Lower Cervical Spine Injuries
Injury to the lower cervical spine can be devastating to the trauma patient, and it remains the responsibility of those involved in trauma care to ensure that all such injuries are properly detected and treated.
Because the anatomy of the lower cervical vertebrae (C3 to C7) is essentially identical, the injury patterns are similar across each segment and can be discussed together. The anatomy differs from the upper cervical spine (occiput to C2), where each segment is unique, creating distinctly different injuries. Much of the existing literature on subaxial spine injuries is of poor methodological quality. Therefore, practice standards cannot be established, only guidelines. This problem must be kept in mind in the course of reading any discussion on the topic.1
Injury Evaluation and Classification
Lower cervical spine injuries can be classified by bony morphology, anatomy, mechanism of injury, and the nature of the neurologic injury. Each of these factors has value, together they give an accurate representation of a particular injury and they guide the development of a treatment plan for that injury. A primary factor that guides treatment is the neurologic status of the patient. When present, a neurologic deficit is further characterized as complete or incomplete. This distinction affects treatment options as well as timing of treatment. For a neurologic deficit to be considered complete, there can be no motor or sensory function below the level of injury. Any discernible motor or sensory function below the level of injury makes it an incomplete lesion. The zone of neurologic injury can vary relative to the bony injury, so it is not uncommon to see function at the levels immediately caudal to the bony injury even when the injury is considered to be complete because there is loss of function at all lower levels. Additionally, there can be a “veil” of intact sensation about the shoulders because roots supplying this area exit from the upper cervical spine. A patient′s injury cannot reliably be considered complete during the period of spinal shock (the first 24 to 48 hours), but the likelihood of neurologic recovery is less than 3% if there is no evidence of distal function or sacral sparing within the first 24 hours.2,3 An incomplete neurologic deficit may be manifested as only sacral sparing, with intact perianal sensation or toe flexion, meaning that there is potential for neurologic recovery. Incomplete deficits are further subdivided into the following distinct patterns:
Central cord syndrome is the most common incomplete lesion and typically occurs after an impact to the spinal cord, compressing it peripherally toward the central core. The applied forces are greatest in the center of the cord, resulting in a distribution of injury that is explained by the anatomic organization of the spinal tracts within the cord. In this syndrome, the upper extremities are affected more than the lower extremities. Hand and upper extremity function is mediated by the corticospinal tract, which is located more medial and central within the spinal cord than are the lower extremity fibers. This spacial organization of the corticospinal tract has never been proven anatomically. Central cord syndrome is better explained by the corticospinal tract having a more dominant role in the upper extremities than the lower extremities in humans. Regardless, the prognosis to regain ambulatory function is relatively good when this injury occurs.
Anterior cord syndrome involves the anteriorly based tracts of the spinal cord while sparing the posterior columns, which preserves vibration and deep pressure sensation as well as proprioception. Motor functions, along with pain and temperature sensation, are the most severely affected. The prognosis for recovery is poor because this syndrome often has a vascular basis.
Posterior cord syndrome is essentially the opposite of anterior cord syndrome but is rarely seen as a result of trauma. Only the posterior columns are involved, perhaps secondary to impact from a lamina fracture.
Brown-Séquard syndrome is of interest due to the anatomic explanation for the resultant neurologic pattern of injury. Distal motor function is impaired ipsilateral to the injury, whereas contralateral pain and temperature sensation is impaired below the level of the injury. This effect is due to the fact that the decussation of the nerve fibers for the motor tracts occurs proximally within the brainstem, and the pain/temperature fibers (spinothalamic tract) cross within the cord, classically two levels below the level of injury. This injury is most often the result of penetrating trauma but can also be seen after a blunt impact applied laterally to the cord. Lastly, root injury differs from the above incomplete syndromes in that it involves lower motor neuron function with improved potential for recovery.
