For ISS calculation only the highest value within each region can be used. For example, in a patient with two thoracic injuries assigned a 3 and 4, an extremity injury assigned a 2, and an abdominal injury assigned a 2, the ISS would not include both the 3 and 4 from the thoracic region even though they are both higher than the extremity and abdomen regions values of 2. The ISS would therefore be 24 (42 + 22 + 22), not 29 (42 + 32 + 22).
The remainder of this chapter will further investigate the imaging of a trauma patient by focusing on the different anatomic region.
3.2 Head
Head injuries are frequently encountered following blunt trauma and often lead to long term disability. Though injuries to the scalp and calvarium can require intervention, the true morbidity and mortality from head trauma occur as a result of intracranial injuries or traumatic brain injuries (TBI). Therefore, early recognition of these injuries is necessary to provide appropriate treatment to patients. One of the first true clinical assessments of intracranial injury is the calculation of the patient’s Glasgow Coma Scale or GCS score during the “D” portion of the Primary Survey [3]. The GCS score is a well validated clinical scoring system developed in the 1970s that assesses a patient’s eye opening, motor response, and verbal response on a 3–15 scale [4]. A GCS of less than 8 is typically categorized as a severe brain injury, 9–12 as a moderate, and 13–15 as minor (Table 3.1).
Table 3.1
Glasgow coma scale
Patient assessment | Score |
---|---|
Eye opening (E) | 1–4 |
Spontaneously | 4 |
To speech | 2 |
To pain | 3 |
None | 1 |
Best motor response (M) | 1–6 |
Follows commands | 6 |
Localizes to pain | 5 |
Withdraws from pain | 4 |
Decorticate posturing (Flexion) | 3 |
Decerebrate posturing (Extension) | 2 |
None | 1 |
Verbal response (V) | 1–5 |
Oriented | 5 |
Confused conversation | 4 |
Inappropriate words | 3 |
Incomprehnsible sounds | 2 |
None | 1 |
GCS also plays a key point in early decision making for head injuries. In patients presenting with a normal GCS of 15 and without loss of consciousness, amnesia to the event, physical evidence of head trauma, headache, vomiting, use of anticoagulants, neurologic deficits or use or drugs or alcohol, dedicated intracranial imaging may not be needed [5, 6].
When further investigation of a potential head injury is indicated in the early stages of trauma evaluation, axial imaging with non contrast enhanced CT is the modality of choice due to its speed and ability to identify pathologies that require intervention. MRI, PET, fMRI and other emerging imaging techniques are often too slow and not as readily available and therefore play limited role early but can be used to assess progression of certain types of traumatic brain injury [7]. Injuries to the brain are typically a result of the acceleration and deceleration forces on tissues. Epidural hematomas, subdural hematomas, intraparenchymal injuries, and skull fractures are the most common early injuries following trauma. Diffuse axonal injuries also develop though the onset is more insidious as they develop later due to fluid shifts along injured axons. These injuries are life threatening when they cause an increase in intracranial pressure potentially leading to brainstem herniation. CT findings of midline shift >5 mm, ventricular collapse due to epidural or subdural hematomas, and depressed skull fractures require immediate neurosurgical evaluation as operative methods to reduce intracranial pressure or to place devices to allow pressure monitoring to guide pharmaceutical therapies are often needed [8, 9].
Epidural hematomas typically form as the result of lacerations of arteries between the dura and the skull due to movement of the brain within the skull or injury by skull fractures. Most commonly they develop in the region that was struck during the inciting events rather than the opposite side of the brain due to a contra coup injury. They are evident on CT scans as having the classically described “Biconvex Lens” shape which forms as the blood collecting in the extradural space pushes the dura towards the brain (Fig. 3.1).
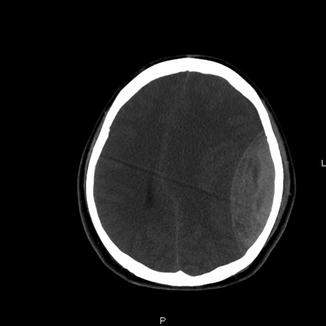
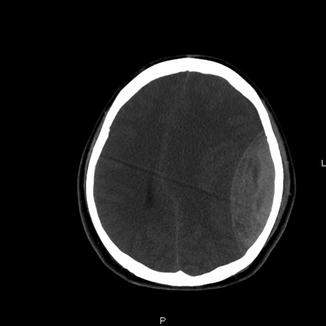
Fig. 3.1
Large left temporal epidural hematoma with ventricular compression and significant midline shift requiring early neurosurgical evaluation for potential evacuation
Traumatic subdural hematomas typically form as the result of shearing or tearing of bridging veins between the dura and the arachnoid matter. As they are a result of venous bleeding they often develop more gradually than epidural hematomas, though they still can become large enough within the fixed space of the skull to cause dangerous increases in intracranial pressure. Outcomes are typically worse with traumatic subdural hematomas due to the associated shearing forces exerted on axons resulting in diffuse axonal injuries (see below). They are evident on CT scans as a “Crescent” shaped collection of blood which pushes the dura toward the skull as it collects outside of the parenchyma of the brain (Fig. 3.2).
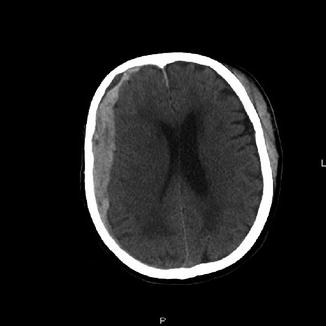
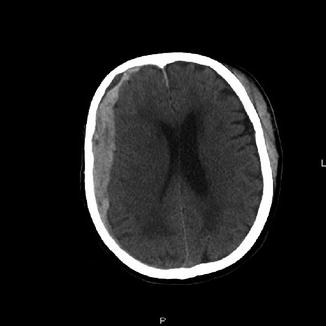
Fig. 3.2
Right fronto-temporal subdural hematoma with midline shift. Some ventricular compression is noted
Intraparenchymal injuries (i.e. subarachnoid hemorrhages) typically form as a result of injury to the brain parenchyma itself and can often “blossom” from contusions to brain matter. This can result in their formation in areas 180° from the site the head was struck resulting in a contra coup injury. They can appear as indistinct higher density areas within the brain matter itself, with larger or older intraparenchymal injuries having the appearance of distinct collections of blood within the brain matter (Fig. 3.3).
