CHAPTER 1
Manipulation
Therapy Theory
Introduction
Manipulation therapy is a type of physical therapy that is practised worldwide by health care professionals in various specialities, such as osteopathy, chiropractic and physiotherapy, to treat musculoskeletal pain and disability (Rubinstein et al., 2011). The therapy uses drug-free, non-surgical techniques to reduce joint pressure, improve joint range of motion, restore muscle and tissue balance, promote body fluid mobilisation, decrease inflammation and enhance nerve function (Di Fabio, 1992; Cyriax, 1973). Scientific research on this modality continues; so far, a number of positive clinical findings have been reported. However, the theoretical base to support every aspect of its therapeutic use is still underdeveloped (Evans, 2010). Hence, the therapy has primarily been used for the management of a range of muscle and joint conditions.
Although the volume of research on joint manipulation has increased significantly in recent years (Bronfort et al., 2008), little is understood about how this therapy works and what physiological effects it causes on various parts of the body (Evans, 2002). To date, many theories have been proposed to interpret these physiological mechanisms, but a unified theory based on scientific evidence is still lacking. However, this chapter is not written to offer a new theory based on the previous literature. Its purpose is to review features suggested to be essential components of manipulation and discuss various theories on physiological mechanisms that have been proposed up to now.
Historically, manipulation is one of the oldest techniques, which has its origins in parallel developments throughout the world (Schiötz and Cyriax, 1975). For thousands of years, it has been widely practised in many cultures to treat a variety of conditions associated with the musculoskeletal and other systems. The techniques have been carried down from one generation to the next (Wiese and Callender, 2005). The earliest record of the practice of spinal manipulation is found in China, which dates back to 2700 bce (Waddell, 1996). In Europe, Hippocrates (460–385 bce) was the first physician to describe the manipulation techniques (Withington, 1948).
Despite having an early history of parallel developments in many parts of the world, manipulation therapy has gained and lost favour with the medical profession many times over the centuries (Pettman, 2007). During the renaissance of medicine in the 16th century, Hippocrates’ manipulation techniques reappeared in the writings of a number of famous scholars, including Guido Guidi, Johannes Scultetus and Ambrose Paré, as a treatment for musculoskeletal conditions (Anderson, 1983; Pettman, 2007). Nevertheless, by the 18th century, the general acceptance of these techniques was rejected by physicians and surgeons. They viewed manipulation therapy as a practice of folk healers, also known as bonesetters, and attributed its successes more to luck than skill (Lomax, 1975).
From the 19th century onwards, the therapy became an area of dispute among medical professionals. However, because of shortcomings in allopathic medicine and the origination of two leading alternative health care systems, osteopathy and chiropractic, by the end of the 19th century, views about manipulation therapy irrevocably changed (Anderson, 1981); in the early part of the 20th century, medical and osteopathic physicians initially paved the way for introducing manipulation techniques to the physical therapy profession. Since then physical therapists have contributed substantially to the field and solidified manipulation therapy within in its legally regulated scope of practice (Pettman, 2007).
There is no satisfactory definition of manipulation because of its colloquial function. The term is so vague that many authors have found it very challenging to distinguish ‘real’ manipulation from its physical therapy counterparts (e.g. Song et al., 2006; Colloca, Keller and Gunzburg, 2004; Harvey et al., 2003). Many researchers have tried to provide a valid definition in diverse sources of literature, but a final definition has not yet been agreed. Moreover, the definition varies across specialities (Maigne and Vautravers, 2003). For example, in osteopathy, manipulation is not regarded as a complete treatment; rather, it is considered a part of the manipulative treatment strategy for a given patient (Wieting and Cugalj, 2008).
In addition, manipulation therapy is different from mobilisation, because, theoretically, it does not allow the recipient to stop joint movement during the procedure, whereas mobilisation techniques involve application of non-thrust passive motion to the spine that can be prevented by the recipient (Corrigan and Maitland, 1983).
In comparing previous definitions and descriptions of manipulation, Evans and Lucas (2010) presented several empirically derived features that are necessary to define ‘manipulation’ (see box below). The authors divided these features into two categories: the ‘action’ (that which one person, the practitioner, performs upon another, the patient) and the ‘mechanical effect’ (that which occurs within the patient, as a result of the action).
