Fig. 7.1
Schematic diagram of possible mechanisms for muscle relaxation. One possible mechanism is shown on the left side: Increases in intracortical inhibition would contribute to decreased cortical excitability in the target muscle. On the right side is another possible mechanism: A different corticospinal pathway from the one that provides an excitatory influence on spinal motoneurons contributes to muscle relaxation
TMS can be used not only to investigate the excitability of the corticospinal pathway, but also to evaluate the excitability of a variety of intracortical circuits. One such circuit involves the GABAergic connections in the motor cortex (short interval intracortical inhibition: SICI) (Kujirai et al. 1993). Reynolds and Ashby (1999) showed that SICI decreases during contraction, and hence the cortical excitability of the target muscle is increased. As for muscle relaxation, the SICI increased during the termination of contraction, which could contribute to decreased cortical excitability in the target muscle (Fig. 7.1 left; Buccolieri et al. 2004b: Motawar et al. 2012). On the other hand, Begum et al. (2005) found that SICI decreased prior to relaxation (thus increasing disinhibition). These authors postulated that spinal inhibitory interneurons are activated due to a decrease of SICI, and hence assist in relaxation of the target muscles (Fig. 7.1 right). After the above statement was published, a debate ensued as to whether muscle relaxation is executed via the cortex or spinal mechanisms. Recently, Motawar et al. (2012) indicated that muscle relaxation accompanies enhancement of SICI, and then induces a gradual increase of intracortical inhibition with progression of the relaxation phase. Although the latter mechanism is still being investigated, at the very least it is certain that muscle relaxation involves an increase in intracortical inhibition.
7.3.2 fMRI Studies
To elucidate the mechanism of muscle relaxation, brain activity was assessed using fMRI in several studies (Toma et al. 1999; Spraker et al. 2009). Some studies demonstrated an active role for the brain in muscle relaxation. For example, Toma et al. (1999) found that increases in the blood oxygenation level-dependent (BOLD) signal in M1 were observed during muscle relaxation as well as during muscle contraction. Areas outside M1, such as the supplementary motor area (SMA) and pre-supplementary motor area (pre-SMA), were also activated during muscle relaxation. Toma et al. (1999) compared the BOLD signals in the SMA and the pre-SMA and found that the activation volume was greater for muscle relaxation than for muscle contraction (Toma et al. 1999). Since the SMA is directly connected to M1 (Dum and Strick 1992), the inhibitory command might be derived from the SMA. Moreover, Spraker et al. (2009) demonstrated that the right dorsolateral prefrontal cortex (DLPFC) had a greater activity during muscle relaxation of the right hand as compared with muscle contraction in this hand. Previously, it was demonstrated that BOLD activation in the DLPFC increased during a Go/No-go task (Garavan et al. 1999; Nakata et al. 2008). The Go/No-go task has been widely utilized to investigate the inhibitory processes of motor control (Waldvogel et al. 2000; Nakata et al. 2006). In the Go/No-go task, subjects respond to one cue (the Go stimulus), and they are required to not respond to another cue (the No-go stimulus). It is possible that a population of prefrontal cortical neurons on the ipsilateral side may be associated with an inhibitory mechanism during the No-go task involved muscle relaxation.
7.3.3 EEG Studies
Movement-related cortical potentials (MRCP) during muscle relaxation have enabled a comparison between the cortical mechanisms of muscle contraction and relaxation (Terada et al. 1995; Rothwell et al. 1998; Yazawa et al. 1999). Readiness potentials, termed Bereitschaftspotential (BP), occur in the SMA (Terada et al. 1995, 1999; Yazawa et al. 1998), pre-SMA (Yazawa et al. 1998) and M1 before voluntary muscle relaxation. The BP that is generated in M1 and which is associated with isotonic muscle relaxation tasks using gravity (passive flexion) has been shown to be remarkably similar to that associated with muscle contraction (Terada et al. 1995; Yazawa et al. 1999). However, Rothwell et al. (1998) suggest that the BP related to M1 is reduced in amplitude for muscle relaxation for non-movement (isometric) tasks as compared to those involved with contraction. Finally, Pope et al. (2007) directly compared muscle contraction and relaxation for both isotonic and isometric tasks and verified that the BP in M1 is greater for muscle contraction than relaxation during isometric tasks, but is similar for isotonic tasks. Therefore, they suggest that isotonic tasks require an active process for relaxation in the motor cortex, as compared with isometric tasks (Rothwell et al. 1998; Pope et al. 2007). Pope et al. also noted that the greater BP seen with isotonic relaxation tasks is related to afferent proprioceptive feedback from the muscle spindle. Thus, the neural mechanism of muscle relaxation may change depending upon whether an isotonic task or isometric task is involved.
