The etiology of most systemic autoimmune diseases remains unknown. There is often a preclinical period of systemic autoimmunity prior to the onset of clinically classifiable disease; established and emerging data suggest that dysregulated immune interactions with commensal microbiota may play a role in the initial generation of autoimmunity in this preclinical period. This article reviews potential mechanisms by which alterations of healthy microbiota may induce autoimmunity as well as mucosal microbial associations with autoimmune diseases. If mucosal microbiota lead to the development of autoimmunity, these mucosal sites, microorganisms, and immunologic mechanisms can be targeted to prevent the onset of systemic autoimmune disease.
Key points
- •
Mucosal microbiota can generate autoimmunity through a variety of mechanisms, including molecular mimicry, alteration of host antigens, exposure of self-antigens, bystander activation, modulation of immune reactivity, and breach of the mucosal firewall.
- •
Autoimmune disease may be triggered by a single microorganism or alterations of the host microbial community.
- •
Microbiota changes associated with autoimmune disease may represent causality or a change to the mucosal environment associated with systemic inflammation.
- •
Prospective studies that evaluate microbial changes at each mucosal site during the preclinical period of autoimmunity are needed to better understand the influence of microorganisms in the pathogenesis of autoimmune disease.
- •
If specific microbiota are found causal or protective in the development of autoimmune disease, novel strategies can be developed that may ultimately prevent disease.
Introduction
Humans live in symbiosis with greater than or equal to 10 14 microorganisms that reside on epithelial surfaces of the body, including the skin and mucosal surfaces of the respiratory, gastrointestinal (GI), and genitourinary (GU) tracts. These microbiota can be pathogenic or nonpathogenic, and they include a diverse community of bacteria, viruses, and fungi. The symbiotic nature of the host-microbial relationship implies a mutual benefit. Microbes gain access to habitats and nutrients important for their survival. Humans need microbes for certain metabolic functions as well as proper immune system development and maintenance.
Healthy individuals maintain a well-balanced microbial composition. Although the exact composition of a normal healthy microbiota is currently undefined, it is the focus of the National Institutes of Health–sponsored Human Microbiome Project. Of particular interest for this review, alterations of what may be considered normal healthy microbiota may result in inflammation and autoimmunity, termed dysbiosis . Such an imbalance may include an overgrowth or elimination of a single microbial species or changes in the relative abundance (increase or decrease) of multiple microorganisms within the community as a whole. Over the past decade, rapid advances in DNA sequencing technologies have allowed expanding knowledge of this intricate relationship and its potential association with disease. This article reviews data supporting the role of mucosal microbiota in the development of autoimmune disease.
Introduction
Humans live in symbiosis with greater than or equal to 10 14 microorganisms that reside on epithelial surfaces of the body, including the skin and mucosal surfaces of the respiratory, gastrointestinal (GI), and genitourinary (GU) tracts. These microbiota can be pathogenic or nonpathogenic, and they include a diverse community of bacteria, viruses, and fungi. The symbiotic nature of the host-microbial relationship implies a mutual benefit. Microbes gain access to habitats and nutrients important for their survival. Humans need microbes for certain metabolic functions as well as proper immune system development and maintenance.
Healthy individuals maintain a well-balanced microbial composition. Although the exact composition of a normal healthy microbiota is currently undefined, it is the focus of the National Institutes of Health–sponsored Human Microbiome Project. Of particular interest for this review, alterations of what may be considered normal healthy microbiota may result in inflammation and autoimmunity, termed dysbiosis . Such an imbalance may include an overgrowth or elimination of a single microbial species or changes in the relative abundance (increase or decrease) of multiple microorganisms within the community as a whole. Over the past decade, rapid advances in DNA sequencing technologies have allowed expanding knowledge of this intricate relationship and its potential association with disease. This article reviews data supporting the role of mucosal microbiota in the development of autoimmune disease.