Proposed Empirically Derived Essential Features of Manipulation
Action (that which the practitioner does to the recipient)
•A force is applied to the recipient.
•The line of action of this force is perpendicular (at an angle of nearly 90°) to the articular surface of the affected joint.
Mechanical response (that which occurs within the recipient)
•The applied force creates motion at a joint.
•This joint motion includes articular surface separation.
•Cavitation occurs within the affected joint.
Source: Evans and Lucas (2010)
Types of Manipulation
Although there are many disputes about the definition of manipulation, it generally involves a thrust being applied to the recipient through either a long or a short lever-arm (Di Fabio, 1999). Osteopaths developed the long-lever techniques, whereas chiropractors the short-lever techniques (Maigne and Vautravers, 2003).
In long-lever manipulation (or low-velocity high-amplitude manipulation), the thrust is delivered in a non-specific manner, not directly to the vertebra – for example, to the shoulder, pelvic region or scapular (Shekelle et al., 1992). During this type of manipulation, the practitioner passively moves many vertebral joints simultaneously within their range of motion (Di Fabio, 1999).
On the other hand, the short-lever manipulation (or high-velocity low-amplitude manipulation) involves a low-amplitude thrust being applied at a contact point on a process, such as spinous process, lamina or mammillary process, of a specific lumbar vertebra to affect the vertebral articulation (Bergmann, 2005). During this process, the therapist applies a fast rotational force at an angle of 90° to the overlying skin surface of the affected joint (Cao et al., 2013).
What is the Cavitation/Crack/Pop?
During high-velocity, low-amplitude (HVLA) manipulation, a strong ‘thrust’ or ‘impulse’ is applied perpendicularly to a diarthrodial synovial joint. This action causes separation of the joint surface beyond a certain magnitude, producing an audible cracking sound. The cracking sound often signifies a successful manipulation (Sandoz, 1969), although it has been highly debated whether the sound is an essential feature of the manipulation or not (Brodeur, 1995; Flynn, Childs and Fritz, 2006).
The most widely accepted explanation for the production of this ‘crack’ sound is an event called ‘cavitation’, which occurs within the affected joint’s synovial fluid (SF) (Evans and Breen, 2006). The term ‘cavitation’ refers to the formation and activity of gaseous bubbles (or cavities) within the SF of the joint, which are created via local decline in pressure (Evans and Lucas, 2010). Cavitation usually results due to certain types of motion between the articular surfaces and can occur during both high- and low-velocity joint manipulation (Evans and Breen, 2006).
What is Paraphysiological Space?
Paraphysiological space, also known as a ‘zone of end-play’ or the ‘barrier’, is the zone of elasticity between the physiologic barrier and the anatomic barrier (Vernon and Mrozek, 2005). Sandoz (1976) first proposed the presence of a ‘paraphysiological space’ within the anatomic limit while describing the nature of joint manipulation. The author published a figure (see Figure 1.1) explaining several phases of a joint’s total arc of motion during manipulation, and depicted a space beyond the passive range but under the anatomic limit. However, the validity of the Sandoz model has been highly debated in recent years (Symons, Leonard and Herzog, 2002; Ianuzzi and Khalsa, 2005) due to the introduction of a new term ‘neutral zone’ by spinal biomechanics experts to describe the zone within a joint’s motion (Panjabi et al., 1988). As a result, many authors have suggested a revision to the old model (Vernon and Mrozek, 2005).
FIGURE 1.1 SANDOZ MODEL
Although some attempts have fallen short of providing a comprehensive revision to the Sandoz model (Gibbons and Tehan, 2001; McCarthy, 2001), Evans and Breen (2006) proposed a new general model of manipulation (see Figure 1.2), considering the requirement of a pre-thrust position and incorporating the ‘neutral zone’ into the original model. However, future research is required to test this model.
FIGURE 1.2 SCHEMATIC REPRESENTATION OF THE PROPOSED MODEL BY EVANS AND BREEN (2006)
Mechanism of Action of Joint Manipulation
Manipulation therapy has some strong clinical evidence for both acute and chronic low back pain (Bronfort et al., 2004; Jüni et al., 2009). However, the mechanism of action behind these clinical effects is only partly understood. Researchers have so far proposed many theories for the possible physiological mechanisms of manipulation, but scientific evidence to support these theories is still limited. This section discusses some of the noteworthy previous and current theories that have been proposed.