7.4 Effect of Muscle Relaxation on the Remote Parts of the Body
Many studies have investigated how muscle contraction influences neural mechanisms of the other limb (remote effect; Baldissera et al. 2002; Tazoe et al. 2007). Because muscle relaxation is an “active process” requiring cortical activation, muscle relaxation as well as contraction might have remote effects However, to date the mechanisms of muscle relaxation that pertain during multi-limb, complex motions have been only minimally examined. Recently, Kato et al. (2014) demonstrated that muscle relaxation in one limb suppressed muscle activity in the other ipsilateral limb (Fig. 7.2). In the experiment, the participants were instructed to execute simultaneous relaxation and contraction in the ipsilateral hand and foot. Although the subjects tried to separately relax and contract their hand and foot, the EMG activity of contracted muscle in one limb became weakened when it was executed with relaxation in the other limb as compared with when the contraction was made alone. Therefore, muscle relaxation in one limb seems to influence muscle activity of the other (ipsilateral) limb.
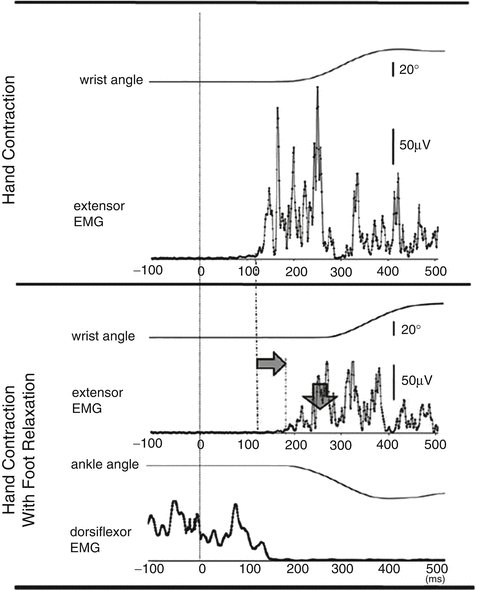
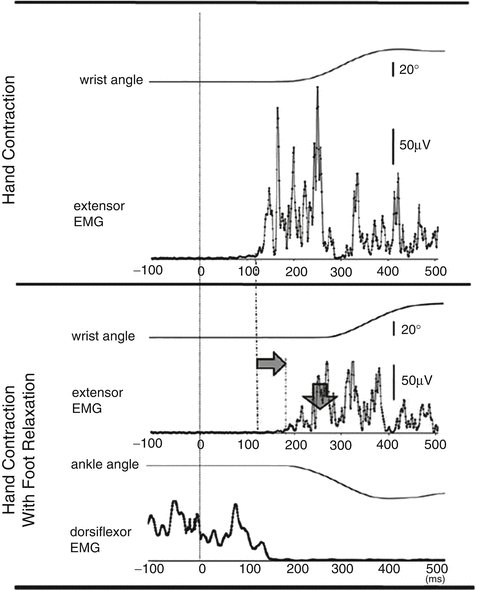
Fig. 7.2
A typical example of EMG activity (hand extensor and foot dorsiflexor) and joint angle (wrist and ankle) for a hand contraction task (top) and hand contraction with foot relaxation task (bottom) in a participant (Kato et al. 2014). Time 0 denotes the beep. The grey arrows indicate the prolonged reaction time and suppressed EMG activity
7.5 Muscle Relaxation and Sports
Not only relaxation as the cessation of contraction, but also relaxation as a state in which there is no unnecessary contraction are important to consider for sports performance. Among the sports players and musicians, it is generally acknowledged that proper muscle relaxation is absolutely necessary in the selective utilization of multiple body parts when smooth movements are required. When we try to improve a multi-limb skill that is necessary for a particular sport, we are often frustrated with the unwanted contraction of muscles which are inappropriate for a desired action. Indeed, novice sports and music players often show unintended over-contraction of relatively superfluous muscles and insufficient contraction of necessary muscles (Lay et al. 2002; Sakurai and Ohtsuki 2000; Fujii et al. 2009; Yoshie et al. 2009).