The neurologically based classification is further applied it to the functional status of the patient. The American Spinal Injury Association (ASIA) Impairment Scale follows the more traditional Frankel scale,4 but differs in several important respects and currently is the most commonly used functional scale. ASIA category A is defined as a person with no motor or sensory function as well as absence of function in sacral segments S4 and S5. ASIA category B is sensory function only with the requirement of preserved sacral S4-S5 function, making this an incomplete lesion. A patient would be an ASIA category C if more than half of the muscles evaluated had a grade of less than 3/5. If a person was graded as having more than half the muscles evaluated greater than 3/5, they are assigned to ASIA category D. ASIA category E implies that one can have spinal cord injury without having any neurologic deficits at least detectable on a neurologic examination of this type. A weakness of the ASIA scale is that subtle neurologic disturbances may be subjectively missed or dysesthesias may be grouped into an ASIA category E.
A mechanistic classification of cervical spine injury was developed by Allen et al,5 based on a retrospective review of 165 injuries (Fig. 10.1). This classification is relatively complex and infers a mechanism based on interpretation of the static radiograph. Treatment can be deduced based on reversal of the mechanism and stabilization in such a way that prevents reproduction of the destabilizing force. However, the mechanism of injury does not always correlate with the resultant fracture pattern. Torg et al6,7 looked at the National Football Head and Neck Injury Registry and found that the same mechanism of injury can result in different fracture patterns depending on the level at which the injury occurs. Additionally, rotational forces are not well accounted for and can play an important role in the fracture pattern.8

The most popular cervical spine injury classification used in the literature is a simple descriptive one based on the pathoanatomy. Typical injury patterns include facet fracture dislocations (unilateral or bilateral), axial injuries (compression, teardrop, burst fractures), and extension fractures (teardrop avulsion, spinous process, lateral mass). With this system, however, the nuances of any particular group of fractures are difficult to distinguish, and these factors can have important implications in determining treatment.
The Subaxial Cervical Spine Injury Classification (SLIC) system was developed to incorporate each of the factors that guide the definitive treatment of the patient and includes injury morphology or major pattern of injury, the integrity of the diskoligamentous complex (DLC), and the patient′s neurologic status.9,10 Each group is broken down into subgroupings and assigned a grade. The grading scale is used to assign numeric values to the injury morphology, DLC complex, and neurologic compromise of the patient (Table 10.1). After adding the value assigned to each group, the classification system applies a total score in an attempt to guide treatment. The higher the score (greater than 4), the more likely the need for surgical stabilization and, with lower scores (less than 4), the patient can be treated nonoperatively. A score equal to 4 is considered indeterminate, with no specific recommendation for treatment. The SLIC was found to be reliable and valid with 93.3% inter-rater agreement regarding treatment recommendations for sub-axial spine injuries.9,11 Because this classification includes the neurology and appears to be a guide to treatment, it is gaining popularity.
Nonoperative Treatment
Many injuries of the lower cervical spine are treated nonoperatively. Nonoperative management can range from benign neglect to halo-vest immobilization. For example, most spinous process fractures and transverse process fractures are stable and amenable to simple hard collar immobilization for 6 weeks, or even comfort care only with soft collars or benign neglect. However, one must be aware that spinous process fractures can, at times, occur from a flexion mechanism rather than from the usual extension, muscle pull (classic clay shoveler′s fracture), or direct-blow mechanisms. When flexion is the mechanism of injury, a more substantial posterior ligamentous injury may coexist, creating a potentially unstable pattern warranting more aggressive management and possibly even surgical stabilization (Fig. 10.2). Either dynamic flexion/extension radiographs or magnetic resonance imaging (MRI) can be used to distinguish a flexion injury from a more benign extension injury. Isolated fractures through the transverse foramen are usually benign and treated with collar immobilization until it is clear that there is no other subtle instability, at which time treatment is symptomatic. One must be aware of the possibility of vertebral artery injury when the vertebral foramen is involved.12

Teardrop avulsion fractures are typically minor extension injuries involving a small fleck of bone pulled off the anterior inferior aspect of the vertebral body by the annulus. They are treated with collar immobilization for 6 weeks. The teardrop avulsion injury is not to be confused with the more ominous teardrop fracture that is the result of a compression-flexion mechanism (Fig. 10.3). The latter is also referred to as a quadrangular fragment fracture. It signifies a much more unstable injury that is only rarely treated nonoperatively. Distinguishing radiographic criteria of a teardrop fracture include a larger anteroinferior fragment (a chunk rather than a chip), posterior retropulsion of the cephalad vertebrae, widening of the facets, and a characteristic sagittal split in the vertebral body.