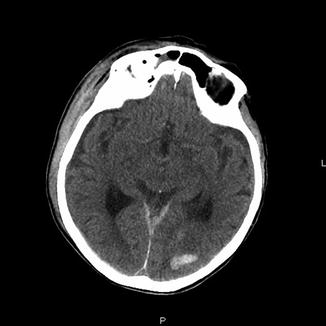
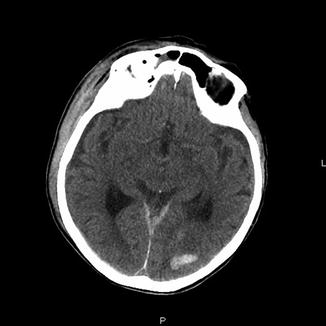
Fig. 3.3
Left occipital intraparenchymal hemorrhage several hours following motor vehicle collision
Depressed skull fractures form as a result of direct traumatic forces to the head. Fragments of skull push into the brain, often directly injuring brain tissue or causing injury to intracranial arteries or veins as previously described. Though skull fractures can be identified on plain X-ray imaging, the presence of a skull fracture necessitates further evaluation of intracranial structures. Therefore, if the clinician has a high suspicion for skull fracture, CT imaging is the modality of choice. There is little to no role for isolated plain films for head injuries if CT imaging is available. Depressed skull fractures can increase intracranial pressures directly and in combination with the associated bleeding, often requiring early surgical intervention (Fig. 3.4).
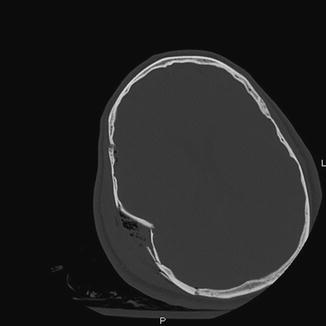
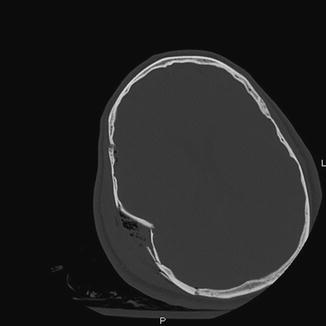
Fig. 3.4
Open right temporal comminuted depressed skull fracture with air in the subcutaneous tissue
Diffuse axonal injuries (DAI) form as a result of shearing and straining forces exerted on axons, often within areas of the brain with differing densities such as the grey and white matter interface. These types of injuries are can be associated with severe morbidity and mortality and present in up to 50 % of traumatic brain injuries. Early recognition is important as non surgical techniques to reduce swelling and optimize oxygen delivery should be employed early in the course of treatment. CT imaging is not as sensitive as conventional Magnetic Resonance Imaging (cMRI) for DAI, though a definitive diagnosis historically has been made at autopsy. Generalized cytotoxic brain edema as characterized by loss of the grey-white differentiation and scattered petechial hemorrhages can be indicators of severe DAI and seen early using CT. cMRI like CT focuses on structural abnormalities. In DAI this can be higher resolution detection of petechial hemorrhages and vastly improved detection of non hemorrhagic lesions due to edema following shear injuries [10].
Other imaging modalities find limited use in trauma. Functional imaging studies such as Positron Emission Tomography (PET) and functional MRI (fMRI) have limited clinical utility, with use primarily as tools to assess response to therapies aimed at improving cerebral perfusion. They are still primarily limited to research settings due to their cost and slow image acquisition which is often not tolerated by critically ill trauma patients. CT angiography can be used if there is concern for extension of blunt injuries to extracranial vessels which will be described in more detail later. CTAs as well as nuclear medicine brain flow do serve a role late in TBI patients as a confirmative test for brain death as evidenced by a lack of intracranial blood flow.
3.2.1 Injury Grading
The Abbreviated Injury Scale Score as related to head injuries may not relate as closely to the clinical outcome as in some other injuries. Most often injuries are based on the CT findings on initial presentation. The long term morbidity of head injuries in trauma patients is well established, though in much of the published data, the presence of a head injury and a severe ISS are often evaluated separately during multivariate logistic analyses. Injuries to brain structures or vasculature are all attributed AIS scores of 3–5 translating to a 9–25 point contribution to the overall ISS. Even clinical evidence of a TBI in the absence of anatomic injury such a clinically diagnosed concussion can carry a significant AIS score of 2–4.
3.3 Spine
In the AIS system, injuries to the spine and vertebrae are scored by the body region through which that segment of the spine traverses (i.e. cervical spine within neck, thoracic spine within chest, and lumbar spine within abdomen). In regards to imaging of these regions and principles for evaluation, injuries to the spine and spinal cord follow similar patterns and will be presented together here. Vertebral injuries in any area of the spinal column are primarily clinically significant in relationship to how they affect the spinal cord, usually defined by whether or not they are stable or unstable. Evaluation of spine stability remains somewhat controversial with one of the more widely accepted systems being that proposed by Denis in the 1980s which divides the spine into three vertical columns, the posterior, middle, and anterior columns [11]. Instability occurs with injury to 2 of 3 columns, making accurate evaluation of the middle column which includes the posterior 1/3 of the vertebral body and posterior longitudinal ligament, key in this determination. Advances in imaging continue to play a key role in this evaluation as multidetector CT has been shown to be an improvement over conventional radiographs. Flexion/Extension radiographs, along with MRI though, are better for ligamentous and soft tissue injury, and are used primarily as an adjunct to CT.