Overview of mucosal immunity
The mucosal immune system ( Fig. 1 ) evolved to prevent invasion of resident and pathogenic microbes. The first line of defense is the physical barrier established by a mucus layer overlying epithelial cells. The epithelial layer is typically composed of several cell types, depending on anatomic location. Within the epithelial layer, there are secretory cells that synthesize and secrete proteoglycans to generate mucus as well as other cells that aid in microbial defense. Almost all epithelial cells produce antimicrobial proteins that are retained in the mucus layer. The GI tract, skin, and lung epithelial layers also contain intraepithelial lymphocytes (IELs) that participate in microbial defense (reviewed in Refs. ). IELs are a heterogeneous group of antigen-experienced T cells that function to protect from microbial invasion and maintain epithelial homeostasis, and similar cells may also be present at other mucosal sites. Not only do the cells of the epithelial layer participate in direct microbial defense but also they aid in communication with other members of the mucosal immune system through cytokine and chemokine production.

Just beneath the epithelial layer is a network of innate immune cells primed for antigen exposure. If microorganisms do breach the epithelial barrier, they are quickly phagocytosed by macrophages; macrophage activation leads to neutrophil, B-cell, and T-cell recruitment. Furthermore, tissue-resident macrophages and dendritic cells continually sample mucosal antigens in the lung and GI lamina propria and migrate to the mediastinal and mesenteric lymph nodes, respectively. Many of these innate cells do not circulate systemically and, therefore, compartmentalize immune responses to the mucosal system. These mucosal draining lymph nodes thus act as a firewall, which allows the host to continuously sample and respond to mucosal microbiota without generating a systemic inflammatory response.
In mucosal lymph nodes, antigen-loaded dendritic cells induce specific IgA + B cells to differentiate into plasma cells. The plasma cells then migrate back to the mucosal lamina propria and produce IgA that is transcytosed across the epithelial barrier. IgD antibodies can also be generated and secreted in response to bacteria, specifically the nasal and respiratory mucosa. These mucosal IgD antibodies are often polyreactive in response to bacteria and, of interest, can frequently be autoreactive. Also, within the draining lymph nodes of mucosal sites, T cells are activated and differentiate into one of several subtypes, in particular T-regulatory (Treg) and Th17 T-cell subsets. These activated T cells can also migrate back to the mucosa into the tissue lamina propria, where they partake in mucosal defense.
Mucosal sites also harbor lymphoid aggregates that are well organized into secondary and tertiary lymph structures, known as mucosal-associated lymphoid tissues (MALTs). For some mucosal sites, such as the GI and nasopharyngeal tract, MALT is constitutive; these include GI-associated lymphoid tissue, to which Peyer patches belong, and nasal-associated lymph tissue, which includes Waldeyer’s ring. In contrast, other areas of MALT are not preprogrammed and develop in response to inflammation or infection. Such areas include the lung mucosa that contains inducible bronchus-associated lymphoid tissue. In these lymphoid follicles, B and T cells mature after antigen exposure, differentiate, and expand.
Importantly, even in MALT that is constitutive, colonization with microorganisms is required for normal immune development. Studies using germ-free mice have demonstrated significant immunologic alterations. Secondary lymph organs are hypoplastic in germ-free mice, and the absolute numbers of innate and adaptive cells are dramatically reduced. Commensal microbes are required to prime innate cells (neutrophils, dendritic cells, and natural killer cells) for reactivity against invasive pathogens. Mature B cells and class switching from IgM to IgG or IgA requires the presence of microbiota. Finally, T-cell differentiation into Treg and Th17 cells has been shown critically dependent on specific microbial populations.
Microorganisms may initiate systemic autoimmunity
The etiology for most systemic autoimmune diseases is currently unknown, although both genetic and environmental factors likely play a role. For systemic autoimmune diseases, such as rheumatoid arthritis (RA) and systemic lupus erythematosus (SLE), data demonstrate a period of preclinical autoimmunity, during which systemic inflammatory markers and autoantibodies specific for disease are abnormally elevated in the blood years prior to the onset of clinically classifiable disease manifestations. The mechanisms as well as anatomic site(s) for the generation of preclinical autoreactivity are, however, unknown.
