Chapter 3 Mechanism of injury
Injuries to the human body from external dynamic exposure occur due to the application of abnormal loads or motions that exceed physiological limits. Specific to motor vehicle rear-impact crashes, abnormal motions of the head–neck complex during the application of posterior–anterior acceleration to the seated occupant have been used to understand the biomechanical mechanism of injury, develop metrics to quantify trauma and, from a clinical point of view, determine structures that sustain the injury to explore improved treatment options. The focus of this chapter is to briefly discuss the kinematics, injury theories, injury metrics, and vehicle- and occupant-related factors involved in rear impact–induced injury.
Injury theories
Hyperextension theory: anterior column injury
Global hyperextension of the head–neck forms the basis of the hyperextension theory (Fig 3.1). Development of this theory was based on experiments using human volunteers, post-mortem human subjects (PMHSs), anthropomorphic test devices and primates.1–4 Clinical studies have reported that hyperextension most commonly results in anterior cervical column injuries in the form of anterior longitudinal ligament and intervertebral disc ruptures.5–11 These injuries were reproduced during rear-impact experiments. For example, hyperextension-type injuries occurred in anaesthetised primates in simulated automotive rear-impact collisions.1 The primates were placed supine on a steel platform without head restraint, and the platform was accelerated by a guided drop tower. The initial platform height varied from 0.6 m to 12 m. Another study documented cervical spinal injuries consistent with the hyperextension mechanism by subjecting PMHSs to single-application rear impacts at a change in velocity of 15 or 25 km/h.12 Sled tests were conducted with PMHSs seated upright in a rigid seat without head restraint. Two out of the three PMHSs subjected to high-velocity tests sustained anterior longitudinal ligament and disc ruptures. Likewise, a study subjecting PMHSs to multiple rear impacts using a relatively rigid seat demonstrated similar injury patterns in specimens following tests without head restraints.13 Anterior disc separation and tears of the annulus and anterior ligaments were identified in two out of the three PMHSs exposed to impacts between 11.0 and 15.5 km/h.
Injuries in the studies cited above occurred without the presence of a head restraint. However, these safety devices were introduced into passenger vehicles in the United States in 1969 with the intent of preventing head–neck hyperextension during rear-impact collisions. Therefore, based on global head–neck kinematics and using the hyperextension mechanism, it is difficult to explain the most commonly reported symptoms of posterior neck pain and headache reported in a majority of contemporary cases of rear-impact collisions. In the absence of global head–neck hyperextension, localised or segmental hyperextension may lead to injuries that result in neck pain. In particular, extension in lower cervical segments (C4–C7) may exceed local tissue thresholds and result in tension injury to anterior soft tissues. Some clinical studies using data derived from vehicles equipped with head restraints seem to support this assertion by continuing to report the presence of anterior cervical column injuries, including anterior longitudinal ligament ruptures, horizontal avulsion of vertebral end plates, and separation of the intervertebral disc from vertebral bodies.5, 14–16 Experimental and computational literature has provided some additional support. Tests using PMHSs subjected to multiple impacts in a rigid seat with a head restraint have reported similar hyperextension-type injuries.13 A computational modelling study showed that segmental anterior longitudinal ligament strains can approach catastrophic failure levels during the retraction phase, before head-to-head-restraint contact.17 This finding is particularly relevant as it demonstrated that global head–neck hyperextension is not required to induce local hyperextension-type injuries.
Hydrodynamic theory: nerve root injury
Increased hydrodynamic pressure in the spinal canal during cervical spine bending forms the basis of the hydrodynamic theory.18, 19 This theory centres around the fact that length and cross-sectional area of the spinal canal increase during flexion and decrease during extension.20 During extension motion, as experienced during automotive rear-impact collisions, decreased cross-sectional area can be attributed to protrusion of the ligamentum flava into the canal space due to laxity from the reduced distance between adjacent laminae. In combination, decreased length and area lead to an overall decrease in spinal canal volume, resulting in increased hydrodynamic pressure. Because of the incompressibility of spinal fluids, the decreased canal volume results in a proportional outward displacement of its contents. The canal outlets are the foramen magnum superiorly, cervicothoracic junction inferiorly, and intervertebral foramina laterally between adjacent vertebrae. In the case of blood supply in the anterior internal venous plexus, the flow can occur through the foramen to the anterior external venous plexus. In the case of cerebrospinal fluid, the flow can occur out of the spinal canal and into nerve root sleeves (Fig 3.2). Increased blood and cerebrospinal fluid flows lead to a decreased cross-sectional area of the foramina (i.e. stenosis), which can apply a compressive load to the nerve root. Depending on the volume and flow rate, the compressive load may exceed tolerance, resulting in transient or chronic dysfunction of the affected root(s).