Source: Reprinted with permission from Vaccaro AR, Hulbert JR, Patel A, et al. The subaxial cervical spine injury classification system. Spine 2007;32: 2365–2374.
Lateral mass fractures vary in severity, and many can be treated successfully in a nonoperative fashion. The first factor to consider in determining the appropriate treatment is the neurologic status of the patient. If there is a spinal cord injury, it is assumed that the skeleton is unstable, and the patient is treated with operative stabilization. If there is only an isolated root injury, nonoperative treatment is possible, with anticipated recovery of the root deficit. If the fracture is minimally displaced or nondisplaced, then the root injury occurred at impact, and external immobilization in a rigid collar or more substantial bracing, such as sternal occipital mandibular immobilization (SOMI) or a Minerva brace should be utilized, followed by close radiographic observation for displacement. If the fracture displacement is extensive enough to compromise the neural foramen, creating residual root compression, then operative treatment should be considered. Such displacement is often a rotational problem that can be reduced with traction but tends to re-displace even with rigid halo immobilization.
In the case of facet dislocations, particularly bilateral facet dislocations, nonoperative management is not appropriate, because the posterior ligamentous injury does not tend to heal well enough to restore structural stability to the spinal column, and recurrent dislocation despite rigid external immobilization in a halo-vest is well documented.13 In one study, only 44% of the facet dislocations (unilateral similar to bilateral) treated with a halo-vest achieved clinical stability, and many of those that were stable had poor anatomic results.14 Interestingly, a poor anatomic result did not correlate with a poor clinical outcome. The exception to this generalization may be the case of unilateral facet dislocations. Occasionally, a patient who has a history of an untreated neck injury is found to have an old unilateral facet dislocation (Fig. 10.4). Because the opposite facet capsule is intact, the dislocated facet tends to “lock” into its new position, making it relatively stable. As long as bony integrity remains, the patient may be asymptomatic and may have suffered a new injury or requested evaluation for chronic neck pain. Spontaneous fusion of the injured facet can occur. In fact, in the past, viable treatment options have included simply allowing the facet dislocation to persist or reducing the dislocation followed by a period of external immobilization, but these approaches tend to have a higher incidence of late pain.

The role of nonoperative treatment of a unilateral facet fracture with minimal displacement is a topic that is currently controversial. Historically, this fracture was treated nonoperatively unless it displaced significantly. Some authors have attempted to use radiographic measurements to predict fractures that were more likely to displace, thus determining a role for early surgical stabilization. Spector et al15 evaluated 26 unilateral facet fractures treated nonoperatively, and found that if the height of the fracture was 1 cm or involved greater than 40% of the facet, then the failure rate exceeded 50%, and thus early operative management should be considered. Halliday et al16 used MRI to assess the degree of associated ligamentous injury as a predictor of future failure. If three of four ligaments (the facet region, the interspinous ligament, the anterior longitudinal ligament, and the posterior longitudinal ligament) were disrupted, then late displacement was likely. Clinical outcomes as measured with the Short Form 36 (SF-36) were also worse with nonoperative treatment in the series reported by Dvorak et al17; as a result these fractures are now being more closely scrutinized for operative stabilization. Future studies will be needed to shed further light on this controversy.
Fractures that result from axial loads, such as compression, burst, and teardrop fractures, can also be considered for nonoperative management (Fig. 10.5). Surgical treatment is more appropriate when there is a spinal cord injury. But if there is no spinal cord injury, then the degree of bony and ligamentous injury dictates how aggressive one should be with treatment. Defining stability can be difficult under these circumstances. If there definitely is a posterior ligamentous injury along with the anterior bony injury, then operative treatment is appropriate. Sometimes such an injury is apparent from plain radiographs or computed tomography (CT) scan, but an MRI may be helpful if some question remains. Nonoperative treatment should only be undertaken if the amount of deformity on the initial films is acceptable, because external immobilization is unlikely to maintain any correction in alignment achieved with longitudinal traction. These injuries tend to result in kyphosis, so any external support should apply a good extension moment and be fairly rigid. Cadaver studies have shown canal compromise to increase with compression and extension positioning, putting the neural elements at greater risk.18 Thus, the use of a SOMI, Minerva, or halo is the best option. In a direct comparison of halo-vest management versus anterior corpectomy with stabilization, Fisher et al19 noted greater residual kyphosis in the halo-vest group (11.4 vs 3.5 degrees) but were unable to correlate greater residual deformity with poorer outcomes. However, four of 24 patients required conversion to surgical treatment due to either loss of alignment or neurologic deterioration.

