Fig. 25.1
The detector device used to monitor relevant object in the operating field, collecting real time data relating to their location and orientation is shown. It detects the joint and the instruments position and need to have visual contact with them. Depending on the technology they use, these tracking systems can be mechanical, optical, electromagnetic or ultrasonic
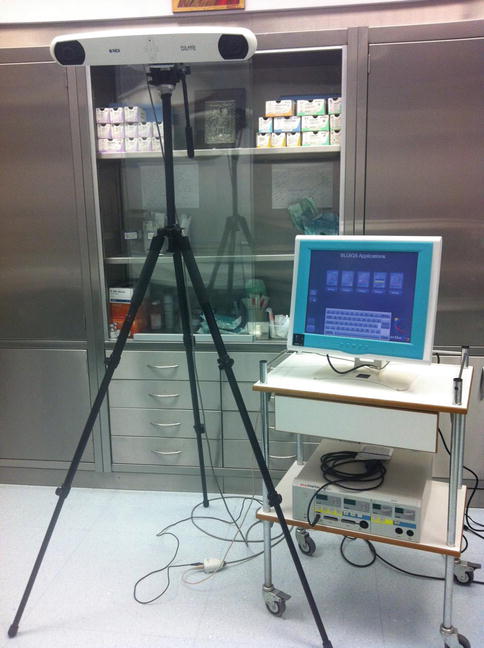
Fig. 25.2
The display device showing the virtual scenario, updated in real time is shown
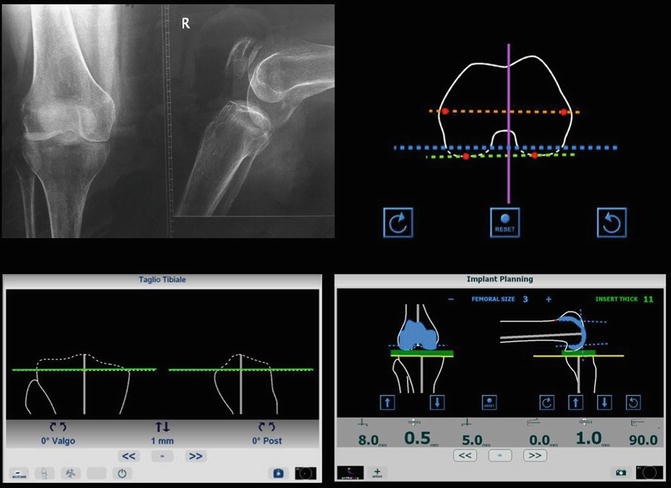
Fig. 25.3
When data collected and processed by the tracking system is completed a virtual scenario is produced. The images appear at the display device and the surgeon can perform the preoperative planning for the orientation of osteotomy and for prosthesis template
All these systems allow a preoperative or intraoperative planning of the surgery based on patient’s specific information, permitting the simulation of different surgical strategies and to choose the optimal one. In case of preoperative planning, during the intervention, the planned strategy can be displayed in the virtual scenario and integrated with intraoperative information. During the intervention, providing visual and numerical information of the position of surgical tools and patient’s anatomy is updated in real-time, these systems give to the surgeon an accurate visual feedback, guiding him towards the completion of the planned strategy. These systems have the ability to provide more visual information in the surgical field than is available to the naked eye and help in solving the problems of visualization especially in case of minimally invasive surgery. It is well known that less invasive surgery contribute to rather better patient outcomes, but minimization of invasiveness generally results in a lack of surgeon’s perception and dexterity. Showing the structural anatomy of the patient together with surgical tools, these augmented reality navigation systems allow the surgeon to accurately localize anatomical areas and to reach predefined positions watching at the display device.
Several orthopaedic procedures lends themselves well to the use of augmented reality navigation systems. Most common tasks developed by existing navigation systems are: joint replacement, arthroscopic surgery, fracture treatment and spinal surgery.
As proposed in Picard et al. [38], surgical navigation systems can be divided in: (a) systems using preoperative models. They can be either from preoperative three dimensional anatomy models reconstructed from CT/MRI images of the femur and of the tibia of the patient (patient specific) or generic anatomical model of the part in exam (non patient specific). By examining the imaging modalities on which the existing systems are based, one can identify two antithetic trends. The first one is patient oriented and tends to exploit the most advanced devices and techniques to reach excellent quality standards. CT and MRI based systems generally go in this direction and are very powerful, accurate and expensive. The other trend is focused on simplicity and accessibility. Conventional X-ray and fluoroscopy based systems are often less accurate, but affordable for most potential users. The choice of a specific imaging modality is frequently linked with that of a particular registration approach [39]. The image based navigation system has the advantage of precise preoperative planning, but the additional radiation, cost of preoperative CT scans, and additional time for preoperative planning do not improve accuracy [40]. (b) Systems using intraoperative models. They help the surgeon to achieve the planned implant. The knee joint, the data collection probe, and the implant insertion tool are sensorized with securely affixed optical targets and, after the registration and calibration process performed using a particular procedure, they can be localized and tracked in real time (Fig. 25.4). They use intraoperatively acquired medical images to determine the model (image based), and systems using models derived from information determined with direct measurement of the bone surface or limb dynamics (non image based). During surgery, using the localizer, the surgeon collects points on tibial and femoral surfaces, and statistical information about the shape variation of the femur are used to interpolate the data points. The positions of all sensorized objects, including the patient’s anatomy, are tracked in real time by an optoelectronic localizer and reproduced in the virtual scene. Then, through the registration process, the system matches the ideal fitting between acquired points and the generic model. Using a statistical method to build the model has the advantage of requiring less data points to obtain a sufficient interpolation accuracy, reducing intraoperative time required for data acquisition [41]. Finally, to be accepted by surgeons, equipment have to be small unobtrusive, user friendly, safe and compatible to the surgical environment. Adding any equipment in the operating room increases the risk of infection, so any part of the system that comes into contact with the sterile field must be easily sterilized or draped. The digitalization of the bony landmarks is one of the crucial steps in navigation. Problems of reproducibility with intraoperative termination of these landmarks have been described to especially appear with the femoral epicondyles [42, 43].