Advances in technologies, including DNA sequencing, have led to culture-independent nonbiased approaches that evaluate the total composition of microbiota present at a mucosal site, even in absence of overt pathologic infection. These advances have led to an increased focus on evaluating the mucosal microbial community as a whole, including consideration of the presence/increase of potentially bad bacteria as well as the elimination/decrease of potentially good bacteria. Therefore, the microbial influence on autoimmune disease can now be evaluated as potentially triggered by a single microbe or alterations of the larger microbial community.
Potential Mechanisms By Which Microbiota Could Induce Autoimmunity
Because of the significant effects microbiota exert on immune development, it is easy to speculate that dysbiosis at a mucosal site can alter immune function and result in autoimmune disease. There are several potential mechanisms by which microorganisms could shape the immune system to generate autoimmunity ( Table 1 ). These include molecular mimicry, generation of neoantigens, bystander activation, modulation of immune reactivity, and a breach of the mucosal firewall. Understanding the details of how each pathway could contribute to systemic autoimmunity at different mucosal sites may lead to novel mechanism-specific or microbial-specific targets for disease prevention.
Mucosal Immune Response | Definition | Example |
---|---|---|
Molecular mimicry | Generation of a cross-reactive antibody that recognizes shared epitopes of microbial and host tissue proteins | S pyogenes in acute rheumatic fever |
Microbial alteration of host antigens | Microbial-induced modification of host proteins creating neoantigens | P gingivalis and citrullinated proteins in RA |
Microbial-induced exposure of self-antigens | Externalization of previously intracellular self-proteins that generate autoimmune responses | Bacterial-induced NET formation and citrullinated proteins in RA |
Bystander activation | Mucosal responses to microbiota producing inflammatory cytokines that spill over and activate nearby autoreactive cells | S aureus and ANCA-associated vasculitis |
Modulation of immune reactivity | Specific microorganisms shaping the development of the host immune system | Clostridia species influence mucosal Treg cell development |
Breach of the mucosal firewall | Mucosal-limited immune responses to microbiota that aberrantly move beyond the mucosa | Antigliadin antibodies in celiac disease |
Molecular mimicry
Molecular mimicry is the development of an antibody that cross-reacts with a microbial protein as well as normal host tissue. A classic example of molecular mimicry triggering autoimmunity is acute rheumatic fever. In acute rheumatic fever, T cells respond to a specific peptide epitope of S treptococcus pyogenes . During pharyngitis with S pyogenes , these specific T cells stimulate B-cell generation of a cross-reactive antibody to human cardiac myosin, resulting in acute rheumatic fever–associated carditis. Because not all infections with S pyogenes result in rheumatic fever, genetics and the specific site of infection seem to play a critical role in generating an autoimmune response. Although acute rheumatic fever is a well-established example of molecular mimicry, such a process may occur in the pathogenesis of other autoimmune diseases.
Microbial alteration of host antigens
Some microbes are able to modify host proteins, thereby creating neoantigens that are recognized as foreign to the adaptive immune system and could generate autoimmunity. For example, Porphyromonas gingivalis is uniquely found to express a peptidylarginine deiminase (PAD) enzyme capable of citrullinating host proteins (eg, fibrinogen and α-enolase). By generating citrullinated fibrinogen and α-enolase, neoantigens are generated that can bind with high affinity to major histocompatibility complex class II HLA-DR4 shared epitopes. Therefore, these protein alterations by P gingivalis could potentially lead to autoimmunity in RA in the form of antibodies to citrullinated protein antigens (ACPAs).
Microbial-induced exposure of self-antigens
In RA and SLE, autoantibodies can be generated to intracellular self-proteins, although these proteins must first be externalized to generate an immune response. Such a phenomenon can occur at the mucosal surface with the induction of neutrophil extracellular traps (NETs). NETs are a mechanism by which neutrophils decondense and expel their chromatin in response to inflammation or bacteria. For example, during NET formation, antibacterial and citrullinated proteins that were previously intracellular are now externalized, and these citrullinated proteins may trigger mucosal ACPA responses. In addition, specific bacterial species can have differential effects on inducing NETs (eg, Lactobacillus rhamnosus inhibits NET formation ). Therefore, certain microbial changes can affect the rate of NET formation and as such may be associated with increases or decreases in generation of autoimmunity.