Unskilled badminton players showed continuous, unnecessary contraction of the triceps brachii when they swang a racket, whereas skilled players showed a minimal amount of unnecessary contraction (Sakurai and Ohtsuki 2000). Furthermore, a decrease in unnecessary contraction of the triceps brachii was observed following 6 days of training of the unskilled players. Fujii et al. (2009) recorded activities in agonist and antagonist muscles during tapping movements on a drum. In novice drummers, a relatively large amount of activity in the antagonist muscles was observed during contraction of the agonist muscles in the forearm. On the other hand, expert drummers were able to suppress activity in the antagonist muscles (i.e. relaxation). The suppression was even more pronounced in the world’s fastest drummer (Fujii et al. 2009). As for pianists, it was observed that execution of muscle relaxation is difficult in situations that produce anxiety or tension (Yoshie et al. 2009). For example, muscle activity in the biceps brachii and upper trapezius while playing the piano in a competition increased compared to what was recorded during a rehearsal. Moreover, strong co-contraction of agonist and antagonist muscles was observed in a concert (Yoshie et al. 2009). Strong co-contraction of antagonistic muscles deteriorates physiological efficiency and thus produces muscle fatigue (Lay et al. 2002). Not surprisingly, Yoshie et al. also reported that performance quality, relative to the rehearsal, decreased in the concert.
7.6 Future Studies on Muscle Relaxation and Sports
In summary, muscle relaxation requires a characteristic brain activation pattern just as does muscle contraction. In the applied field of body movements, moreover, although muscle relaxation is clearly essential for skilled movements, it is difficult for novice players to execute relaxation of the appropriate muscles, especially in situations that make them nervous. In most situations, skilled-players are able to simultaneously use the required muscles with an appropriate force level along with simultaneous relaxation (no or minimal contraction) of unnecessary muscles. On the other hand, when a beginner or unskilled-player tries to perform a complex motor skill, they are often frustrated by the contraction of muscles in body parts that should remain relaxed during the desired action. On such occasions, coaches are often heard to say “Relax more!” to the player. However, to simply relax the unintentionally contracted muscles is not so easy as coaches think. First, relaxation of one particular muscle during contraction of another muscle is hard to execute (e.g. badminton, drum and piano players; see previous chapter). Muscle contraction in one limb tends to induce activation of circuits controlling other limbs (Baldissera et al. 2002; Tazoe et al. 2007). Second, as noted above, when we focus on an inappropriate contraction and try to suppress it, the desired muscle contraction is likely to also be suppressed (Kato et al. 2014). This inhibitory mechanism may be associated with the simultaneous relaxation and contraction seen during active sports.
In previous studies on muscle relaxation, only mechanisms involved with muscle relaxation of a particular target muscle were analyzed. In the future, it will be important to clarify the reason why proper muscle relaxation, in the sports field, is so difficult. This problem needs to be investigated from many different perspectives.