FIGURE 1.3 SCHEMATIC DIAGRAM OF THE PROPOSED PHYSIOLOGICAL MECHANISMS OF SPINAL AND PERIPHERAL MANIPULATION
Joint Gapping
The theory of joint gapping has a significant importance in under-standing the mechanism of joint manipulation. It has been hypothesised that gapping of the facet joint in the spine encourages release of the entrapped meniscoid (Evans, 2002), a capsule process that fills in empty spaces and compensates the incongruence of articular surfaces (Kos, Hert and Sevcik, 2001). Meniscoids are structures that have been thought to play an important role in inducing joint pain, because it has been identified that fibro-adipose meniscoids are capable of creating a painful situation (Bogduk and Jull, 1985; Mercer and Bogduk, 1993). Evans (2002) suggests that a HVLA manipulation, involving the facet joint gapping, results in impaction and an increase in joint space. These changes encourage the meniscoid to go back to its normal anatomic position in the joint cavity; once the meniscoid returns to its position, the joint capsule distension is ceased. As a result, the joint pain is also reduced.
Joint gapping theory is based on the most widely held belief that HVLA manipulation has biomechanical effects. The earliest biomechanical studies (e.g. Roston and Wheeler Haines, 1947; Unsworth, Dowson and Wright, 1971; Sandoz, 1976) to investigate the phenomenon of ‘joint cracking’ in finger joints (metacarpophalangeal) showed that joint surface separation was associated with the production of an audible ‘crack’ sound. These studies demonstrated that the separation of joint surfaces resulted in cavitation, the process responsible for the cracking sound, and an immediate increase in radiolucent joint space. Sandoz (1976) reported that this was associated with a 5–10° increase in range of movement at the joint. The author also noted that for about 20 minutes the cracking sound could not be repeated.
Similar results were reported in later biomechanical studies (e.g. Meal and Scott, 1986; Watson, Kernohan and Möllan, 1989) of ‘joint cracking’ in metacarpophalangeal (MCP) joints. To investigate this phenomenon further, Conway et al. (1993) compared the sounds from the spinal facet joint cavitations with the sounds from the MCP joint distractions. After analysing the sound signals from both the joints, the authors reported similar sound waves and proposed that a similar process was occurring in both joints. This means that HVLA manipulation may also result in an increase in joint space at the facet joints. In a more recent study, Cramer et al. (2000) provided further evidence to support this hypothesis. Using MRI scanning, the authors demonstrated that HVLA thrust caused an immediate increase in joint surface separation. In this study, the average increase in gapping for the HVLA group was +1.2 mm, whereas the average change for the control group was only +0.3 mm.
Unbuckling of Motion Segments
This theory is derived from the oldest concept that spinal manipulation realigns misaligned joints (Hood, 1871). For centuries, there was a long-held belief that traditional practitioners could put the bone back in place by manipulating the joint. This was also the likely reason that manual practitioners earned their name as ‘bonesetters’ (Bigos, Bowyer and Braen, 1994). Evans (2002) stated that the production of the audible cracking sound and the immediate symptomatic relief following manipulation could be the reasons for the development of this concept.
However, it is now identified that the source behind the ‘crack’ sound is the phenomenon called cavitation (Evans and Lucas, 2010). Moreover, recent biomechanical studies on the vertebral motion following spinal manipulation have found that the manipulated vertebrae associated with cavitation only show transient relative movements (Gal et al., 1997; Herzog, 2000; Evans 2002). As a result, the old theory of realigning misaligned vertebrae has become an epiphenomenon and a new theory has emerged in its place.
As individual motion segments can buckle, it has been hypothesised that production of relatively large motions at the vertebrae may help attain a new position of stable equilibrium (Wilder, Pope and Frymoyer, 1988). Based on this theory, a variety of hypotheses have been developed over the past decades. Triano (2000) suggested that the mechanical force applied during a high-velocity, low-amplitude thrust (HVLAT) manipulation might deliver enough energy to restore a buckled segment to a lower energy level, thus lessening mechanical stress or strain on soft and hard paraspinal tissues (Pickar, 2002). One of the major reasons behind the developing theory is the long-held hypothesis that spinal manipulation can restore the affected joint mobility and joint play. However, further research into this mechanism is required to establish the theory.