Additionally, despite the potential for severe neurologic deficits especially in the cervical spine, imaging evaluation can at times be temporarily deferred. This allows appropriate spinal precautions including a cervical collar and flat bed rest to be maintained, while other life threatening injuries (i.e. exsanguination, respiratory compromise) are addressed. Injuries to the spine are also often associated with other predictable injuries which should help guide clinical decision making when deciding when to further image the patient. Cervical spine injuries are most common, comprising over half of all injuries to the spinal cord, and are often associated with other injuries to the spine as 10 % of patients with fractures of their cervical spine will have a non contiguous spine fracture. Up to 25 % of patients with spine injuries also will have an associated TBI, which though often mild, warrants a prudent intracranial evaluation. Injuries to the spine are usually due to the effects of blunt acceleration and deceleration forces on the spinal column. As its rate of being most frequently injured implies, the cervical spine is at greatest risk due to the lack of extraneous support that the thoracolumbar spine has from the rib cage and abdomen. For this reason the cervical spine requires special attention and will be addressed first.
3.3.1 Screening
Screening evaluation of the cervical spine should be considered in essentially all patients who suffer from blunt trauma. This includes clinical evaluation as well as typically radiographic evaluation. Current recommendations from the Eastern Association for the Surgery of Trauma (EAST) Practice Management Guidelines Committee are to perform initial evaluation with CT imaging for any patient with a high risk mechanism of injury which include motor vehicle crash (MVC) at >35 mph, fall from >3 ft, any neurologic deficit, when the mechanism includes an axial load on the spine, in those 65 years of age or older, or when a neurologic deficit is present [12]. In the absence of any of these CT is still indicated if the patient has any pain, tenderness, or altered mental status during the attempt to clinically clear the patient’s cervical spine. If the CT is felt to be negative after being evaluated by a trained surgeon or radiologist, accuracies of >99 % for excluding injury are often reported, with missed injuries usually of no clinical significance. Even with normal imaging, clinical evaluation should be performed prior to cervical collar removal. In patients who have pain with active range of motion despite a normal CT scan, the practitioner can choose to perform an MRI or repeat the clinical exam in 7–10 days prior to removing the collar. In patients with abnormal cervical spine CT scans, immediate consultation with a Neurosurgeon or Orthopedic Surgeon is indicated.
As with cervical spine screening, identifying unstable thoracolumbar fractures are important due to potential spinal cord compromise, so screening imaging should be considered in many cases of blunt traumatic injury [13]. As stated before, there is a 10 % rate of non-contiguous vertebral fractures with the first detected not always being the most clinically significant. Also as with the cervical spine, EAST Guidelines recommend CT as the modality of choice for screening evaluation of the thoracolumbar spine [14]. There are several reasons for this. X-ray has consistently been found to have a relatively low sensitivity for detecting injury ranging from 25 % to 75 % compared to >95 % for CT. Additionally, patients who would require imaging of their thoracic or lumbar spine based on mechanism such as fall >10 ft, high speed MVC, motor pedestrian crashes, or those who report back pain or tenderness often also meet criteria to have further thoracic or abdominal imaging. Protocols for Chest, Abdomen, and Pelvis CT scans for trauma now can include dedicated reformats of the thoracic and lumbar spine without need for additional time or radiation exposure.
3.3.2 Unique Cervical Spine Injuries
Other unique fractures of the cervical spine include atlanto-occipital instability, C1, and C2 fractures. Occipital condyle fractures are often seen of CT imaging but frequently carry little clinical significance. Dens fractures are more often associated with spinal instability. They are classified as Type 1, involving just the tip of the dens, Type 2, involving the base (most common) and Type 3, involving the C2 body. Injury to the spinal cord at these levels can cause devastating neurologic outcomes including quadriplegia and death. Therefore early consultation with a Neurosurgeon or Orthopedic Surgeon is necessary to help determine the stability of the affected area [15]. MRI may be needed to evaluate ligamentous injury, and stabilization with either an aspen collar, halo vest device, or surgical fixation may be needed early in the course (Fig. 3.5).
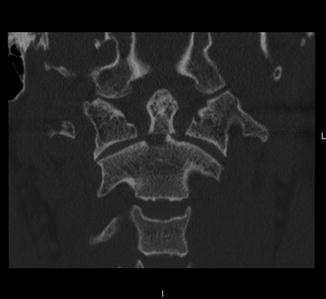
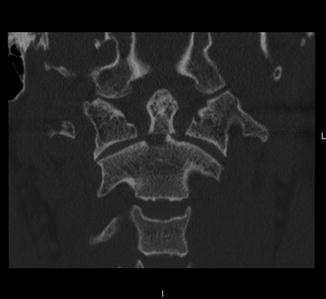
Fig. 3.5
Type 2 dens fracture with distraction ultimately requiring halo placement for stabilization
3.3.3 Compression and Burst Fractures
Compression and Burst fractures occur as the result of axial load forces on the spinal cord. The most common compression fractures are anterior wedge compression fractures which occur as a result of hyper flexion as the upper body and torso continue travel forward while the lower body is restrained during deceleration. Wedge compression fractures typically only involve the anterior column and therefore are less likely to cause spinal instability. Burst fractures are more severe as they can involve both the anterior and middle columns and can have retropulsion into the spinal canal causing direct cord compromise. They are significant based on the effect they have on alignment, vertebral body height loss, percentage of canal compromise (increasing risk of cord damage) and risk of injury to the posterior longitudinal ligament leading to instability [16, 17]. Fractures in the lumbar region are often of greater concern due to the increased axial load on these vertebrae when standing, the altered directions of the forces due to the natural lordosis of the region and lack of stabilization by the rib cage that the thoracic vertebrae have (Figs. 3.6 and 3.7).