Indications for Surgical Treatment
General Indications
The goals of surgical treatment are essentially the same as the goals of nonoperative treatment: to achieve stability, to restore and maintain spinal alignment, to decompress and protect the neurologic elements, and to minimize late pain. Surgical management should be considered when it is unlikely that these goals can be achieved by nonoperative means. Although indications for surgical treatment focus on the fracture pattern and neurologic status of the patient, one must also take into account factors such as age, preexisting medical conditions, body habitus, additional injuries, patient compliance, and patient expectations or desires.
Neurologic Deficit
Neurologic deficit is the most common indication for surgery. When considering neurologic injury, one should assess whether it is a spinal cord deficit or nerve root deficit. Spinal cord injuries are further divided into incomplete or complete lesions. When the neurologic deficit is isolated to a nerve root, then the surgical indication is less strong. Because the root is a lower motor nerve, the potential for recovery is greater, even with nonoperative treatment. When root deficits are present in a spine fracture that is nondisplaced or minimally displaced, nonoperative management should be considered. An exception occurs when the associated bony injury is unstable and repetitive damage could result from continued motion at the fracture site. When the neurologic injury involves the spinal cord, it is generally presumed that the associated bony injury is unstable. Although a significant component of the neurologic injury occurs at the time of impact, there is often residual cord compression, and the risk of repetitive trauma from the bony instability is high. Thus, surgical intervention is indicated to relieve the residual pressure on the cord and prevent further damage. These principles hold true across the array of lower cervical injury patterns, but the means of achieving the desired effect varies with the injury pattern. For example, neurologic decompression in a facet dislocation can be achieved nonoperatively with traction to realign the bony elements, at which point surgical stabilization is performed to prevent re-dislocation and further neurologic injury. This situation is different from a burst fracture with retropulsion of bone onto the spinal cord. Under these circumstances, traction has a limited ability to decompress the cord, and complete decompression can only be achieved surgically by direct removal of bone from the canal.
Stability
Another reason for surgery is to achieve stability in a position of anatomic alignment. However, defining the degree of instability that warrants surgical stabilization is controversial. An important distinction is made between bony and ligamentous injury because stability can eventually be achieved with healing in a bony injury, whereas instability often persists in a predominantly ligamentous injury. Stability as a determinant for surgical stabilization is more specifically addressed in the following discussion of individual fracture patterns.
Facet Joint Dislocation
It has long been accepted that surgical stabilization is warranted in bilateral facet dislocation due to the degree of ligamentous injury.20 The treatment of unilateral facet dislocations is more controversial. Once reduced, a unilateral facet dislocation is more stable than a reduced bilateral dislocation. One factor determining stability after reduction is an associated fracture of either the superior or the inferior facet. With a facet fracture, re-dislocation occurs more easily. If reduction by traction occurs easily with low weights, then re-dislocation will also occur more easily. Rather than dislocating at the extreme of flexion, the dislocation can occur earlier with less flexion by anterior translation of the inferior facet on the superior facet. Without a fracture, the reduced facet is often reasonably stable; in fact, spontaneous fusion occurs in 50% of cases.21 Beyer and Cabanela22 compared patients treated nonoperatively with halo-vest immobilization to patients treated with posterior fusion. They found that anatomic reduction was achieved and maintained in only 25% of halo patients as compared with 60% of surgical patients; as a result, late pain was more frequent in the nonoperative group. Surgical fusion in these patients is indicated as a means of minimizing late pain. Our preferred treatment for unilateral facet dislocations is surgical stabilization, assuming no extraneous circumstances.