The muscular reflexogenic effect is another important theory that has been thought to play a role in the mechanism of manipulation. The musculature in the human body has some reflex responses, by way of its reflex arcs, to protect itself from potentially harmful force (Evans, 2002). Therefore, when a damaging force is applied to joints, the musculature creates positive synergism between the active (muscular) and passive (capsuloligamentous) joint restraints (Solomonow et al., 1998). This reflexogenic effect is hypothesised to create a reflex mechanism of reducing pain and improving muscle hypertonicity and functional ability (Potter, McCarthy and Oldham, 2005).
Manipulation has long been thought to influence the activation of the musculature through reflex pathways (Wyke, 1979). To confirm this hypothesis, a number of studies have been done over the past decades (e.g. Herzog et al., 1993; Herzog, Scheele and Conway, 1999; Symons et al., 2000; Suter et al., 2005). These studies demonstrated that spinal manipulation caused (excitatory) reflex responses not only in muscles local to the manipulated joint but also in more distant muscles. In addition, in a comparable experiment, Colloca and Keller (2001) measured the electromyographic (EMG) reflex response to spinal manipulation in patients (n = 20) with low back pain. Not surprisingly, the authors reported elicitation of a reflex response in the musculature; however, they also found that those who had frequent to constant pain symptoms had the largest amplitude of reflex response in comparison with those who had infrequent to occasional symptoms.
These studies provide some strong evidence for the fact that spinal manipulation results in reflex muscular contractions through mechanoreceptors in the joint capsules and muscles. However, it is not yet clear whether these reflex responses are actually caused by the joint manipulation, and are not merely artefacts resulting from some other part of the therapy (Potter et al., 2005).
Modulation of Alpha Motor Neuron Activity
It has long been hypothesised that back pain causes muscle hypertonicity, and that spinal manipulation stimulates nociceptive afferents, which in turn relax or normalise hypertonic muscle through modulating alpha motor neuron activity (Evans, 2002). However, scientific evidence to support this theory is still limited, and there has been some debate on this hypothesis (Potter et al., 2005). The earliest studies done to evaluate this theory suggest that a high-velocity thrust manipulation may activate nociceptive afferents, but only when forces involved with the manipulation are transferred to the surrounding joint capsule and soft tissues (Gillette, 1986, 1987; Herzog et al., 1993). Ahern et al. (1988) supported this hypothesis to some extent. The authors reported that a majority of patients with low back pain in their study actually prevented the activation of inhibitory afferents, as they failed to achieve the range of flexion necessary for the relaxation.
Lederman (1997) argued that the activation of inhibitory afferents is highly questionable because sudden stretch produced by high-velocity thrust manipulation would stimulate the motor neuron rather than inhibiting it. In contrast, Dishman and Bulbulian (2000) provided evidence that both spinal manipulation and mobilisation could result in significant but temporary attenuation of alpha motor neuronal excitability. The authors commented that their findings substantiate the theory that spinal manipulation might activate transient inhibitory effects on the human motor system. However, more evidence is needed to establish the theory, as well as its clinical and therapeutic relevance.
Modulation of Gamma Motor Neuron Activity
Korr’s theory (1975) of the facilitated segment is a decades-old theory that has been used to interpret the mechanism of manipulation. The theory was developed based on outcomes of the early EMG studies (Denslow, 1944; Denslow and Clough, 1941; Denslow, Korr and Krems, 1947). These primitive experiments found that when a stimulus was applied to a painful section, it showed an enhanced EMG response. Recently, Lehman, Vernon and McGill (2001) also demonstrated the same result with a more methodically correct study design. The authors also reported that spinal manipulation seemed to decrease the EMG response to a painful stimulus.
