Study, year [ref]
N
Sex
Duration
Design
Intervention
HbA1c, %
Metabolic bone markers
PTH
25(OH)D
IGF-1
Others
M/F
Baseline
End
CTX
NTX
DPD
BAP
P1NP
OC
Gregorio, 1994 [27]
50
24/26
12 months
OBS
Various
11.6
NR
↓
↓
Hyp↓ uCa↓
Nagasaka, 1995 [26]
28
13/15
38 days
OBS
Various
11.1
9.3
↓ALP
↑
↓
uCa↓1,25D→
Sayinalp, 1995 [28]
16
NR
NR
OBS
Various
9.8
7.8
↑
Hyp →
Okazaki, 1997 [29]
78
27/49
3 weeks
OBS
Various
9.9
8.7
↓
↓
↓
↑
→
uCa↓1,25D→
Rosato, 1998 [30]
20
12/8
2 months
OBS
Various
10.0
8.1
↑
↑
→
→
↑
PYD↑
Okazaki, 1999 [95]
33
16/17
1 month
OBS
Tro
8.4
8.4
↓
↓
↓
→
Watanabe, 2003 [96]
25
11/14
1 month
OBS
Tro
8.4
7.6
↓
↓
12 months
7.5
→
→
Capoglu, 2008 [31]
35
17/18
12 months
OBS
Met ± SU
10.6
7.7
↓
↓
↓
→
→
Kanazawa, 2009 [97]
50
31/19
1 month
OBS
Various
10.0
8.8
→
↓
↑
Kanazawa, 2011 [32]
50
28/22
6 months
OBS
Various
7.6
6.9
→
↑
→
Zinman, 2010 [38]
250
F
12 months
RCT
Rosi
7.4
~6.8
↑
↓
↓
↓
↓
E2 ↓
299
M
Rosi
→
↓
↓
↓
↑
E2 →
225
F
Met
7.3
→
↓
↓
↓
→
E2 →
326
M
Met
↓
↓
↓
↓
↓
E2 →
214
F
Glyburide
7.4
↓
↓
↓
→
↑
E2 →
291
M
Glyburide
↓
↓
→
→
↓
E2 →
Borges, 2011 [36]
87
43/44
80 weeks
RCT
Met + Rosi
8.6
6.8
↓
↓
↓
→
→
87
38/49
Met
8.6
6.6
↓
↓
↓
→
→
Bilezikian, 2013 [37]
111
F
52 weeks
RCT
Rosi
6.8
6.4
↑
↓
↑
↓
↓
→
110
F
Met
6.8
6.3
↓
↓
↓
↓
↓
→
van Lierop, 2012 [38]
37
M
24 weeks
RCT
Met
7.0
6.3
↓
↓
Scl →
34
M
Pio
7.1
6.5
↑
→
Scl ↑
In 1997, we did the first such study using specific bone markers including urinary DPD, CTX, BALP, as well as OC. Prior to our study, there had been a few longitudinal studies, but they either had not assessed bone resorption markers [26] or used urinary hydroxyproline, a nonspecific collagen degradation product as a bone resorption marker [27, 28]. All three studies [26–28] used OC as a sole bone formation marker and reported conflicting results.
Gregorio et al. [27] reported that in 50 poorly controlled T2DM (basal HbA1c 11.6 %), insulin or oral agents available at that period resulted in a decrease in fasting blood glucose level from around 250 to 130 mg/dl in a month and the improved glycemic control was maintained up to 12 months (HbA1c levels were not reported except at the baseline). This was associated with time-dependent decreases in both OC and HYP. They also reported time-dependent decreases in PTH and urinary Ca along with amelioration of hyperglycosuria. They suggested that osmotic hypercalciuria triggers PTH secretion, which results in high bone turnover state in poorly controlled T2DM, and that glycemic control decreases bone turnover dependent on PTH. Nagasaka et al. [26] also found that glycemic control decreased urinary Ca and PTH, but they reported serum OC was increased rather than decreased. Interestingly they reported total ALP was decreased after glycemic control. They did not assess bone resorption markers. Increase in OC after glycemic control was also reported by Sayinalp et al. [28].