Burst and Teardrop Fractures
Because burst and teardrop fractures are the result of compression, the ligaments can be preserved, resulting in a predominantly bony injury. When a component of flexion is added to the mechanism of injury, there will be a greater degree of ligamentous disruption that increases the likelihood of late instability.23 When the patient is neurologically intact, the amount of deformity and the degree of ligamentous injury are the primary determinants of treatment. Unfortunately, many patients suffer neurologic injury with the fracture, which is an indication for surgery because it enables the canal to be decompressed. The presence of neurologic injury indicates that more energy was imparted on the spine, increasing the likelihood of instability. In a review of surgical versus conservative treatment of burst and teardrop fractures, a statistically significant neurologic recovery (as measured by the American Spinal Injury Association [ASIA] scale), canal restoration, and overall sagittal alignment were noted in the surgical group.24,25 Even if the neurologic injury is deemed complete, aggressive decompression is warranted to improve the possibility of regaining root function caudal to the level of injury.26,27
Floating Lateral Mass Fractures
Lateral mass fractures seldom present with spinal cord injury. The majority of neurologic deficits associated with this fracture pattern are single-root injuries; thus, the neurologic deficit itself is usually not an indication for surgery. These injuries are often difficult to detect on plain radiographs and are typically identified on CT scans. However, the degree of instability is based on the severity of associated ligamentous and disk disruption, which is best seen on MRI scans. Instability in these injuries is generally rotational, which is difficult to control nonoperatively, even in a halo vest. If there is greater than 15% subluxation or greater than 10 degrees of angulation on plain radiographs or CT, enough instability can be implied to warrant surgical stabilization.14 Such small amounts of displacement may not be noted on the original films, so Halliday et al16 postulated that the degree of instability could be predicted by the amount of ligamentous involvement noted on MRI. They concluded that operative stabilization should be considered if at least three of the following ligaments were involved: the facet capsule, the interspinous ligament, the anterior longitudinal ligament (ALL), and the posterior longitudinal ligament (PLL).
Initial Management of Lower Cervical Spine Subluxations and Dislocations
Cervical facet fractures and dislocations account for ~ 6% of all cervical spine fractures.28 Facet injuries occur most commonly in the lower cervical spine at C5-C6 (25 to 60%) and C6-C7 (25 to 30%).17,29 Some in vivo studies attribute this injury distribution to the anatomic difference in facet orientation as well as size. Cervical flexion-distraction injuries can be broken down into four groups with increasing severity: facet subluxation, unilateral dislocation, bilateral dislocation with 50% displacement, and complete displacement. In an animal study, Delamarter et al30 showed that early decompression improved neurologic outcomes. In the clinical setting, there is also a growing trend that early decompression of spinal elements improves neurologic outcome.28,31–33 In facet dislocations, decompression of the canal can be rapidly achieved with closed traction reduction. Once accomplished, surgical stabilization can be performed less emergently.
The most widely accepted method of closed reduction for facet dislocations is the use of skeletal traction. There has been a great deal of controversy surrounding the safety of closed reduction. Immediate skeletal traction reduction can be performed safely but requires an awake, alert, and cooperative patient in whom a neurologic exam can be performed.34 Additionally, one must be able to visualize the involved cervical spine segment on radiography or fluoroscopy and lack contraindications to Gardner-Wells tong application such as a skull fracture underlying the insertion site.
Usually the reduction performed in the emergency department immediately after the injury is clarified radiographically using plain radiographs or CT scanning. This step has the most urgency if the patient is neurologically impaired, particularly in those patients with incomplete neural deficits.28,35 Some surgeons suggest obtaining an MRI prior to closed reduction with plans for an open anterior cervical diskectomy if a herniation is identified.36 However, the current literature does not indicate that the finding of a herniated disk is clinically significant prior to awake, closed reduction.37,38 For reduction of facet dislocations, Gardner-Wells tongs are positioned with the points in line with the external auditory meatus. They can be positioned slightly anterior or slightly posterior, depending on whether extension or flexion, respectively, is desired. Patient positioning can also be used to maintain a flexion or extension moment during traction.39 Care is taken to ensure that the points of the tongs are below the maximum circumference of the skull to avoid cephalad migration of the tongs, resulting in loss of fixation. In addition, one should ensure that a patient with concomitant closed head injuries has not sustained a skull fracture that would compromise pin position. This area should be sterilized and anesthetized; remember to anesthetize not only the skin but also the periosteum. The indicator pin will determine the appropriate amount of pressure to be applied to the skull. Once the indicator pin is flush with the skeletal traction pin, the appropriate skull pressure is achieved.