Nerve root pain from compressive radiculopathy is commonly reported by occupants involved in automotive rear-impact collisions. In particular, sharp pain and paraesthesia in the neck, shoulders and upper back are associated with compression of the nerve roots.21 Clinical rear impact studies have reported consistent findings. For example, neck, shoulder and back pain were reported by 92%, 49% and 39% of referred patients, respectively, following whiplash injury.22 However, pain directly attributed to the nerve roots (radicular pain) was present in only 15% of patients. Another study reported that neck pain was present in 100% of consecutive patients involved in an automotive rear-impact collision.23 Forty-eight per cent of those patients reported concomitant paraesthesia symptoms. Other clinical and epidemiological studies have reported similar findings, although this list is not comprehensive.24–27 While cervical nerve roots may be injured during automotive rear-impact collisions, nerve root injury can only explain some of the symptoms involved in whiplash associated disorders (WAD).
Eccentric contraction theory: muscle injury
Eccentric contraction of neck muscles forms the basis of the eccentric contraction theory.28–30 Under physiological circumstances, muscle contraction results in shortening of the muscle’s overall length (i.e. concentric contraction). However, during eccentric contraction, muscle length increases due to an external force of greater magnitude than the contraction force. Lengthening contractions result in greater injury potential and severity to the muscle than concentric contractions.31–33 During automotive rear-impact collisions, the anterior neck muscles are subjected to eccentric contraction during the retraction and extension phases as the relative length between muscle origin and insertion increases due to the head moving posteriorly relative to the lower cervical spine and thorax. Posterior neck muscles are subjected to eccentric contraction during the rebound phase as the head moves anteriorly. This theory was advanced in a study in which 42 human volunteers were subjected to rear-impact events at changes in velocity of 4 and 8 km/h.28 Electromyographic (EMG) activity of the superficial neck muscles was correlated to the timing of specific rear-impact events. Based on the observation that neck muscles initiate contraction rapidly during the impact event, it was hypothesised that injury may occur to the sternocleidomastoid muscles during the retraction phase (Fig 3.3). This hypothesis is consistent with earlier findings in which injuries to the sternocleidomastoid and longus colli muscles were identified in primates, as discussed earlier.1 As muscle injuries generally heal quickly, they may not result in chronic symptoms, reported by a considerable number of patients with WAD.
Localised posterior joint motions: facet joint injury
Stretching of the facet joint capsule forms the basis of the facet joint injury theory (Fig 3.4). This theory is based on the recognition that lower cervical facet joints may be responsible for posterior neck pain, a common outcome of rear impact–induced trauma.16, 22–24, 27, 34–39 Level-specific nerve blocks were used to determine the manifestation of facet-joint mediated pain.40, 41 Other studies identified the presence of mechanoreceptors/nociceptors,42–44 substance P,45, 46 and calcitonin gene-related peptide (CGRP)46 in facet joints. Mechanoreceptors may generate a noxious response during joint capsule stretch.47 Substance P is responsible for the initiation of pain and CGRP is associated with the persistence of pain.48 These anatomically and physiologically based studies have clearly identified the link between facet joint injury and neck pain. Concurrently, biomechanically based investigations were focused on defining the biomechanical role of facet joints in the injury mechanism.