References
Baldissera F, Borroni P, Cavallari P, Cerri G (2002) Excitability changes in human corticospinal projections to forearm muscles during voluntary movement of ipsilateral foot. J Physiol 539:903–911PubMedCentralPubMedCrossRef
Berardelli A, Hallett M, Rothwell JC, Agostino R, Manfredi M, Thompson PD, Marsden CD (1996) Single-joint rapid arm movements in normal participants and in patients with motor disorders. Brain 119:661–674PubMedCrossRef
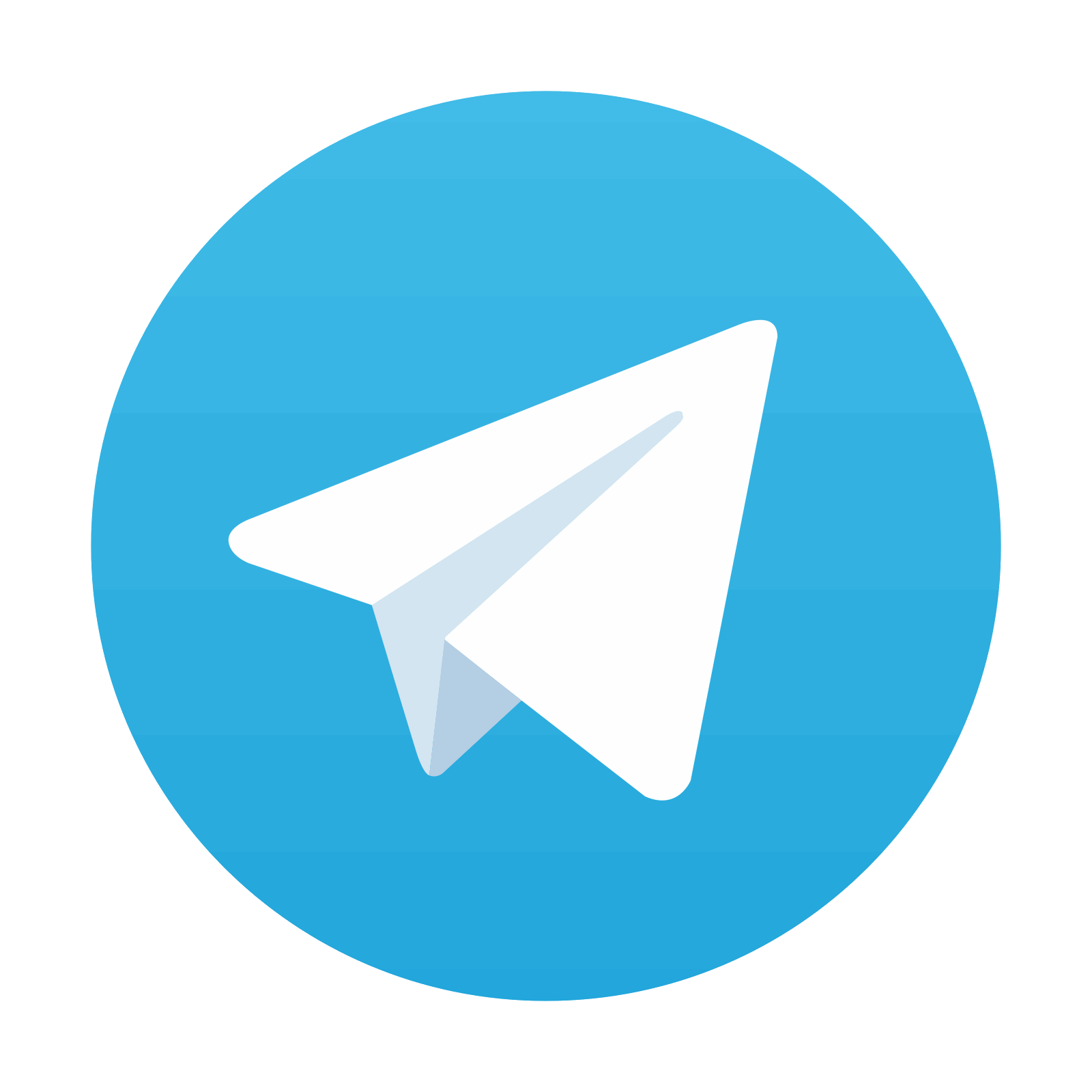
Stay updated, free articles. Join our Telegram channel

Full access? Get Clinical Tree
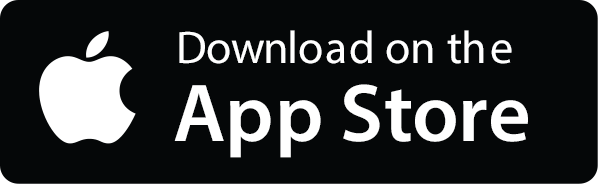
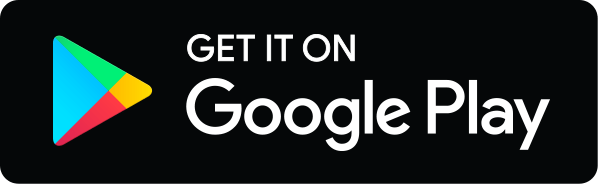