Initially, a weight of 10 to 15 lb is applied to accommodate the weight of the head. After this initial traction is applied, a cross-table lateral radiograph should be obtained to ensure that excessive distraction does not occur at the facet dislocation or that an occult concomitant injury such as occipital cervical dissociation is present. Weight is then added in 5- to 10-lb increments, followed by serial neurologic exams and repeated cross-table lateral radiographs. Mild sedation is useful to relax the patient, and adequate time should be allowed between radiographs to allow the patient to relax the neck muscles. It is critical that the patient remains alert and cooperative throughout the procedure to facilitate an effective neurologic exam. The surgeon continues to add weight until any of the following occurs: reduction is achieved, two thirds body weight has been applied,40 the injured or adjacent segments are over-distracted, or the patient reports neurologic complaints or excessive pain. Clinical and cadaveric studies both support traction weights up to 140 lb.41,42 If heavy weight is to be utilized, then standard steel Gardner-Wells tongs should be used because carbon-fiber MRI-compatible tongs typically are limited to 60 lb. Applying traction with some flexion aids in unlocking bilateral facet dislocations; once reduction is achieved, then the neck can be extended to maintain anatomic alignment and the weight is decreased to 10 to 15 lb.
Most bilateral facet dislocations can be reduced prior to surgery with this technique. Unilateral facet dislocations may be more difficult to reduce because of their tendency to “lock,” as noted earlier. Sometimes gentle manipulation into flexion with rotation away from the dislocated side can aid in final reduction once traction has been applied to distract the facet into a perched position.39 When a neurologic deficit develops during the course of reduction with traction, it should not be assumed that the etiology is a herniated nucleus pulposus. In this situation, an MRI is warranted to accurately diagnose the cause and plan the surgical approach before proceeding to the operating room. Ludwig et al43 describe a case of epidural hematoma that developed during reduction, resulting in a progressive neurologic deficit. However, in this case, the neural compression was found to be posterior to the cord, warranting a posterior laminectomy rather than an anterior diskectomy.
Some debate remains on performing an MRI prior to closed reduction. This controversy is due to the fear of displacing a herniated disk posteriorly into the spinal canal, causing an iatrogenic spinal cord injury. There are basically three clinical scenarios to consider: (1) the patient is neurologically intact, (2) the patient has an incomplete neurologic injury, and (3) the patient has a complete neurologic injury.
One could argue that the patient who is neurologically intact has little to gain from immediately reducing the facets, and obtaining an MRI may avoid potentially displacing a herniated disk into the canal. On the other hand, leaving the patient dislocated for a longer period of time with the required transport and transfers to obtain a pre-reduction MRI also carries a potential risk of neurologic deterioration. The incomplete spinal cord–injured patient perhaps has the most to gain with rapid canal decompression achieved by immediate traction reduction, but, conversely, already has a damaged cord that may be more sensitive to further canal compromise from disk herniation. Finally, patients with a complete neurologic injury have little to lose with an immediate attempted reduction of a malaligned spinal canal and everything to gain, so they are the most ideal candidates for traction reduction.
In the patient with an axial load injury, traction can also be considered to improve alignment and achieve some secondary canal decompression. This treatment should only be considered a temporizing measure because full evacuation of the spinal canal can only be achieved surgically. Thus, it is reserved for those patients who have contra-indications to proceeding directly to the operating room. When utilized, only enough weight to control the head and grossly align the neck is used.
