surgery is the appropriate timing of surgery when treating closed fractures. Many closed fractures are surrounded by significantly damaged soft tissues that impair the skin′s ability to heal incisions or to perform the defensive barrier functions of normal skin. In this situation, the surgeon must wait until the soft tissues have healed before performing definitive fracture surgery. Contrary to previous opinion, there is no safe early window in which one can operate on damaged tissues. A commonly used indicator of when the skin is ready is the “wrinkle test,” performed by gently pinching the skin together between two fingers. If it is soft, mobile, and forms normal wrinkles, it may be able to tolerate a surgical incision (Fig. 3.1). While awaiting the resolution of blisters, ecchymosis, and swelling, the surgeon can use temporary external fixation to maintain the length and alignment of the extremity and to provide some stability for the healing of the tissues. This technique has been called “traveling traction” and can span joints without adverse effect22 (Fig. 3.2). This technique enables elevation of the extremity by suspending the frame and by applying pneumatic devices such as foot pumps to aid in the resolution of swelling. Soft tissue healing may take several weeks. This staged treatment strategy for complex injuries has been shown in several studies to reduce infection rates compared with acute open reduction and internal fixation (ORIF).23


At the time of conversion to definitive fixation, the frame should be partially disassembled to the minimum required for maintenance of stability intraoperatively. The fixator can be prepped and draped into the operative field and used to maintain reduction during internal fixation.24 The infection rate for intramedullary nailing after external fixation has been reported to be unacceptably high if there has been prior pin-site infection,25 but this complication seems to be less common if the pin sites are clean and the duration of fixation is short. Although performing the conversion to intramedullary (IM) fixation within 2 weeks is best, we have used temporary external fixation for up to 4 weeks without a problem.
Diagnosis
Established posttraumatic osteomyelitis often presents with pain, instability, chronic skin changes, and purulent drainage through fistulas (Fig. 3.3). When this occurs, diagnosis is easy, but treatment can be much more difficult than when infection is found early. Local infection at the site of surgical intervention that manifests itself within 14 days of internal fixation is usually confined to the soft tissues only.26 However, the classic clinical signs and symptoms of wound infection may be difficult to interpret in the setting of fracture surgery. The traditional signs of pain, fever, swelling, tenderness, warmth, and erythema may be attributed to the injury or surgical treatment. Damage to the soft tissue envelope in the proximity of the fracture is frequently underestimated during the initial evaluation and management.27 Marginal skin necrosis or superficial wound breakdown with erythema or minimal serous drainage may not represent bacterial infection but rather a process related to the soft tissue trauma itself.

Patients who present more than 2 weeks following their surgery more commonly display the classic signs of infection. These findings occur beyond the time frame attributable to the surgery, and may be worsening rather than improving.26,28–31 Patients may have a low-grade or indolent infection in which the clinical signs and symptoms are absent or subtle. Surgeons should maintain a high level of suspicion regarding infection throughout the course of fracture treatment, particularly when confronted with delayed or nonunion.
Diagnostic Laboratory Studies
Preliminary studies that should be obtained when one suspects an early postoperative infection usually include white blood cell count (WBC), erythrocyte sedimentation rate (ESR), and the C-reactive protein (CRP). An elevated WBC may be seen with acute infection but is often normal with chronic osteomyelitis. The ESR is a sensitive but non-specific measure of inflammation, and is elevated in 90% of patients who present with a serious orthopaedic infection. It can be influenced by numerous factors such as age, fluid balance, nutritional status, smoking status, and hormonal changes. After major surgical interventions or extensive trauma, the ESR often increases to high levels but should return to normal within 6 months. As a result of high sensitivity, the ESR is utilized as a screening tool. Because of the lack of specificity, care must be taken in the interpretation of a persistently elevated or even rising ESR as an isolated clinical finding. The ESR in combination with the patient′s age and immune status has predicted the success of antibiotic treatment; however, the ESR′s response alone is not a clear predictor of success.