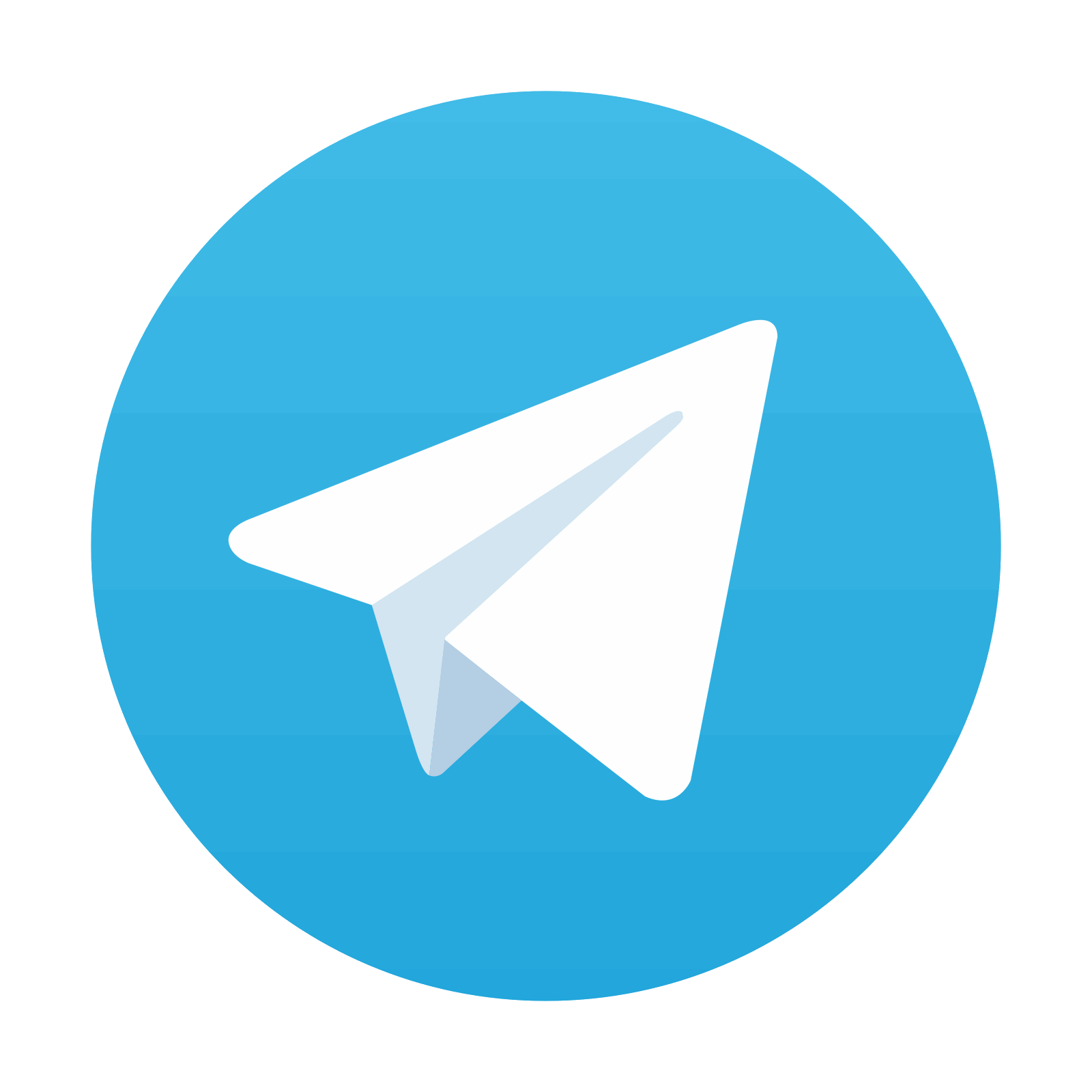
Stay updated, free articles. Join our Telegram channel

Full access? Get Clinical Tree
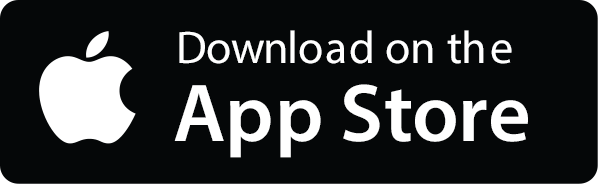
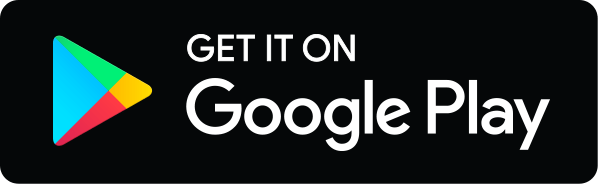