Our study [29] was the first to demonstrate that bone resorption markers, urinary DPD, and urinary CTX were decreased with glycemic control. We found that BALP and ALP were decreased but that OC was increased. Similar to previous studies, glycemic control was associated with a decrease in uCa excretion, but contrary to previous studies, we did not see any change in PTH. We concluded that poor glycemic control in T2DM is associated with increased bone resorption independent of PTH, which would be normalized after short-term glycemic control.
In contrast to our study, Rosato et al. [30] reported that in 20 T2DM patients, glycemic control over a 2-month period (mean HbA1c level, from 10.0 % to 8.1 %) with insulin or various oral hypoglycemic agents (OHA) was associated with significant increase not only in OC but also in uDPD and uPYD. Although details of OHA they used were not described, the use of thiazolidinediones (TZDs) seems unlikely as they were not clinically available at the time. Then this is the only study demonstrating increase in resorption markers after glycemic control with non-TZDs. It is of note that compared with nondiabetic controls, T2DM patients’ baseline OC was lower, whereas urinary DPD and PYD were not different. In this study, serum 25(OH)D and PTH did not change significantly, but there was a significant increase in serum IGF-1 level.
The results reported by Capoglu et al. [31] were similar to ours except osteocalcin. They reported that in 35 T2DM patients’ urinary DPD and NTX, osteocalcin and BALP were all decreased in the course of 1 year, associated with an improvement in glycemic control from HbA1c level 10.6–7.7 % with metformin and/or sulfonylureas [31]. PTH and 25(OH)D did not change. Kanazawa et al. [32] reported a small but significant increase in BAP after improvement of glycemic control over 6 months, but it is of note that in this study change in HbA1c was positively associated with the change in BAP. On the other hand, OC and NTX did not significantly change, but their changes were negatively associated with the change in HbA1c.
After it became evident that TZDs increase fracture risk, several randomized control studies (RCTs) examined metabolic bone markers in response to TZDs compared with metformin or SUs. The largest RCT is the post hoc analysis of A Diabetes Outcome Progression Trial (ADOPT), in which the effects of rosiglitazone, metformin, and glyburide monotherapy were compared [33–35]. Zinman et al. [33] measured CTX, BALP, and P1NP at the baseline and after 1 year in more than 500 subjects in each monotherapy group. As shown in Table 4.1, all the bone markers were decreased or stable except in female rosiglitazone group showing an increase in CTX. PTH was decreased in rosiglitazone and metformin group but was stable with glyburide. In this post hoc analysis, HbA1c levels were not presented, but in the parental study, basal HbA1c levels were around 7.4 %, which were decreased around 6.8 % after a year [34]. Borges et al. [36] did a RCT comparing effects of rosiglitazone plus metformin versus metformin alone for 80 weeks. Both treatments were associated with decreases in CTX, P1NP, and BALP with improvement in glycemic control with HbA1c level from 8.6 % to around 6.8 %. Another RCT comparing effects of rosiglitazone against metformin reported that similar small improvement in glycemic control (HbA1c 6.8–6.3 %) was associated with decreases in CTX, P1NP, and BALP in metformin but that in rosiglitazone group increases in CTX and P1NP and a decrease in BALP [37]. Finally van Lierop et al. compared the effects of pioglitazone against metformin [38] and reported that 6 months treatment with metformin decreased both CTX and P1NP, whereas pioglitazone increased CTX and had no effect on P1NP.
To summarize all the results, bone resorption markers were decreased in the course of glycemic control with most treatment modalities except TZDs. Among bone formation markers, BALP was also decreased whereas OC was increased at least in some studies. BALP decrease after glycemic control was suggested to be caused by improvement of vitamin D status, but in two studies [33, 37] BALP was decreased despite the decrease in serum 25(OH)D level. P1NP may be also decreased with glycemic control. OC increase after glycemic control may be associated with direct insulin action on osteoblasts, as will be dealt in another chapter.
4.4 Hyperglycemia and Calcium-Regulating Hormones
Bone turnover is controlled by many systemic and local factors. Among the systemic factors, conventional calcium-regulating hormones, PTH and vitamin D, have been studied in relationship with glycemic control. There are little data on other hormones such as sex steroids in the context of bone abnormalities in diabetics.