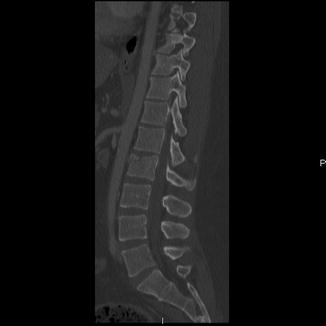
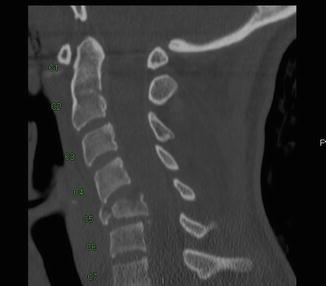
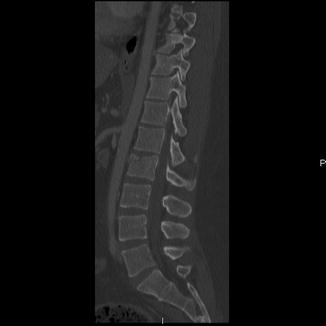
Fig. 3.6
Mildly displaced fracture of the anterior/superior endplate of L2 following a 20 ft fall
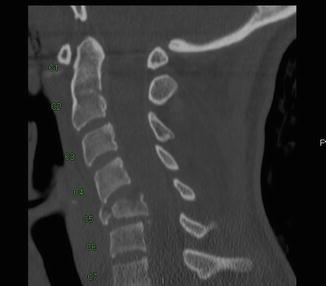
Fig. 3.7
Burst fracture of C5 following motor vehicle crash with retropulsion
3.3.4 Flexion Distractions and Fracture Dislocations of the Spine
Flexion distraction injuries as described by Denis are unstable fracture patterns due to injuries of the middle and posterior columns usually due to hyperflexion injuries with vertebral compression while the most serious fracture dislocations usually result in jury to all three columns as a result of hyperextension or hyperflexion. Chance Fractures are similar to flexion distraction injuries however a fracture line in the axial plane of the vertebral body and spinous process forms rather than a compression fracture. These bony fractures are seen best on CT imaging though, there is a low threshold for MRI to evaluate for cord injury when any potential for neurologic deficit exists (Figs. 3.8 and 3.9).
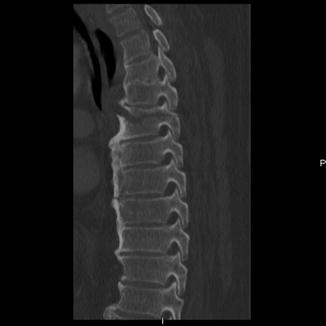
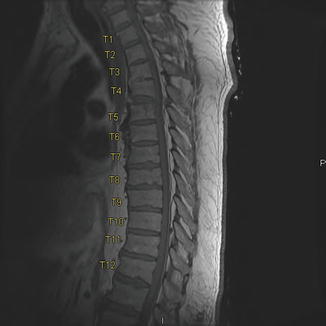
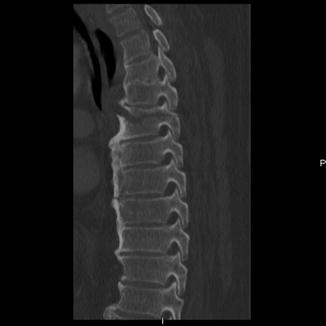
Fig. 3.8
Chance fracture at T5 with moderate displacement but no canal compromise in a pedestrian struck by a car
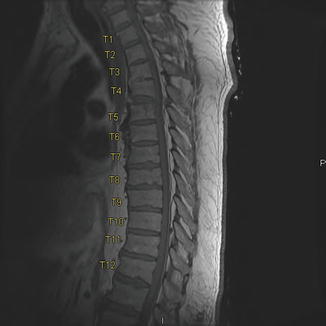
Fig. 3.9
T1 weighted MRI images of the patient in Fig. 3.8 with a T5 chance showing the focus on fat containing structures compared to bony CT windows
3.3.5 Transverse Process Fractures
Isolated transverse process (TP) fractures thoracolumbar spine are typically not clinically significant from a spinal stability standpoint and usually do not require special precautions or surgical evaluation. However, in the cervical spine, TP fractures will often include the transverse foramen. This injury pattern does not carry major significance to the spinal column or spinal cord but does warrant investigation of the vertebral artery with CT angiography (will be discussed further later in this chapter) (Fig. 3.10).
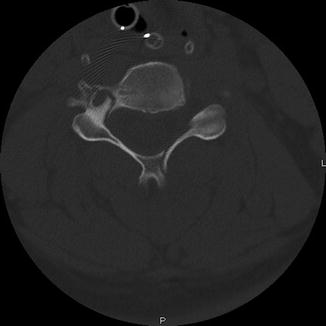
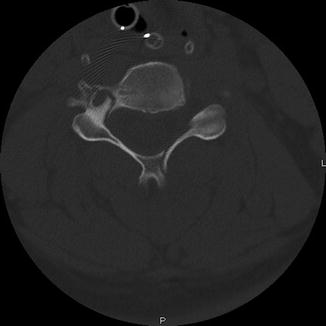
Fig. 3.10
Fracture through the right C4 transverse foramina which prompted a CTA showing occlusion of the right vertebral artery from C3–C6. A left lamina fracture can also be seen
3.3.6 Spinal Cord Trauma
Injuries to the ligamentous structures and spinal cord are not well seen on CT. Ligamentous injuries are extremely rare in the thoracolumbar spine in the absence of bony injury however persistent midline pain in the cervical spine despite a normal CT scan can warrant MRI prior to removal of a c-collar as a final method of excluding ligamentous injury. Fractures causing narrowing of the canal, clinical neurologic deficits, or unstable spine injuries seen on initial CT imaging can guide the clinician to pursue MRI imaging for evaluation for spinal cord infarcts, hemorrhages, contusions and epidural hematomas not seen on CT (Figs. 3.11 and 3.12).