Tips and Tricks
Prerequisites to attempting closed reduction:
An alert patient in whom a neurologic exam can be performed
Ability to visualize the involved cervical spine segment on radiography or fluoroscopy
A patient who is not obtunded and does not have a distracting injury
Ability to safely apply skeletal traction (no skull fracture)
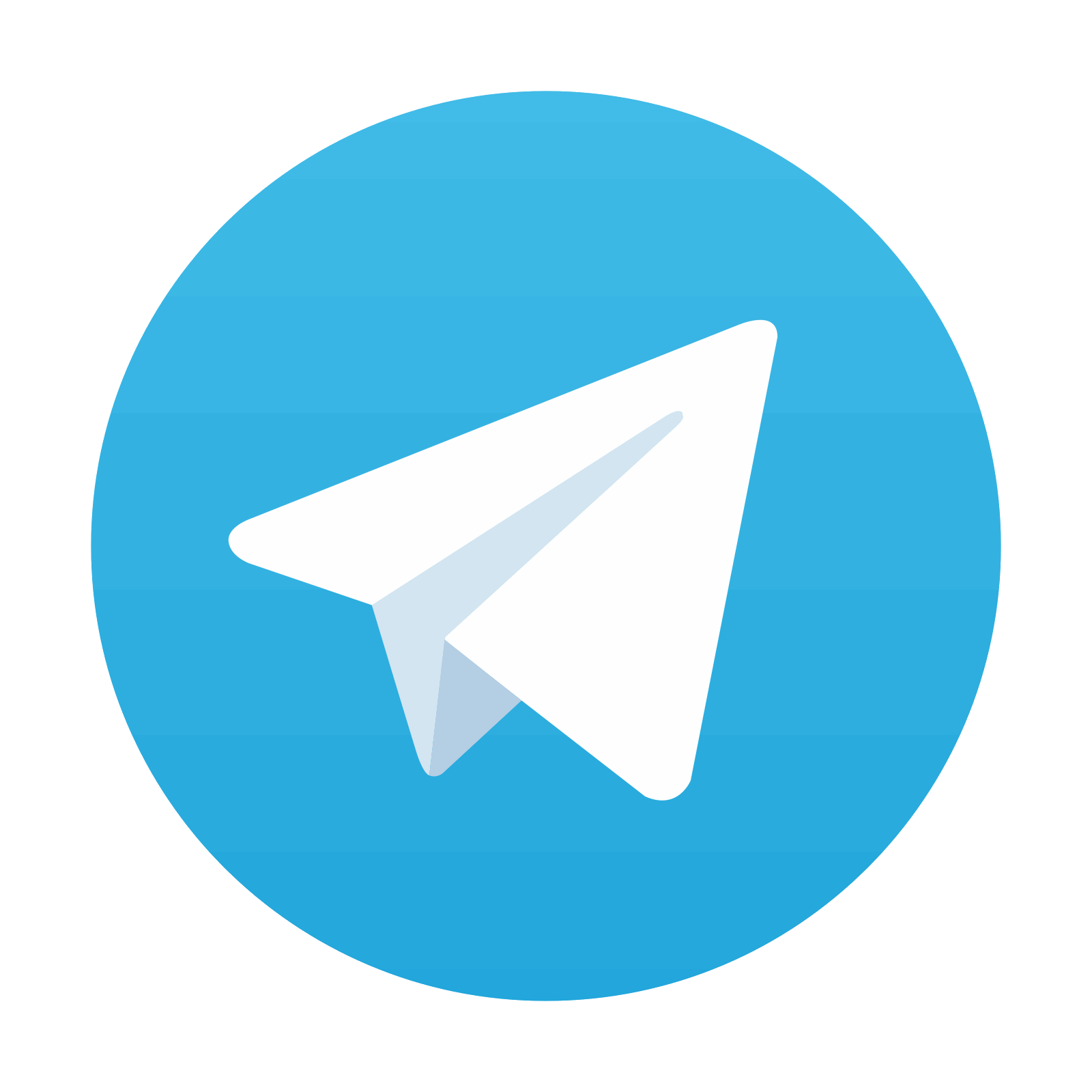
Stay updated, free articles. Join our Telegram channel

Full access? Get Clinical Tree
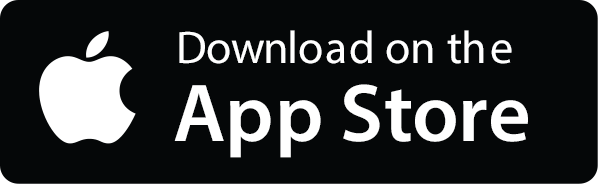
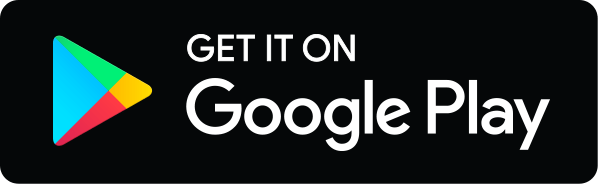