Bystander activation
Microbial infection at a mucosal site results in activation of innate and then adaptive immune responses. Both microbe-specific and nonspecific B and T cells are recruited to an inflamed mucosal site. Bystander activation occurs when the cytokines of the inflammatory milieu activate the nonspecific adaptive cells in addition to the microbe-specific B and/or T cells. Alternatively, some bacteria express superantigens that can activate T cells in a T-cell receptor–independent manner. In such a fashion, autoreactive B or T cells can be activated, partake in tissue damage, and perpetuate autoimmune disease. One hypothesized example of bystander activation is the association between Staphlococcus aureus and antineutrophil cytoplasmic antibody (ANCA)-associated vasculitis. Methylation motifs within S aureus DNA can activate ANCA-producing B cells via Toll-like receptors (TLRs) in patients with ANCA-associated vasculitis. Similarly, bacterial and viral DNA can signal through TLRs, generating exacerbated type I interferon (IFN) responses and polyclonal B-cell activation in patients with SLE.
Modulation of immune reactivity
As discussed previously, commensal microbiota have a significant impact on immune maturation, and specific bacteria can modulate immune responses. For example, selected Clostridia species increase mucosal Treg cell development whereas segmented filamentous bacteria (SFB), also members of the Clostridia family, allow generation of Th17 cells. SFB or similar species, however, have not been identified in humans by sequencing studies. Furthermore, one microbial species may affect development of T cells in different compartments. Intestinal polysaccharide antigen A from Bacteroides fragilis promotes splenic Th1 responses whereas in the intestinal lamina propria it promotes Treg cells. Therefore, changes in commensal microbiota can have significant impacts on immune reactivity both locally and systemically; these effects may diverge based on immunologic site. It can be projected that these and other microbiota changes, either single organism or community alterations, may be sufficient in the setting of genetic predisposition to shape the immune system, resulting in autoimmunity.
Breach of the mucosal firewall
As discussed previously, inflammatory reactions are generally limited to the mucosa during the body’s natural response to environmental antigens and microbes. This represents a mucosal firewall within which mucosal responses are contained and do not move beyond the regional lymph nodes. If this mucosal firewall is compromised, however, immune responses that once were limited to the mucosa could become systemic. For example, in celiac disease, antibodies to gliadin that are generated in response to the presence of gliadin (a wheat protein) in the gut can become systemic rather than remaining contained within the gut mucosa.
Furthermore, in localized mucosal immune responses to microbiota, autoantibodies are generated locally as part of the body’s natural immune response and can serve as broad protection against microorganisms. Such a phenomenon has been demonstrated in the lung mucosa because autoantibodies, including rheumatoid factor and antinuclear antibodies, have been found in airway sections in response to bacteria. Furthermore, in a study by Willis colleagues that evaluated ACPA responses in the lung, arthritis-free subjects at risk for future RA had sputum RA-related autoantibody positivity found generated in the lung. Some individuals, these natural mucosal-generated autoantibodies may become systemic, perhaps related to genetic or other environmental factors.
Although there are several different mechanisms by which microorganisms could generate autoimmunity, further study is needed to determine the role of each in the development of specific autoimmune diseases. As such, prospective natural history studies are critical to define the specific microorganisms, mechanisms, and additional factors involved in disease pathogenesis.
The Role of Mucosal Microbiota in Specific Systemic Autoimmune Diseases
Rheumatoid arthritis and mucosal microbiota
Mucosal dysbiosis has long been suggested as playing a role in the development of RA, and numerous microorganisms resident to a variety of host mucosal sites have been linked to RA pathogenesis. Therefore, unlike rheumatic fever in which a single bacterium has been found to trigger disease, the connection of mucosal microbiota and the development of RA is likely more complex. Some studies have identified associations of RA and viruses, such as Epstein-Barr virus (EBV). A majority of associations of microorganisms and RA have focused on bacteria, including older studies that demonstrated associations between RA and Mycoplasma, Proteus , and Escherichia as well as a study by Eerola and colleagues that identified the total composition of gut microbiota differed in newly diagnosed RA compared with controls.