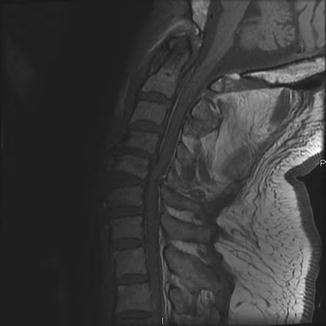
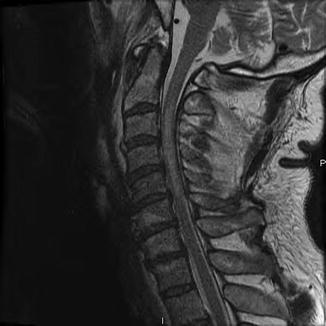
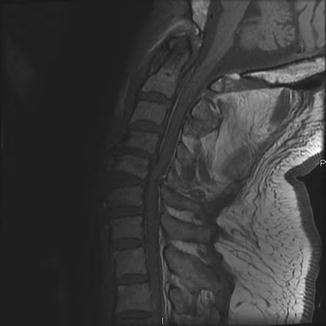
Fig. 3.11
T1 weighted MRI images showing epidural hematoma extending from C5 up to C2. This was not evident on CT but due to unexplained upper extremity weakness, and MRI was obtained
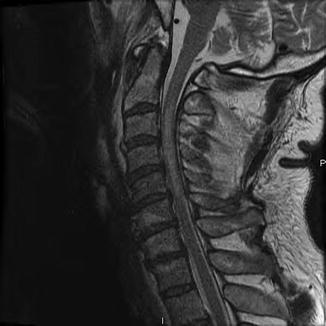
Fig. 3.12
Brightness within the cord in T2 weighted images making water bright shows cord edema in a patient with symptoms of incomplete cervical paraplegia
3.3.7 Injury Grading
As stated at the beginning of this section, injuries to the spinal column are assigned AIS scores in the area through which that portion traverses. Injuries to the brachial plexus are also included with the cervical spine. Cord injuries are assigned considerably higher values, often 4–5, regardless of the presence of a fracture based on the associated morbidity. Higher injuries (above C3) are also scored higher. Dedicated spinal cord imaging, usually MRI, is needed to assign these scores radio graphically unless CT shows obvious evidence of major cord injury. However, as imaging purely for the purposes of AIS scoring is not recommended, clinical evidence of a cord injury can be sufficient to assign an AIS score. Scores for fractures and dislocations are assigned based on imaging, however their inclusion in the patient’s ISS will only occur in the setting of no damage to the cord as the scores range from 1 to 3.
3.4 Neck
Imaging of the neck following trauma has traditionally focused primarily on the cervical spine and spinal cord as previously discussed. Major blunt injuries to aerodigestive structures are extremely uncommon and will typically be associated with external findings that will help guide further management. However, vascular injuries of the neck, especially if missed, can lead to delayed stroke or death devastating outcomes to otherwise young previously healthy patients and therefore cannot be overlooked. Rates of blunt carotid injuries of 0.3–1 % have been quoted in patients suffering from blunt trauma, therefore appropriate selected screening to identify at risk patients should be part of the evaluation algorithm for all trauma patients. Additionally, any patient with cervical transverse processes fractures that include the transverse foramen places the patient at risk for vertebral artery injury. Prior to the widespread availability of CT angiography (CTA), traditional angiograms were needed to evaluate for blunt carotid and vertebral injuries. Though highly sensitive and specific, and still the gold standard for diagnosis, they are also time consuming and expensive. CTA can be performed as a screening tool in a cost effective manner with appropriate selection criteria based on mechanism, exam findings, and associated injuries [18]. In addition to transverse foramen fractures, basilar skull fractures involving the carotid canal should also warrant CTA. Neurologic deficits on exam not consistent with other injuries, a “seat belt sign” or other abrasion to the anterior neck, severe hyperextentsion/rotation/flexion of the cervical spine especially when associated with midface or mandibular fractures and near hanging injuries also warrant further examination for vertebral or carotid injuries (Table 3.2).
Table 3.2
Blunt carotid injury grading scale
Grade | Criteria |
---|---|
I | Luminal irregularity or dissection with <25 % luminal narrowing |
II | Dissection or intramural hematoma with >25 % luminal narrowing, thrombus, or raised flap |
III | Pseudoaneurysm |
IV | Occlusion |
V | Transection with free extravasation |
If an irregularity is discovered, the Blunt Carotid Injury Grading Scale developed by the Denver Health Center has become the major system for injury scaling [19]. The injury scale originally used for the extracranial carotids are now also used to grade vertebral artery injuries. The grading system has important clinical applicability in prediction of stroke rates as well as guiding clinical treatment. Grade 5 injuries need urgent intervention and have the highest associated mortality. Grade 4 injuries have high stroke rates though often no intervention is needed and the goal of anticoagulation is to prevent propagation more than improving flow in the already occluded system. Similarly, though no intervention other than anticoagulation is immediately warranted for most grade 1 and 2 injuries they can progress to pseudoaneursyms (grade 3) which do require further intervention, though typically not urgently. Additionally, with the high resolution multidetector CT scanners providing much finer detail than has been available in the past, many times potential grade 1 injuries cannot be excluded. It is for this reason that follow up CTA is needed in grade 1 or 2 injuries 7–10 days following injury to either select patients who no longer require anticoagulation if the scan has normalized or need further intervention if progression has occurred. This is where traditional angiography still plays a role in the management of vertebral and carotid injuries, as the angiogram provides more definitive diagnostic ability as well as the potential for intervention (Figs. 3.13 and 3.14).