C-reactive protein is an acute-phase reactant, a substance that rises in concentration in the bloodstream as a response to inflammation or tissue damage. The concentration of CRP increases several hundredfold within 6 hours after a triggering infectious inflammatory stimulus. There is a constant clearance rate of CRP from the bloodstream with a half-life of approximately 24 to 28 hours. A return of CRP levels to baseline indicates the effectiveness of treatment. However, if high levels of CRP persist or a second spike is noted, this may be a warning sign of a new process or exacerbation of the previous inflammatory or infectious process.32 A transient rise in CRP is demonstrated following uncomplicated fracture surgery, which peaks at about 2 days and should decline rapidly over the next few days. Baseline levels are achieved by 3 weeks after uncomplicated surgery. Infected fractures demonstrate a rapid rise and do not normalize unless specific therapy is undertaken.
Obtaining serial CRP levels in patients with open fractures and nonunions may have value in helping predict the development of infection following surgery. Wright and Khan33 identified 52 patients who had soft tissue reconstructions following open fractures and chronic infections, and nonunions of the lower limbs; 41 of the patients reached peak CRP levels within 4 days of their final surgery. A peak CRP level that occurred longer than 4 days after surgery indicated an infection (p < 0.01). Acute and chronic groups showed a peak in mean CRP at day 2. Patients who developed wound infections showed significantly elevated CRP levels compared with patients who did not develop a wound infection at day 7 (p = 0.05) and day 8 (p < 0.001). Patients with nonunion or deep infections showed persistently elevated CRP levels throughout the treatment time. The authors found that the CRP peaks on day 2 following soft tissue coverage and falls thereafter. Peaks after day 4 indicate infective complications or further surgery. Patients with chronic wounds show a slower decrease in their CRP. Persistently elevated CRP following surgery is associated with infection.33
An additional study also confirmed the value of serial CRP and interleukin-6 (IL-6) levels in predicting infection following fracture surgery.34 Serum CRP and IL-6 concentrations were measured preoperatively and on postoperative days 2 and 4. For the majority of patients, CRP levels were higher on day 2 than they were preoperatively. On postoperative day 4, CRP levels declined sharply in patients without infection (mean, 8 µg/mL) but were persistently elevated in patients who subsequently developed infection. In those patients who developed an infection, the IL-6 concentrations were elevated at day 2 (mean, 689 pg/mL) and decreased progressively at day 4 (mean, 175 pg/mL). The authors determined that infection correlated with CRP and IL-6 levels, and that serial serum measurements of IL-6 and CRP levels can help in the early diagnosis of infections after open fractures before the infection is clinically evident.34
Normalization of CRP is rapid after control of the infection, and declining levels can be seen within 8 hours of instituting effective treatment. Measurement of both ESR and CRP is helpful in differentiating septic and mechanical loosening with failing hardware, detecting complications, and monitoring the effects of treatment. CRP is more sensitive than ESR for these purposes.35
Imaging
Radiographic Imaging
Conventional radiographs remain the initial imaging modality in the diagnosis of subacute or chronic infection. Patients seen with a deep infection may present with classic X-ray findings, such as periosteal elevation, endosteal scalloping, areas of hardware failure or loosening, radiolucencies around implants, and endosteal and periosteal scalloping (Fig. 3.4). These radiographic changes occur later in the course of the infectious process and require actual bone destruction or reactive changes. Posttraumatic changes and hardware may obscure findings on radiographs and make interpretation difficult, yielding a low sensitivity (< 20%).

Computed Tomography
Scanning with computed tomography (CT) can be useful in detecting osseous destruction and the presence of a sequestrum, foreign body, or gas formation. The sensitivity and specificity of CT for the diagnosis of osteomyelitis has not been established clearly and are in the range of 65 to 75%.36 However, CT is generally less sensitive than other modalities in the detection of early bone formation or erosion. CT is valuable in the determination of osseous continuity. CT scanning yields valuable information regarding the three-dimensional architecture of regions of bone loss or defects. This information is crucial in planning the reconstructive procedures necessary following the eradication of an infection.37,38
Nuclear Medicine
Bone scintigraphy is much more sensitive than conventional radiographs. A variety of radiopharmaceuticals are available for use in scintigraphy. Traditional bone scanning uses technetium polyphosphate (99mTc), which accumulates in areas of reactive new bone formation and increased bone blood flow. However, it does not specifically diagnose the increased uptake as osteomyelitis.37 The specificity of this test for infection following trauma may be below 20%.39 To overcome this limitation, 99mTc scans are often combined with other types of scanning, such as gallium 67 or indium-labeled leukocyte scans.