4.4.1 PTH in Diabetes
It has been often noted that PTH is low in diabetes, especially when associated with overt renal insufficiency [29, 39–43]. However, a recent meta-analysis of PTH level in both type 1 and 2 diabetics revealed no differences compared with nondiabetics [25]. Uncontrolled diabetes with overt glycosuria causes hypercalciuria by osmotic diuresis. Such renal calcium loss would trigger PTH secretion in normal subjects. However, results from longitudinal studies are mixed even at the baseline. Some studies reported high PTH in uncontrolled diabetic state [27, 31], but others reported relatively low PTH despite overt hypercalciuria [26, 29]. PTH responses to the improvement in glycemic control are also variable: decrease, no change, and even increase have been reported (Table 4.1).
The causes of such variations in PTH secretions in diabetics are unknown. As will be discussed later, vitamin D deficiency is common in diabetics, which would stimulate PTH secretion. However, basal serum 25(OH)D levels in those two studies that reported high basal PTH were not very low [27, 31] and comparable to the studies reporting normal PTH at the baseline [33]. Changes in serum 25(OH)D with glycemic control are also mixed, which could not explain variable PTH responses to an improvement of glycemic control (Table 4.1). Serum levels of 1,25(OH)2D, the most active metabolite of vitamin D, do not significantly change with glycemic improvement [26, 29] (Table 4.1). Thus, vitamin D would affect PTH secretion, but there are likely to be other unknown factors that influence PTH secretion in diabetes. In bovine parathyroid cells, hyperglycemia itself inhibits PTH secretion [44] with unknown mechanisms.
4.4.2 Vitamin D Deficiency/Insufficiency in DM
Vitamin D deficiency/insufficiency is very common among diabetics. Indeed, meta-analyses of prospective cohort studies reported that low serum 25(OH)D level is associated with higher incidence of diabetes [45]. Whether this relationship is casual or not remains to be determined. It is of note that obesity with visceral fat deposition is associated with low serum 25(OH)D and high PTH [46]. In obese subjects with or without type 2 diabetes, weight loss by diet and/or exercise, but not by bariatric surgery, is associated with an increase in serum 25(OH)D concentration [47–49]. Furthermore, weight-associated change in serum 25(OH)D concentration is associated with reciprocal change in serum PTH level in nondiabetics [50]. Whether or not such change in PTH is observed in diabetics is not known.
Another factor that results in decreased serum 25(OH)D level in diabetes is diabetic nephropathy. In circulation, most vitamin D metabolites including 25(OH)D are bound to vitamin D-binding protein (DBP). In the kidney, DBP-25(OH)D complex is freely filtered across the glomerulus allowing transport to the proximal tubule. In the proximal tubule, DBP-25(OH)D complex is reabsorbed in a manner dependent on megalin, an endocytic receptor, expressed in polarized epithelial cells including proximal tubule cells [51]. It is a multiligand receptor, with an extensive repertoire of ligands including albumin, lipoproteins, hormones, enzymes, drugs, as well as DBP. Diabetic nephropathy with microalbuminuria or proteinuria causes megalin loss into the urine, which results in a decreased vitamin D reabsorption and aggravates poor vitamin D status [51, 52]. In human type 1 diabetics, it was reported that both albuminuria and urinary megalin levels are associated with low serum 25(OH)D [53]. Similar results have been obtained in both type 2 and type 1 diabetic model rats [52, 54].