Using whole-body, intact head–neck and isolated PMHS cervical spine specimens, increased and abnormal facet joint motions were reported during the retraction phase.13, 49–51 Lower cervical facet joints demonstrated region-dependent kinematics: uniform shear motion along the joint, and distraction in ventral and compression in dorsal regions of the joint.51 This pattern was significantly different from facet joint motions under physiological loads.52 Excessive joint stretch can result in capsular ligament failure. The stretch theory is supported by whole-body PMHS tests that identified lower cervical joint capsule injuries following rear-impact loading.12, 13, 53 Facet joint diastasis and capsular tear were observed in sagittal anatomical sections of the spine.53 Using a rodent model, cervical facet joint stretch was found to be associated with allodynia.54 Although pain symptoms were manifested in the forepaw, this study was the first to associate experimentally induced facet joint injury through joint capsule stretch with noxious response. The anatomical, physiological and biomechanical studies outlined in this section have identified a key role of lower cervical facet joints in the whiplash injury mechanism.
Injury metrics
Neck injury criterion
The neck injury criterion (NIC) is based on the spinal canal hydrodynamic theory described above.55, 56 A mathematical model was used to predict the change in pressure within the canal at the C4 level due to changes in canal volume associated with retraction of the head relative to the thorax. Greatest pressure gradients were associated with the S-shaped curvature of the cervical spine. The mathematical equation used to predict these pressure gradients was:
where L is the length of the neck, and arel and vrel are the relative horizontal acceleration and velocity changes between the C1 and T1 vertebrae during the retraction phase.
Because the head remains stationary during the retraction phase, arel and vrel can be approximated as the acceleration and velocity of the torso, and arel and vrel are derived from relative acceleration–time traces for the head and upper thorax in a dummy. Studies cited above proposed the tolerance limit at 15 m2/s2 for a neck length of 0.2 m. The NIC metric is valid when data are analysed from the time of rear impact initiation until head restraint contact.
Neck displacement criterion
The neck displacement criterion (NDC) assesses injury risk using overall head–neck rotation, anterior–posterior displacement, and vertical displacement of the occipital condyles relative to the T1 vertebra. It was developed to be used with human volunteers and dummies, including the BioRID P3 and Hybrid III,57 and was based on data derived from human volunteer rear impact tests.58, 59 Tolerance limits were based on linearised versions of ±2 standard deviation responses of human volunteers subjected to 9.3 km/h rear impacts. The reader is referred to the 2002 paper for the specific tolerance curves.57
Interaction criterion
The interaction criterion in its most basic form (Nij) is based on the interaction of forces and moments on the cervical spine.60 For rear impact, the criterion uses tensile force and extension moment, Nte. The tension intercept of 3300 N was derived from a study correlating injuries sustained in real world frontal collisions with the Hybrid III dummy responses under similar loading conditions.61 The extension moment intercept of 57 Nm was approximated using PMHS sled test results.62 The interaction equation is:
where Fz refers to the peak tensile force, My refers to the peak extension moment, and Fint and Mint refer to the respective intercepts. Values for force and moment intercepts have also been presented for different-sized occupants, such as the 95th percentile male and 5th percentile female.
Interaction criterion
The shear-moment interaction criterion was developed based on the argument that anterior–posterior shear forces rather than axial forces are important in cervical spine trauma induced during rear-impact collisions.63 It also has the rationale that injuries induced in the rebound phase cannot be analysed using the NIC as it is only applicable to the retraction phase. Instead of the axial tensile force in the Nte formulation, shear force is used in the interaction equation for Nkm:
where Fx refers to anterior–posterior shear force and My refers to upper neck sagittal bending moment.
Intercept limits to represent human tolerance for the Abbreviated Injury Scale (AIS) level 1 or minor injuries were: extension moment, 47.5 Nm; flexion moment, 88.1 Nm; and shear force, 845 N.64, 65 Forty sled tests using the Hybrid III dummy with TRID neck and BioRID and production motor vehicle seats were used to validate the metric. The metric showed sensitivity to seat type, with seats equipped with a ‘whiplash-protection system’ resulting in lower values associated with lower injury risk.