The use of indium-111 (111In)-labeled leukocytes for scanning increases the specificity for infection. This technique involves removal of blood by venipuncture, in vitro radiolabeling of the leukocytes, reinjection into the patient, and delayed (16 to 24 hours) scanning. Indium scans are much less sensitive in the detection of chronic infections and are not indicated for this purpose. Similar information can be obtained more rapidly, with better imaging, accuracy, and specificity and without the need for handling patient blood by the use of immunoscintigraphy, using radiolabeled monoclonal antibodies that bind leukocytes in vivo.40
The combination of 99mTc and 111In-labeled leukocytes may increase the sensitivity and specificity to over 80% for diagnosis of infection in united fractures. Labeled leukocyte localization returns to a normal pattern faster than does magnetic resonance imaging (MRI) after bone trauma, surgical procedures, and treatment of osteomyelitis; thus, the incidence of false-positive exams is significantly less.41
Magnetic Resonance Imaging
Magnetic resonance imaging provides more accurate information on the local extent of the soft tissue involvement in patients with musculoskeletal infection. The superior anatomic resolution compared with that of nuclear imaging helps differentiate bone and soft tissue infection and the extent of each. MRI has become indispensable in the delineation of many types of musculoskeletal infection, in particular the evaluation of soft tissue infections, including cellulitis, myositis, fasciitis, abscesses, and septic arthritis. In several comparative studies, MRI has been superior to scintigraphy, CT scan, and conventional radiography in detecting the presence and determining the extent of osteomyelitis. MRI visualization of the bone marrow facilitates highly sensitive detection of early osteomyelitis, although specificity for the diagnosis is aided by other findings, including cortical destruction, which may be best seen with CT scanning. The overall sensitivity of MRI for bone infection approaches 100%, whereas specificity is 60 to 75%.39,42 Use of gadolinium enhancement with MRI improves the ability to distinguish abscess from cellulitis or myositis. MRI may facilitate differentiation of acute from chronic osteomyelitis and may help to detect reactivation of old infection in the presence of chronic inflammation or other posttraumatic lesions.43
A retrospective review of MRI studies has found that its overall specificity regarding the diagnosis of bone involvement can be improved to over 80% without significant loss of sensitivity if increased marrow signal intensity on T2-weighted images is included as an additional criterion in the diagnosis of bone infection. As now practiced, evidence of osteomyelitis on MRI consists of abnormalities of the bone marrow with decreased signal intensity on the T1-weighted images and increased image sensitivity and enhancement as seen with the T2-weighted or short T1 inversion recovery (STIR) images (Fig. 3.5).37 Acute activity in chronic osteomyelitis can be excluded with a high probability if the MRI findings are negative.44

Many prospective studies have compared MRI with three-phase bone scanning and with indium-labeled WBC scanning (111In-WBC scanning) to diagnosis acute infection. Patients were evaluated at presentation with all three modalities; the sensitivity of each modality was calculated using additional biopsy as the gold standard. The sensitivity of MRI is consistently 90% or greater, compared with 70% for bone scan and 45% for indium scan. MRI should be used to evaluate patients with positive bone scintigraphy to improve the specificity and accuracy of diagnosis for osteomyelitis.45 It permits the differentiation of septic arthritis or cellulitis from osteomyelitis.