< div class='tao-gold-member'>
Only gold members can continue reading. Log In or Register a > to continue
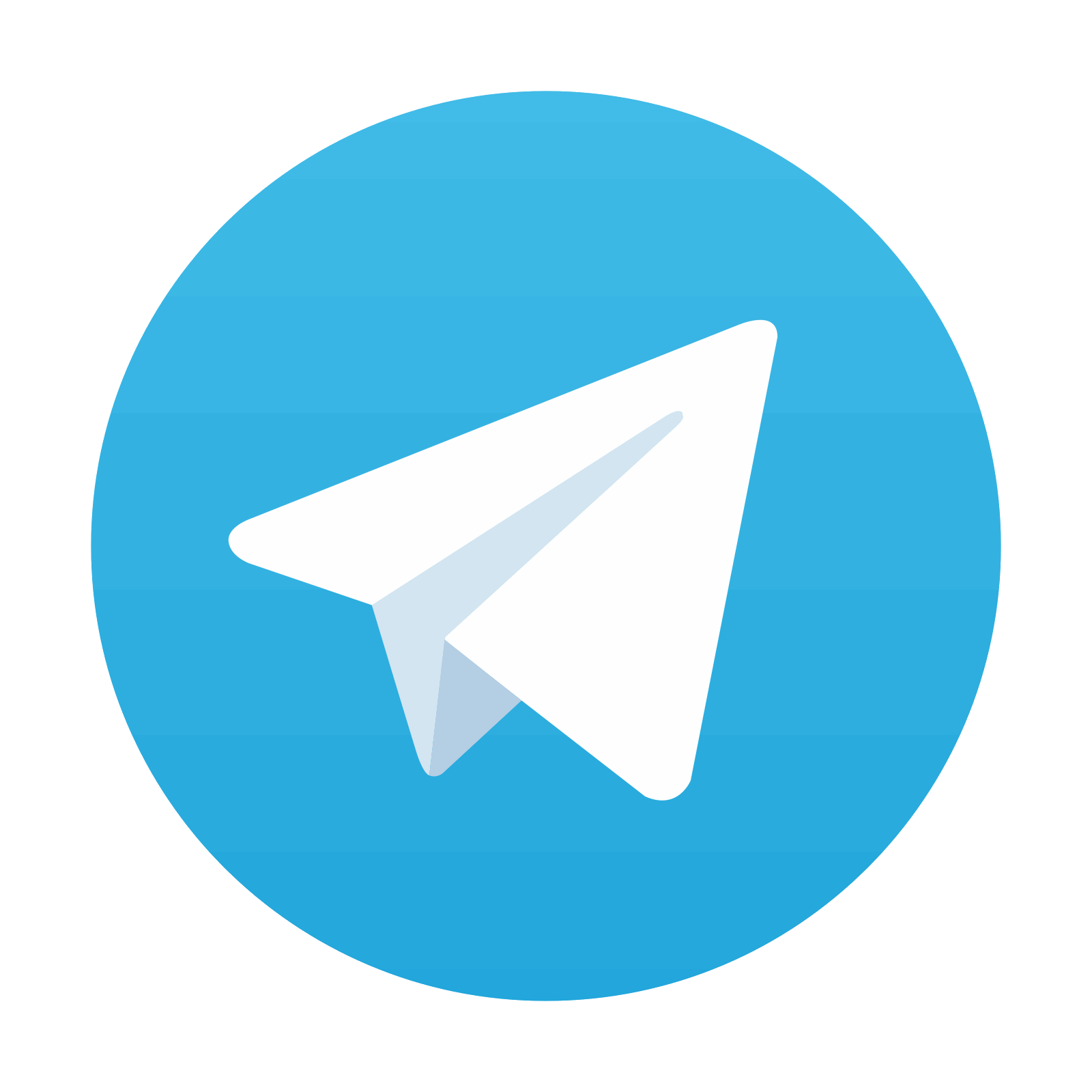
Stay updated, free articles. Join our Telegram channel

Full access? Get Clinical Tree
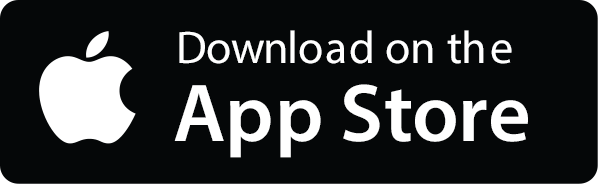
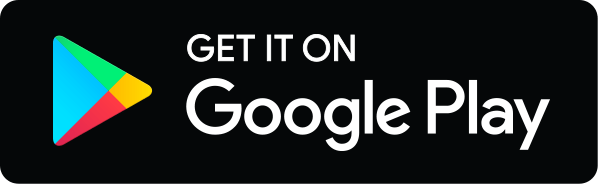