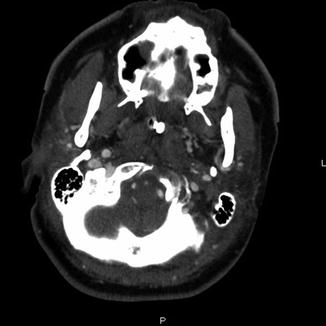
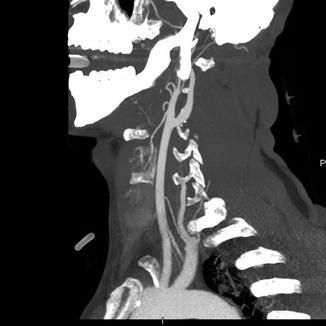
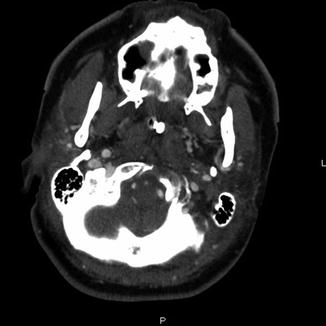
Fig. 3.13
Left internal carotid dissection with thrombus in over 50 % of the lumen on axial images of the CTA of the neck following a motor vehicle crash
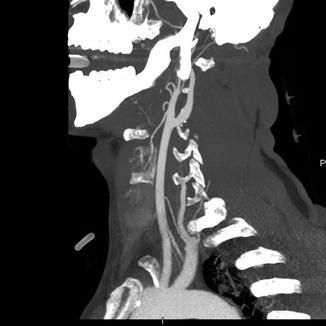
Fig. 3.14
Reconstructions of the CTA of the neck from the same patient presented in Fig. 3.10, showing an abrupt cutoff of the right vertebral artery at C6
3.4.1 Injury Grading
The AIS grading for extracranial carotid and vertebral injuries are scored in the neck section. Though the AIS system does not perfectly correlate with the Blunt Carotid Injury Grading Scale CTA or angiographic findings do help with injury scaling. Also, as with spinal cord trauma, the presence of neurologic deficits also can increase the AIS score for a specific arterial injury. Venous injuries, also included in the system are of more concern in non accidental and penetrating trauma. Lastly, though not described in detail here, injury to other structures of the neck including the thyroid and salivary glands, laryngeal and pharyngeal structures, and hyoid bone are also scored in this section.
3.5 Face
Imaging of the face is typically guided by physical exam findings consistent with facial trauma. Swelling, ecchymosis, and deep lacerations can all indicate injury to underlying structures. In general, imaging focuses on bony structures of the face. If injury to cranial nerves or branches are suspected based on physical exam findings, the mechanism of injury will often play the largest role in deciding if operative exploration (for lacerations) or a watchful waiting approach (for blunt contusions) is taken. Bony injury involving a specific foramen can additionally guide the decision making process. As with many other systems, CT scans are the primary imaging modality. However, using specific programs, 3D reconstructions can be generated using standard CT technology which can greatly aid surgeons in determining the need for facial reconstruction. The fracture patterns of the face are complex and beyond the focus of this chapter, however the principles for using imaging to help decide when intervention is warranted is based on determining stability and cosmesis which requires consultation with a surgeon with training in facial trauma. Likewise, injuries to the orbital walls which lead to intrusion into the orbit or cause retro bulbar hematomas may indicate the need for immediate ophthalmologic consultation. Dedicated dental imaging, when needed, continues to rely on plain film X-ray, though dedicated imaging machines are necessary. The ordering practitioner should keep in mind that in many institutions, consultation with Oromaxillofacial Surgeons or Dentists may be needed to interpret these images as some radiologists will not read these types of films (Figs. 3.15, 3.16, and 3.17).
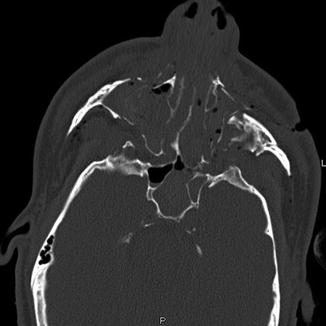
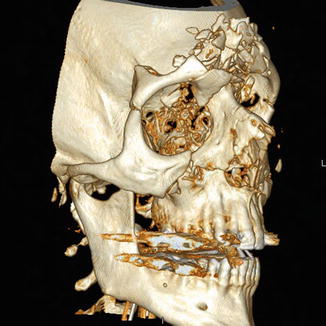
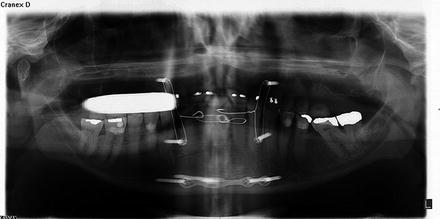
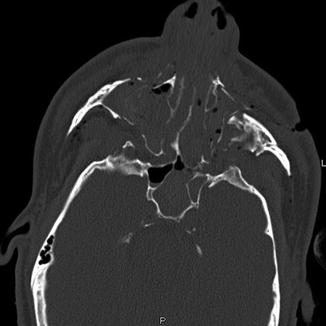
Fig. 3.15
Complex midface fractures with Lefort II and III components after being kicked in the face by a deer
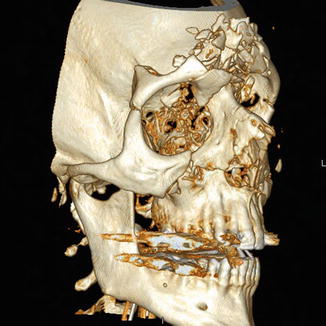
Fig. 3.16
3D reconstructions of the facial fractures in the same patient as Fig. 3.12 further showing the comminuted nature and midface depression
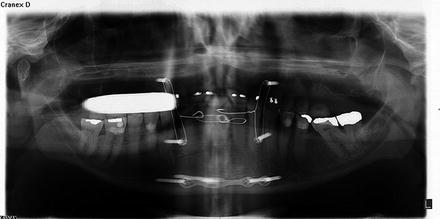
Fig. 3.17
Panorex image illustrating the detail in dental hardware and alignment in a patient with bilateral mandibular fractures
3.5.1 Injury Scaling
Injuries included in the Face category of the AIS, including the eye and ear, are heavily biased towards bony fractures. The only nerve included in this area is the intraorbital portion of the optic nerve. Injuries to the globe itself, though often morbid due to impairment in vision, still receive fairly low AIS scores of 1 or 2. Fractures to the Mandible, Maxilla, and Orbit also carry reasonably low scores despite the fact they can require multiple operations and carry long term consequences in altering the cosmetic appearance of the face and in affect the psychologic recovery of the patient. Complex fractures of the maxilla that involve deeper bony structures including the pterygoid or sphenoid bones can lead to an overall higher ISS score as they are coded in both the facial and head categories (assuming no other intracranial injuries are present).