Positron Emission Tomography
Scanning with positron emission tomography (PET) with the use of fluorine 18–labeled fluorodeoxyglucose (FDG) is becoming more frequently used in the diagnosis of infection. It has high sensitivity and specificity but is not widely available. FDG-PET has been found to accumulate nonspecifically at sites of infection and inflammation. Investigational studies showed that PET is particularly valuable in the evaluation of chronic osteomyelitis and infected prosthesis. However, the use of this modality for the diagnosis of acute infection following fracture surgery has yet to be determined.36
Cultures
Specific bacteriologic diagnosis requires positive culture of pathogens from biopsy material. Swabs of drainage from cutaneous fistulas or open wounds are not adequate, nor are specimens obtained by needle biopsy.46 Multiple specimens from different sites and tissues should be obtained in surgery and sent to the laboratory for aerobic and anaerobic culture. Selected patients, such as those with compromised immune status, should have specimens analyzed for fungi or mycobacterium. It is useful to obtain an immediate Gram stain of the culture material, both for the early institution of therapy and to ensure prompt processing of the specimen, which will result in better recovery of organisms.
Staging and Classification
Cierny et al47 developed a useful classification for the staging of chronic osteomyelitis according to the anatomy of the involved bone and physiological status of the host. They identified four anatomic types:
Type 1 is a medullary osteomyelitis and is primarily an endosteal process. It denotes infection confined to the intramedullary surface of the bone.
Type 2 is a superficial infection that involves only the outer portion of the cortex. This occurs when a contiguous focus of infection exposes bone.
Type 3 is a localized osteomyelitis involving cortical sequestration, with cavitation extending into the medullary cavity, and combines features of types 1 and 2.
Type 4 is a diffuse osteomyelitis with a permeating, circumferential, and through-and-through lesion with extensive involvement of the medullary cavity and often involves an entire segment of bone. All infected fractures and nonunions are considered type 4 osteomyelitis.
Physiologically, patients are classified as hosts as follows:
Type A hosts have a competent immune system, with normal physiology and metabolism and good vascular supply to the affected area.
Type B hosts are compromised locally or systemically, or both. Examples include those patients taking corticosteroids and patients with peripheral vascular disease.
Type C hosts would not benefit from treatment.
The clinical stage of osteomyelitis is determined by combining the anatomic “type” of involvement of disease with the physiologic health of the host. Most patients with posttraumatic osteomyelitis are classified as having Cierny stage 3 or 4 disease. A chronic wound infection can lead to stage 2 osteomyelitis. Patients with infected medullary rods or nails and an intact, healed, stable tibia have stage 1 osteomyelitis.
A variety of other characteristics can be used to classify musculoskeletal infection following trauma, for the purposes of making treatment decisions or prognostic estimations. Fracture healing versus nonunion can be very important with regard to treatment options. If the patient has undergone internal fixation, the hardware construct may be stable or unstable. The temporal relationship from surgical intervention to the development of symptoms helps to define the etiology and severity of the infection. The infection can be described as acute (within the first 2 to 3 weeks), subacute, or chronic. Local infection at the site of surgical intervention that manifests itself within 14 days of internal fixation is usually confined to the soft tissues only.27,30
Treatment
Principles of Antibiotic Therapy in Musculoskeletal Infection
Empirical antibiotic coverage is begun after obtaining samples for culture, utilizing a broad-spectrum drug such as cefazolin. Culture results should be used to guide definitive antibiotic therapy. Staphylococcus aureus is the most common pathogen,48 although Staphylococcus epidermidis and gram-negative organisms are increasing in frequency. Antibiotic resistance to penicillin is common, and resistance to the antipenicillinase penicillins (methicillin, oxacillin) is increasing. Vancomycin resistance has been reported in Staphylococcus but it is rare thus far. To preserve the efficacy of vancomycin, it should only be used in documented cases of methicillin resistance, and combination drug regimens should be considered to increase efficacy. Rifampin is a commonly used supplementary drug that can be given orally, but when used alone can lead to rapid development of resistance.48,49 Quinolone antibiotics have become popular for oral treatment of osteomyelitis due to gram-negative enteric organisms in adult patients,48 but failure is more common in cases caused by Pseudomonas aeruginosa or S. aureus. For S. aureus associated with orthopaedic implants, combination with oral rifampin is promising.50 It is important to be aware that there is experimental evidence from a rat model that fluoroquinolones, specifically ciprofloxacin, inhibit bone healing.51 In cases of unusual, multiple, or resistant organisms, infectious disease consultation may be useful.