In more recent studies, Scher and colleagues demonstrated that patients with recent-onset RA had an increased abundance of Prevotella and a decreased abundance of Bacteroides compared with subjects with chronic treated RA, those with psoriatic arthritis, and healthy controls. This study further identified Prevotella copri as the most abundant Prevotella species present in a majority of patients with recent-onset RA. Murine gut colonization with P copri led, however, to increased severity of colitis and not increased susceptibility for inflammatory arthritis. In accordance with Koch postulates, if a microbe is causal for disease it should be able to cause disease when introduced into a healthy organism. Although this may suggest P copri is not causal for RA, it may also represent the difficulty of applying Koch postulates if disease due to dysbiosis is a community effect and not a single species. Other human RA studies have identified different patterns of gut dysbiosis associated with RA. For example, Liu and colleagues found an increased abundance of fecal Lactobacillus in RA, and Vaahtovuo and colleagues demonstrated decreased Bacteroides in RA compared with controls.
Investigations of RA and microbiota have also placed significant focus on the oral mucosa because RA patients have a significantly higher prevalence and severity of periodontal disease. Specific focus has centered on P gingivalis because, as discussed previously, it is capable of generating neoantigens, such as citrullinated α-enolase, due to its unique expression of PAD. Studies have found ACPAs to human citrullinated α-enolase that are specific for RA and are also reactive to citrullinated bacterial α-enolase, suggesting the possibility of molecular mimicry. Furthermore, elevations of antibodies to P gingivalis were associated with RA-related autoantibody elevations in subjects in the preclinical period of RA, suggesting a possible role in initiation of autoimmunity. De Smit and colleagues, however, recently demonstrated elevations of anti– P gingivalis antibodies in preclinical RA subjects with arthralgias did not predict future onset of inflammatory arthritis.
Furthermore, recent studies suggest that variation in the oral microbiome and P gingivalis are associated with severity of periodontal disease and not specific for RA. For example, Mikuls and colleagues found that serum anti– P gingivalis antibody levels were similar in patients with RA and controls, but they were significantly elevated in those with periodontal disease. Similarly, Scher and colleagues evaluated subjects with new-onset RA and found that subgingival microbial communities were different between RA and controls, but this difference was explained by the presence of periodontal disease and was not specific to RA. This study reported alternative bacterial associations with RA, including increased abundance of Prevotella and Leptotrichia , as well as Anaeroglobus geminatus that was specifically associated with RA-related autoantibody positivity. Therefore, it may be that the association of periodontal disease and P gingivalis in RA does not trigger autoimmunity, but periodontal disease–associated inflammation and/or P gingivalis –related PAD activity may generate local citrullinated peptides in the gingival tissue, which are targeted by autoantibodies generated elsewhere. Further studies are needed to determine how each of these factors contributes to the pathogenesis of RA.
In addition, the lung mucosa has been suggested as playing a role in the development of RA. Lung microbiota have a more limited repertoire than other mucosal sites, but lung dysbiosis has been associated with chronic diseases, such as asthma and emphysema. Previous studies have identified associations between RA and Mycoplasma pneumonia . Also, heat shock proteins (HSPs) have been suggested as a potential cross-reactive antigen associated with bacterial infection and synovitis in RA, and recent studies identified an association of serum anti-HSP antibodies and RA-related lung disease. Therefore, lung microbiota may also play a role in development of RA-related autoimmunity, but additional studies are needed.