3.6 Chest
Imaging of the chest occurs earlier in the evaluation of trauma patients than any other body region as a chest X-ray is considered such an important tool it is an adjunct to both Primary and Secondary Surveys. Current recommendations from ATLS favor liberal use of chest X-rays as they can detect several potentially life threatening injuries such as a large hemothorax, simple pneumothorax (tension pneumothorax being a clinical diagnosis), tracheobronchial tree injuries, traumatic aortic disruptions (widening of the mediastinum), blunt esophageal injuries, and diaphragmatic injuries [20]. Detection of many of these processes are important early in the evaluation of a trauma patient as they can trigger immediate intervention such as tube thoracostomy (i.e. chest tube) placement prior to continuing the Secondary Survey as well as help guide the decision making process as to what additional imaging may be needed (Figs. 3.18, 3.19, and 3.20).
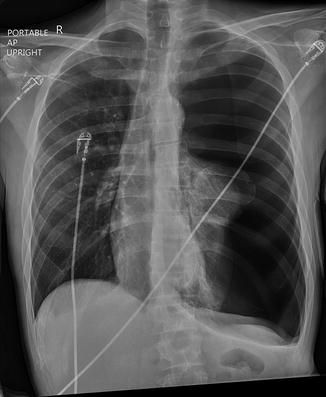
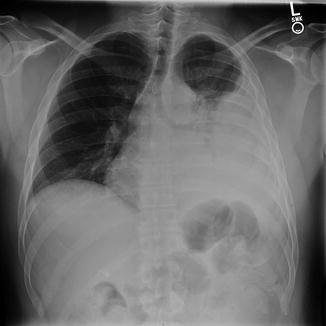
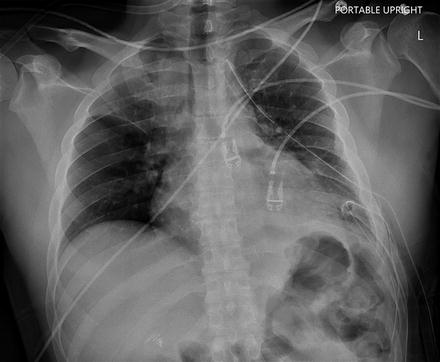
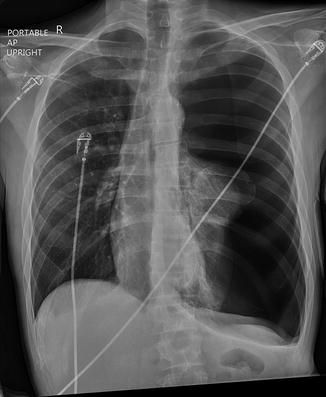
Fig. 3.18
Large left pneumothorax with mediastinal shift
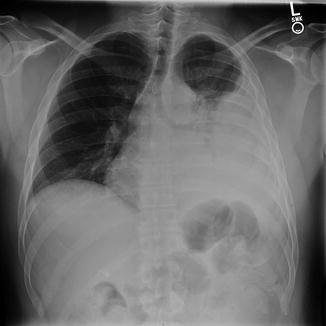
Fig. 3.19
Large left hemothorax identified 3 days after motor vehicle crash. A left seventh rib fracture is also identified
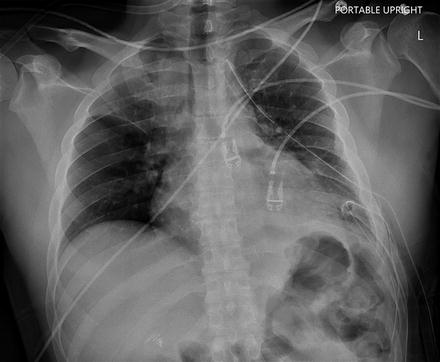
Fig. 3.20
Post operative chest X-ray of the patient in Fig. 3.20 showing marked improvement of the hemothorax
The other imaging modality used early in the resuscitation process as an adjunct to the Primary Survey is ultrasound. A FAST exam can be used to identify life threatening injuries very early in the resuscitation. The use of abdominal FAST will be discussed in greater detail later. The ultrasound imaging view obtained during a FAST exam to evaluate for thoracic trauma is a pericardial view with the goal primarily being to identify fluid, presumably blood in the setting of trauma, within the pericardial sac which could be causing cardiac tamponade. Beyond evaluation for tamponade, FAST in the thoracic cavity can also detect hemothorax and pneumothorax. Hypotension can come from a multitude of places in the trauma patient; however, early recognition of tamponade physiology is critical. Physical examination identifying the classic Beck’s triad of muffled heart tones, hypotension and jugular venous distension can be difficult in the initial assessment of a critically ill patient. Ultrasound provides the Emergency Medicine physician or Trauma Surgeon the opportunity for a rapid assessment of the pericardial space, with an accuracy of up to 90 % when performed by an experienced provider.
3.6.1 Heart
In the vast majority of patients with blunt cardiac injury, the injury does not pose significant danger, and those with the most significant injuries often do not survive long enough for them to be recognized. Screening for blunt cardiac injury begins with obtaining an Electrocardiogram (ECG) on arrival to the hospital in patients with a suspected injury and abnormality noted on telemetry [21]. Significant chest wall trauma should be an early warning sign, however studies have shown sternal fractures alone are infrequently associated with blunt cardiac injury [22]. The most common ECG findings include unexplained sinus tachycardia, frequent premature ventricular contractions, arrhythmias, heart block, or signs of ischemia. Though FAST exam in the hands of an experienced operator can help identify some of the more significant valvular disruptions and pericardial bleeding, ECG remains the screening modality of choice, with a negative predictive value (NPV) of >95 %. Cardiac enzymes including Troponin I have been evaluated as an additional measure, but have been found to be more indicative of cardiac ischemia than an actual cardiac injury [23]. If tested and elevated, concomitant myocardial infarction should be appropriately excluded, as elderly trauma patient can be at increased risk of MI as an inciting event or as a result of trauma. In patients with ECG abnormalities concerning for a blunt cardiac injury or otherwise unexplainable hemodynamic compromise, formal 2D Echocardiogram transthoracic or trans esophageal should be performed as a diagnostic test [24]. Screening with echocardiogram is not recommended due to the cost and low yield in low risk patients. Lastly, the role of CT and MRI in evaluation has long been limited by their speed and poor ability to detect abnormalities in a beating heart. As the technology improves especially with MDCT and the advent of ECG gated image acquisition, their role may expand. Presently, the utility of ECG gated imaging is limited to case reports and series and the feature is not widely available or part of most trauma imaging protocols.