The traditional duration of antibiotic treatment for musculoskeletal infection is 4 to 6 weeks. Intravenous antibiotics can be administered as an outpatient or at home via a peripherally inserted central catheter (PICC) line. Recently, a shorter course (< 2 weeks) of intravenous (IV) antibiotics followed by oral administration for 4 to 6 weeks has been proposed as an equally efficacious regimen in adult osteomyelitis.52
The treatment of infection following stable osteosynthesis using irrigation/drainage and antibiotics can be successful in a high percentage of patients. One study of 20 osteomyelitis patients treated with IV teicoplanin with and without oral ciprofloxacin or rifampin, followed by oral antibiotic therapy for an average of 28 weeks (range, 12 to 64 weeks), was successful in 100%.53 In another study of treatment of infected orthopaedic implants (joint replacements as well as fracture fixations), only oral antibiotics consisting of rifampin and ofloxacin were used. A successful outcome was achieved in 74% of the 47 patients.50
Pin- or Wire-Site Infection
The most common complication associated with the use of external fixation is pin-tract infection. The etiology of pin-tract infection is multifactorial and includes soft tissue impingement or tethering, poor insertion technique, inadequate site care, and loosening of fixation. Multiple studies have documented the relationship between pin loosening and pin sepsis. Stabilizing the pin–bone interface can reduce the prevalence of pin-related complications. Pins in cancellous bone frequently loosen over time, whereas fixation pins in cortical bone can remain intact and infection free for extended periods of time. Each pin in a fixation construct should be continually evaluated for these potential problems to avoid an unstable fixator.54,55
Radiographic evidence of pin loosening includes cortical rarefaction and lucency, which typically occur initially at the near cortex (Fig. 3.6). The occurrence of pin-tract lucency is reduced by hydroxyapatite coating or by the use of titanium pins, which have demonstrated a marked improvement in their holding power (torque on removal) when compared with stainless steel pins.55 Histological examinations of multiple retrieved pin specimens found that tight pin tracts are characterized by a lack of bone remodeling, whereas loose pin tracts are characterized by extensive bone resorption and inflammatory infiltrates. However, it should be noted that pin-tract infection can occur without mechanical pin loosening.

Pin insertion technique is important to improve the initial pin torque resistance and minimize loosening. Correct technique begins with a generous skin incision directly at the site of pin insertion. This is followed by gentle blunt dissection to the periosteum, which is incised as well. A small Penfield-type elevator is used to gently reflect the periosteum off the bone at the site of insertion. With this technique, extraneous soft tissue tethering and necrosis are avoided. A trocar/drill sleeve assembly is advanced directly to the bone. A pilot hole is predrilled, and the pin is inserted by hand to the correct depth. Any soft tissue tethering should be released with a small scalpel following pin insertion.
The use of self-drilling, self-tapping pins results in less purchase in the bone compared with the purchase achieved with the predrilled pin technique. Frequently, there is a tendency to increase the depth of insertion when using self-drilling pins to achieve comparable pin purchase. This can lead to more soft tissue invagination into the pin tract.54 Excessive heat generation during insertion can lead to bone necrosis (“ring sequestrum”) and early loosening. Temperatures in excess of 55°C can occur during the insertion of self-drilling pins. Self-drilling pins have shown increased microfracture of both cortices with increased bone absorption, decreased pullout strengths, and insertion torque.56,57
Pin- and wire-site complications are now graded by the classification described by Dahl et al.58 A grade 0 pin site appears normal and requires only weekly pin care. Grade 1 infections show marginal inflammation; however, no drainage is apparent, and treatment requires more frequent pin care consisting of daily cleansing with mild soap or half-strength peroxide and saline solution (Fig. 3.7). Grade 2 pin-tract infections consist of an inflamed pin site with serous discharge. Grade 3 pin-tract infection consists of an inflamed pin site with purulent discharge. Both grade 2 and grade 3 pin-tract infections require oral antibiotics and continuing daily pin care. Grade 4 pin-tract infection consists of serous or seropurulent drainage in concert with erythema, inflammation, and radiographs demonstrating osteolysis at both the near and far cortices. Once osteolysis is visible, demonstrating bicortical involvement, the offending pin should be removed immediately, with reinsertion at another site if the pin is required for frame stability. Local soft tissue debridement of the pin tract with peroxide or other astringent irrigant may be performed. Formal surgical management is unnecessary as long as there are no obvious radiodensities noted on the plain X-rays at the site of osteolysis. Grade 5 pin-tract infection consists of inflammation, purulent drainage, and osteolysis, as well as sequestrum noted or a Brodie′s abscess within the medullary canal. At this point deep-seated infection is present and requires formal irrigation and debridement procedures in concert with culture-specific antibiotics. Pin exchange should be performed in conjunction with the pin removal process (Fig. 3.6).59,60