Spondylarthritis and microbial associations of disease
A causal relationship may exist between microbes and disease in the spondyloarthridities (SpA), in particularly reactive arthritis (ReA), during which infection clearly precedes the onset of arthritis. ReA can develop after infection with various GI and GU infections, including Salmonella , Shigella , Yersinia , Campylobacter , and Chlamydia . Bacterial products, such as strain-specific lipopolysaccharides, have been found within the synovium of patients with ReA due to Yersinia , Salmonella , and Chlamydia , suggesting the disease is due to persistent inflammation of the target tissue driven by the bacterial products. In these cases, the bacterium may generate an arthritogenic peptide via mechanisms (discussed previously), such as molecular mimicry or neoantigen formation that can be presented by HLA-B27 to CD8 + T cells, and this hypothesis is supported by bacteria-specific CD8 + T cells that are found to be expanded in the synovial fluid of patients with ReA.
In the same fashion, it has been suggested that Klebsiella pneumonia is the causative agent for ankylosing spondyloarthritis (AS), a view that is controversial. Another line of studies, using denaturing gel gradient electrophoresis, failed to show a difference in the microbiota in a cohort of 15 AS patients with a median duration of 14 years compared with controls. More rigorous studies using 16S ribosomal RNA sequencing demonstrate microbial associations in AS may be similar to inflammatory bowel disease, a disease in which alterations within the intestinal microbial community have been identified. An abstract presented by Stoll and colleagues demonstrated that among 28 children ages 7 to 14 with SpA, there was a decreased abundance in Faecalibacterium prausnitzii , which is hypothesized to have a regulatory effect on the mucosal immune system. These studies are greatly limited, however, by population size and/or microbial analysis methodology. Again, rigorous preclinical natural history studies are essential in understanding the link between the microbiota and disease.
Lupus, Sjögren syndrome, and viral microorganisms
Microorganisms have also been hypothesized to trigger SLE and primary Sjögren syndrome, although a majority of these studies have focused on viral rather than bacterial microorganisms. Viral microorganisms, similar to bacteria, have the potential to induce autoimmune responses through the mechanisms (described previously), such as molecular mimicry, bystander activation, and so forth.
Multiple viruses have been linked to SLE, including EBV, cytomegalovirus (CMV), and parvovirus B19. In these studies, SLE patients have higher prevalence of viral antibodies and higher viral PCR levels compared with controls. SLE-associated antibodies can be elevated, however, in the serum years prior to disease onset, and the association of these microorganisms with the generation of autoimmunity in the preclinical period of SLE is unclear. In addition, for EBV, molecular mimicry and bystander activation have been suggested as viral-induced mechanisms of SLE pathogenesis. Specifically, similar amino acid sequences have been identified between an EBV peptide and SLE autoantigen, and EBV can generate polyclonal activation as well as transactivate superantigen.
In Sjögren syndrome, disease pathogenesis induced by molecular mimicry and bystander activation has also been suggested with EBV, CMV, and human T-lymphotropic virus type 1. Furthermore, increased serum type I IFN levels have been identified in individuals with SLE and Sjögren syndrome and may be related to viral induced immune responses. Also, the antigenic protein La/SSB, often targeted in patients with SLE and Sjögren syndrome, is increased in virally infected cells and involved in viral RNA processing, suggesting molecular mimicry may play a role in disease development.
Unfortunately, understanding of the human virome is limited. As more research evolves in this area, it will be interesting to see how exposures to specific commensal viruses can influence immunity similar to what is observed with commensal bacteria.