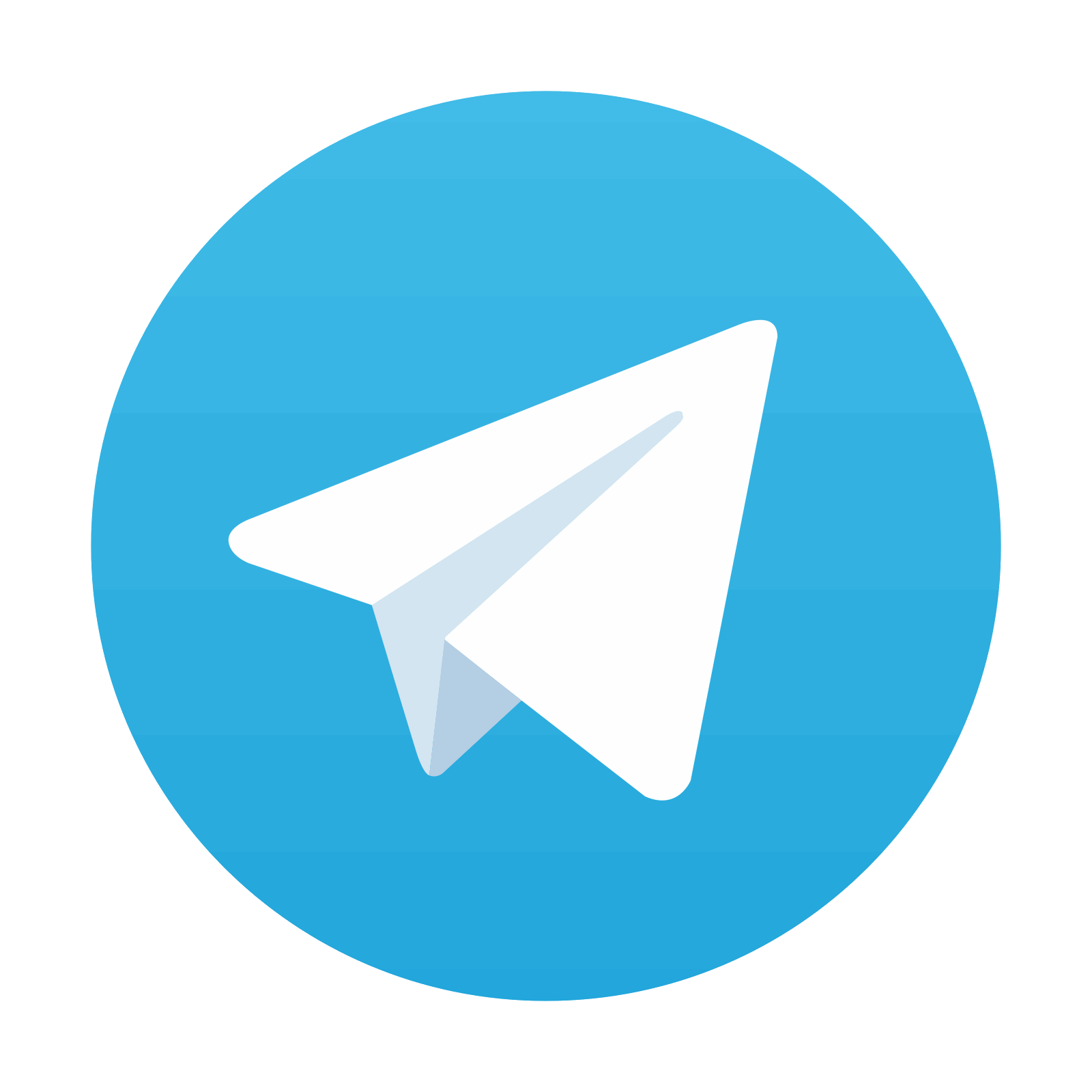
Stay updated, free articles. Join our Telegram channel

Full access? Get Clinical Tree
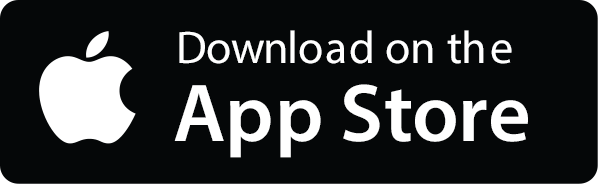
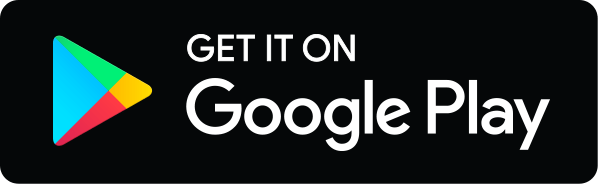