There is no consensus in the literature as to the appropriate regimen for pin-tract care and infection prevention. A recent intra-subject, randomized, prospective controlled trial comparing daily pin-tract care to no pin-tract care was undertaken.61 Outcome measures included soft tissue interface integrity, stability of the pins, torsional stability as determined with a torque meter, pin-site osteolysis, and pain. There were no statically significant differences between the two groups (pin care versus no pin care) when comparing granulation tissue and pin-site drainage (36% vs 35%), pin stability (20 vs 25 pins with loosening), osteolysis (7 vs 6 pins), or torque on extraction (mean 0.75 Nm and maximum 3.05 Nm vs mean 0.60 Nm and maximum 3.55 Nm). This study suggested that routine pin-tract care is unnecessary as long as daily hygiene for the patient and frame is maintained.61 However, doing nothing may not be the best recommendation for the overall management of these issues, especially when treating a noncompliant patient population.
Thus, a universal standard for pin care has yet to be identified. Pin-site recommendations are based more often on clinical preference rather than on strict research findings. Ideally, a pin-care protocol should be based on the pathophysiological processes involved in the development of pin-site infection.62 It should be noted that correct pin-site insertion technique removes most of the factors that cause pin-site infection and subsequent pin loosening.21,61–65
If appropriate insertion technique is utilized, the pin sites will completely heal around each individual pin, much like a pierced earring insertion site heals. Once healed, simple showering without any other pin cleaning procedures is appropriate.24,67 The occasional removal of a serous crust around the pins using dilute hydrogen peroxide and saline may be necessary.62,64,68–72
In general, recommendations include using normal saline as the cleansing agent in concert with dilute hydrogen peroxide.62,64,71,72 Review of the Cochrane database with regard to the most effective pin-care regime was undertaken. All randomized controlled trials (RCTs) comparing the effect on infection and other complication rates of different methods of cleansing or dressing orthopaedic percutaneous pin sites were evaluated. Three trials compared a cleansing regimen with no cleansing, two trials compared cleansing solutions, one trial compared identical pin-site care performed daily or weekly, and four trials compared dressings. One of these trials reported that infection rates were lower (9%) with a regimen that included cleansing with half-strength hydrogen peroxide and application of Xeroform dressing when compared with other regimens.73,74
Additional studies have recommended the use of polyhexamethylene biguanide, silver sulfadiazine, or 10% polyvinylpyrrolidone iodine (polyod)-impregnated gauze pin wraps to reduce the risk of pin-tract infection compared with pin gauze wraps soaked in normal saline.65,75,76 Despite these studies, there is insufficient evidence for a single particular strategy of pin-site care that minimizes infection rates.73,76–79
One should avoid ointments for post-cleansing care, as these tend to inhibit the normal skin flora and alter the normal skin bacteria, and thus can lead to superinfection or pin-site colonization.73,80 It is important to remove the buildup of crusted material, which tends to stiffen the pin–skin interface and increase shear forces at the pin–bone interface. Immediate postoperative compressive dressing should be applied to the pin sites to stabilize the pin–skin interface and thus minimize pin–skin motion, which can lead to additional necrotic debris. By “training” the skin, the pin site remains stable.64,68,77 This enables the skin to heal around the pin undisturbed. Compressive dressings can be removed within 10 days to 2 weeks once the pin sites are healed. If pin drainage does develop, then pin care should be provided three times per day. This may also involve rewrapping and compressing the offending pin site in an effort to minimize the abnormal pin–skin motion.64,77
Tips and Tricks
When using external fixation, proper surgical technique for pin insertion is critical to avoid later pin-tract infection. This includes predrilling the pin sites, inserting the pins by hand, releasing the skin appropriately, and compressing the skin adjacent to the pin.