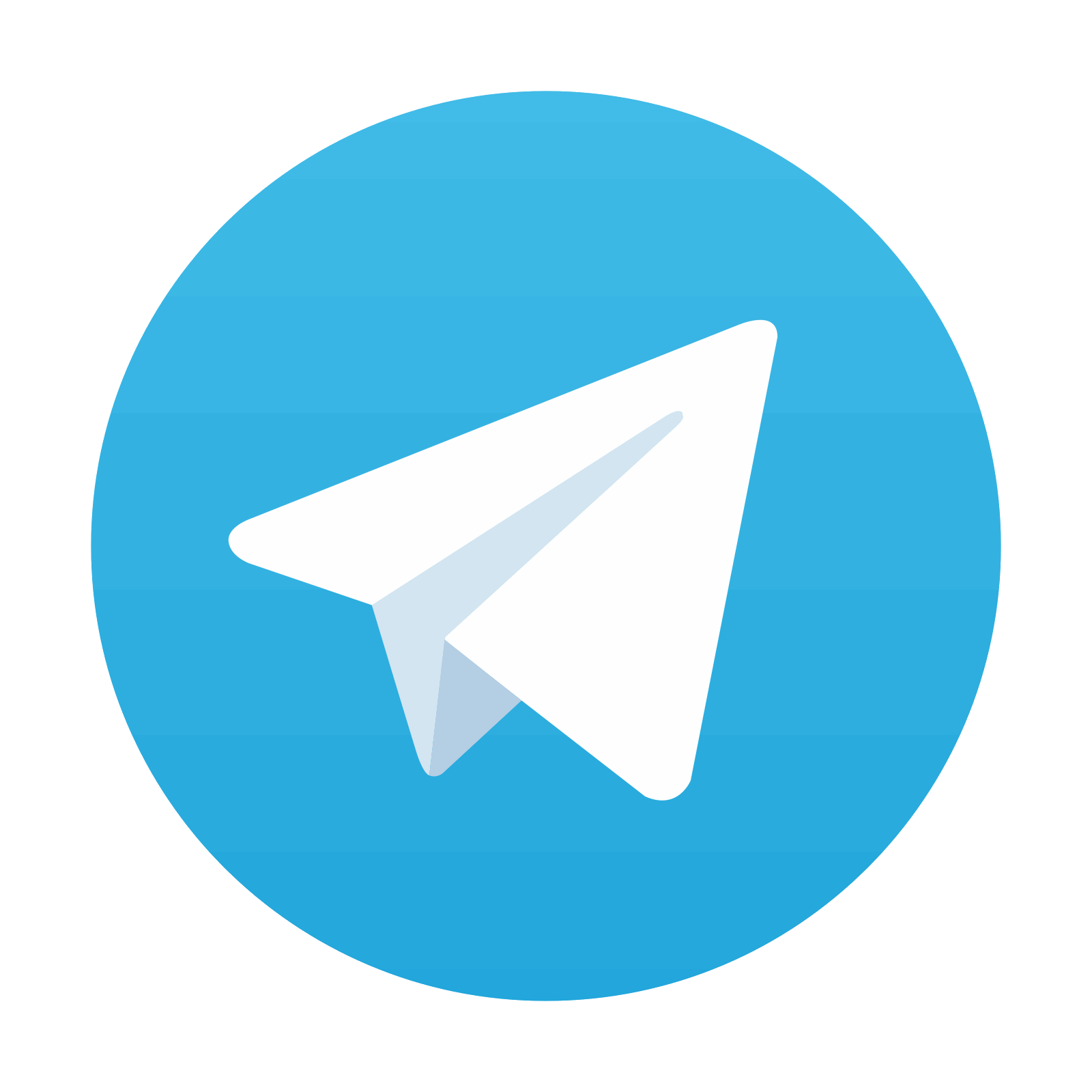
Stay updated, free articles. Join our Telegram channel

Full access? Get Clinical Tree
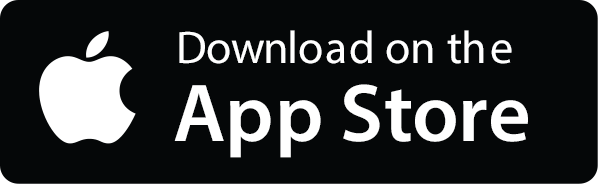
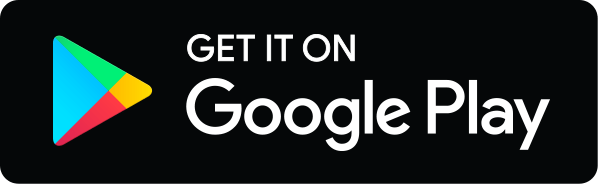