3.6.2 Chest Wall
Chest wall injuries, fractures of the ribs are among the most common injuries following blunt trauma, occurring in up to 70 % of injured patients, with injury to the underlying lung occurring frequently, even without bony fracture [25]. In selected young otherwise healthy patients with minimal associated injuries, non-displaced rib fractures with minimal symptoms can be insignificant enough to allow discharge directly from the emergency department. However, often times rib fractures carry a significant morbidity and mortality, especially in older patients, and therefore identifying them to develop an appropriate plan of care and rule out injuries to the lung is important. Fractures to >3 ribs has been suggested as potential criterion for transfer to a trauma center, and rib fractures in patients over the age of 65 are associated with a significantly increased morbidity and mortality. Chest wall imaging begins with the initial Chest X-ray during the Primary or Secondary Survey. Significant hemothoraces or pneumothoraces may require intervention at that time. A normal supine chest X-ray in an otherwise hemodynamically normal patient without a concerning mechanism of injury can be enough information to exclude the need for further CT imaging.
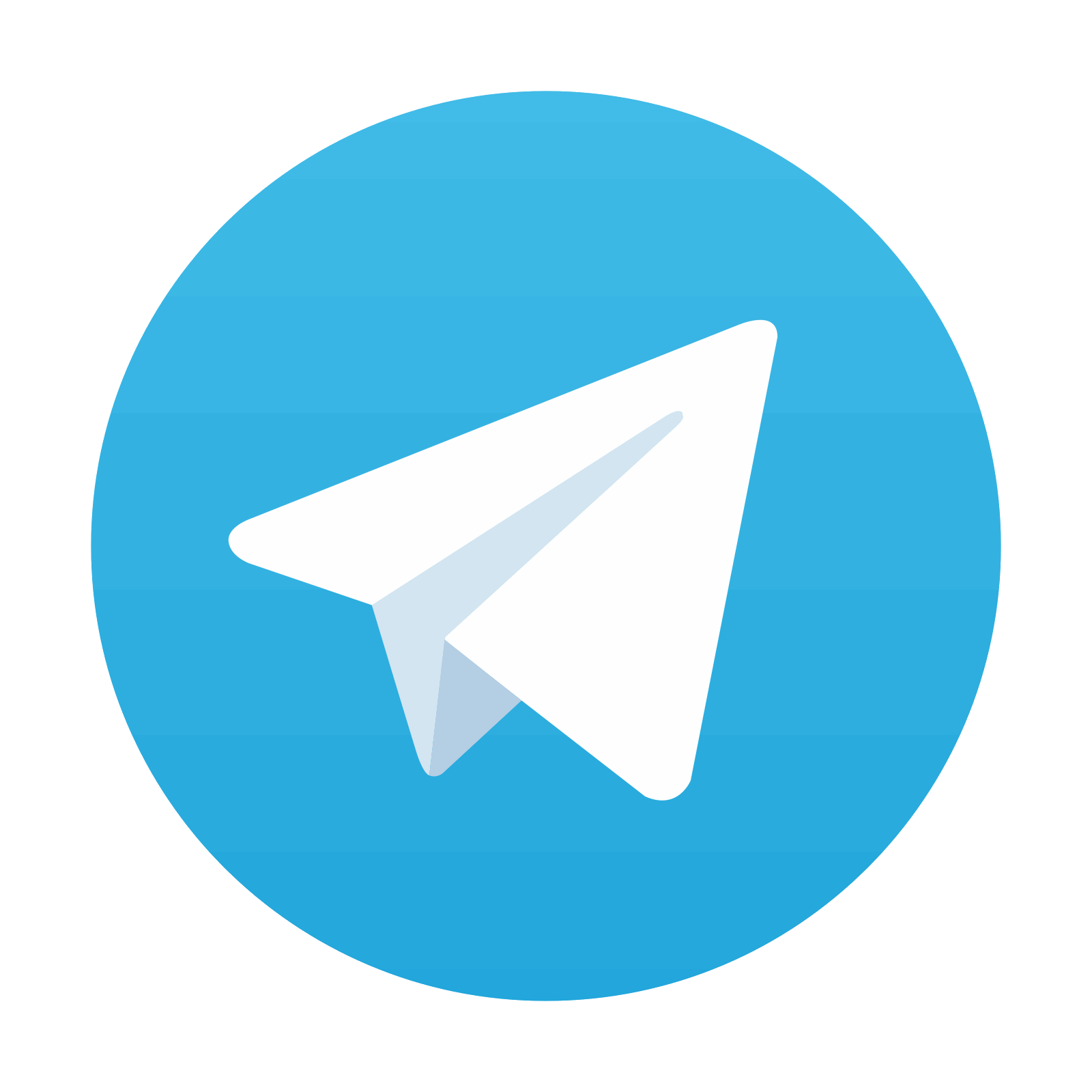
Stay updated, free articles. Join our Telegram channel

Full access? Get Clinical Tree
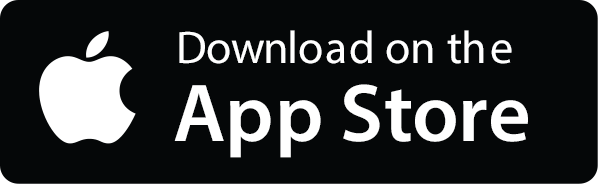
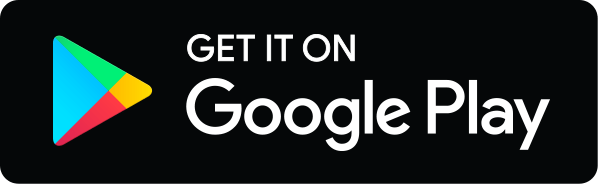