After pin placement, compress the skin around the pin site with a bandage at first, avoid ointments, and do not cleanse vigorously. Simple showering is best. If crust begins to form, it should gently be removed.
Acute or Subacute Infection with Stable Hardware
When dealing with orthopaedic implant-related infections, the knee-jerk recommendation of nonsurgical consultants is often to remove all hardware, obtain deep cultures, and administer antibiotics. This is partially correct. Cultures are helpful and antibiotics are essential, but removal of stable, functioning hardware in the setting of the acutely infected fracture should be resisted resolutely. Although it is well known that the presence of inanimate material surfaces increases the risk of infection, lowers the inoculum necessary to cause infection, and reduces the chances of successful treatment, long-standing clinical experience teaches that skeletal stability reduces the infection rate.17,81 This reduction is supported by the results of animal studies.82,83 The mechanism by which instability promotes infection is not clear but may have to do with interference with revascularization of injured tissues, ongoing tissue damage, or increased micro–dead space. Although instability seems to interfere with the resolution of infection, the presence of infection does not necessarily prohibit bone healing. A logical strategy is to maintain stable internal fixation, which will facilitate union, and plan for hardware removal later if infection persists after the bone is healed (Fig. 3.8).

For the treatment of acutely infected fractures, Berkes et al84 reported a 75% rate of fracture union and resolution of infection utilizing a standardized protocol of operative debridement, retention of stable fracture hardware, and culture-specific IV antibiotics. Factors that were predictors of treatment failure included the injury being an open fracture (p = 0.03), the presence of an intramedullary nail (p = 0.01), the patient being a smoker (high association with treatment failure), and any infection with Pseudomonas species or other gram-negative organisms.
Other authors have also identified factors that contribute to the successful salvage of acutely infected fractures. These include the maintenance of stable hardware, and that the time of surgery to infection diagnosis is less than 2 weeks.
Another factor for successful salvage is the ability to achieve a thorough debridement of the fracture construct. If a collection of pus exists around an implant or under a flap or incision, it must be thoroughly drained. Incisions made for irrigation and debridement of infection should rarely be closed and should be placed carefully to avoid exposing hardware, bone, tendon, or neurovascular structures. If these are unavoidably exposed, consideration should be given to flap coverage of the wound. The ability to achieve competent wound closure is another predictor of successful salvage. The VAC dressing (Kinetic Concepts Inc.) can be used while awaiting definitive coverage (Fig. 3.9).

Rightmire et al85 treated a cohort of patients who developed acute infection within 3 weeks of fracture surgery. An average of two surgical debridements was done prior to closure. Cases that were judged unsuccessful were those in which the hardware had failed or those in which treatment other than debridement and suppressive antibiotics was instituted (such as the use of wet to dry dressings initially). The majority of the infected fractures that failed debridement and antibiotics with retained hardware failed within 3 months from the time of initial surgery. Patients who smoked were at a significantly higher risk of experiencing failure than were nonsmokers. This study confirms what other investigators have found when treating acute fracture infection—namely that smoking is an independent predictor of failure. Smokers are estimated to have a risk of failure at least three to four times higher than nonsmokers in these studies.
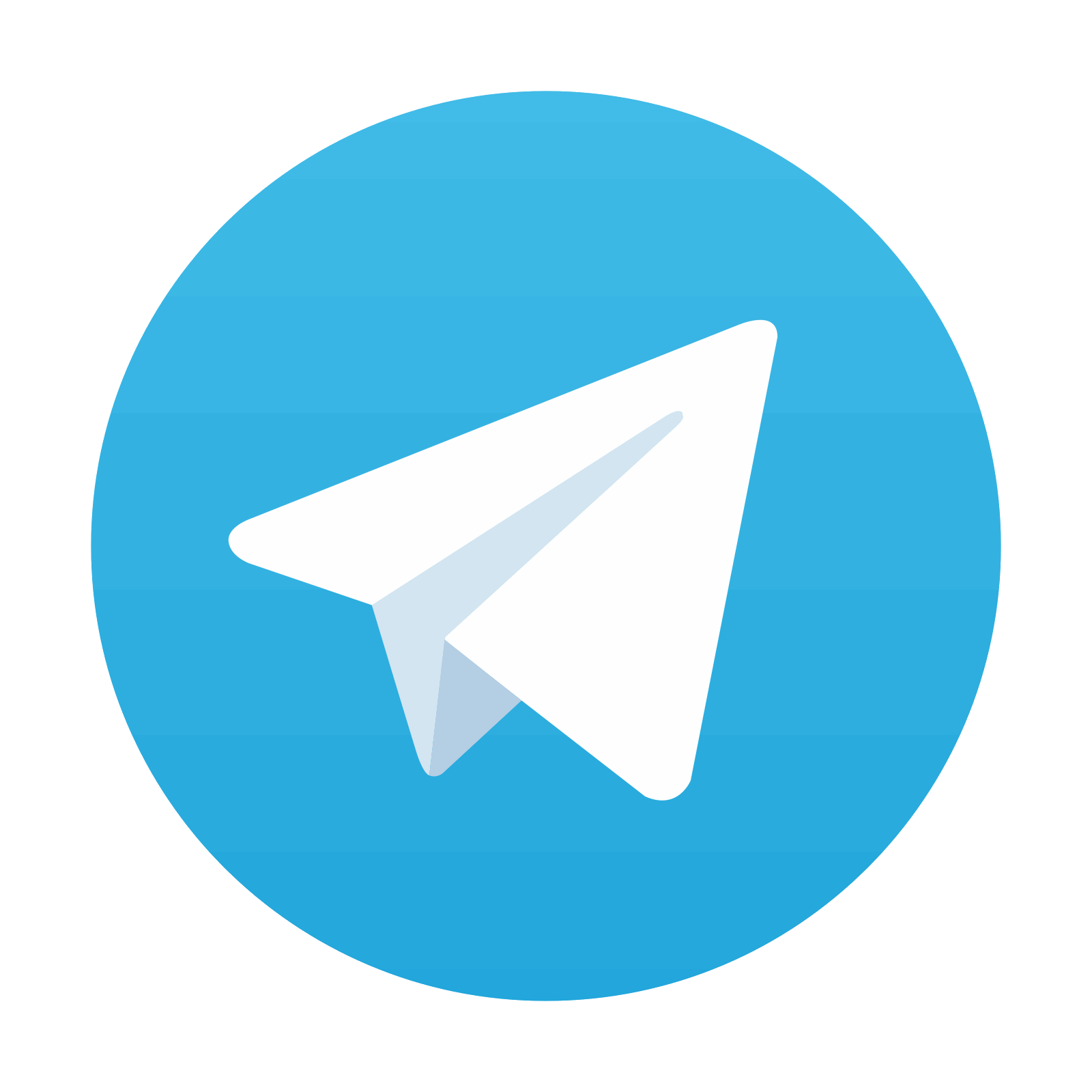
Stay updated, free articles. Join our Telegram channel

Full access? Get Clinical Tree
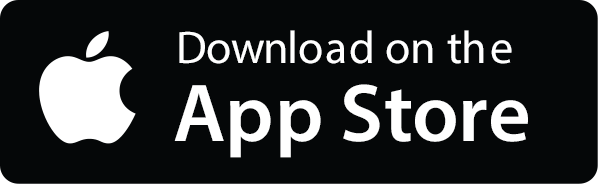
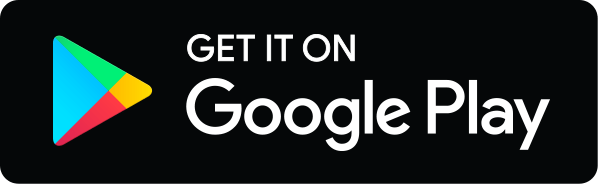