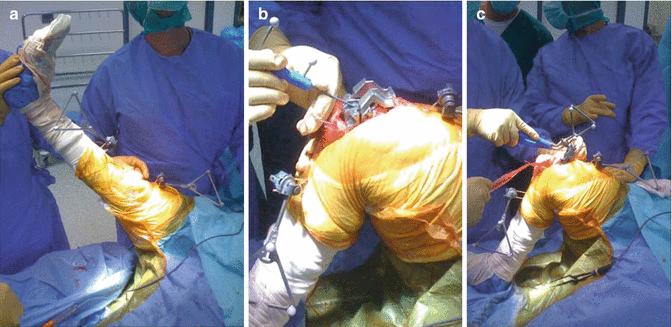
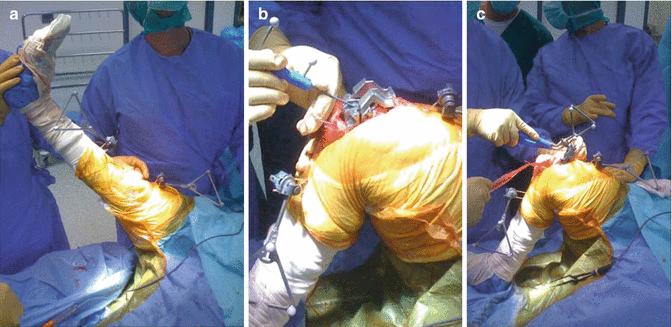
Fig. 25.4
At the patient side the surgeon has to place bone markers on femur and tibia. (a) After that, using the data collection probe, he has to define a list of specific landmarks. (b) Finally according to his preoperative planning he can use the sensorized cutting blocks and perform the osteotomies, while they can be localized and tracked in real time (c)
Clinical Data
In order to evaluate the advantages of navigated TKA over conventional TKA we need to look very critically at the percentage of radiographic outliers in coronal and sagittal plane alignment, the accuracy in component axial rotation, the improvement of flexion extension gap and ligament balancing, the operative times once experience is gained, the costs, the complication rates, the duration of learning curves, the postoperative functional scores and the survival of TKA implants as a result of application of a new surgical technique.
According to recent literature, several studies have demonstrated that computer assisted navigated TKA achieves straight mechanical leg axis in coronal plane (on full length standing radiographs of the lower extremity) within the range of 3° of deviation [44–67]. Moreover the number of limb alignment outliers is reduced compared to traditional mechanical instrumented TKA [35, 40, 46, 49, 63, 68–70]. However, all studies between image based navigation and conventional techniques [40] groups were similar in early clinical outcomes as range of motion, knee scores, and postoperative complication rates at the final follow up. There were no statistical significant differences between the study groups. Moreover many of the reports supporting navigated TKA include small cohorts with a relative short follow up and present low levels of evidence [40, 71].
Improved alignment in navigated TKA in the coronal plane and a reduction in radiographic outliers have been demonstrated in most of the reports which compare the two groups. Despite this fact, an improvement in clinical function scores, revision rates, or improved survival for TKA performed with navigation compared to conventional techniques has not been demonstrated [69]. There are many studies which do not provide functional follow up data, but only report less than 2 years clinical outcome and radiographic data. Clearly these studies, although providing useful feedback to surgeons about radiographic and alignment results, do not add to the body of evidence in favor of navigated TKA in terms of the question of long term functional improvements and lower revision rates. Generally there is no evidence from mid to longer term studies which supports functional improvements or reduced revision rates for navigated TKA. On the other hand, even published meta-analyses studies cannot agree on the concept of whether there is evidence to support any functional improvements in navigated TKA [69]. Different methods of statistical analyses, incomplete power calculations, and cohort studies combined together lead to confusing and contradictory results.
Moreover, many of the studies have been performed in high volume surgical centers by surgeons with an interest or even conflict of interest with industry and the development of navigation technology and who perform many TKA procedures already. Although, there is evidence to support improvements in coronal plane alignment, however, sagittal plane and axial/rotational alignment have been less well studied. The accuracy to adjust the rotational alignment of the femoral component is a further prerequisite to avoid malfunctioning in TKAs. It is well known that even small deviations of rotational alignment of the components have a considerable influence on patellar tracking, stability and on the overall biomechanics of the joint [42]. Diagnosis of malrotation is challenging enough since it usually requires computed tomography (CT), bony landmarks (usually the femoral epicondyles) and special software to reduce the imaging artifacts which can provoke imprecision with and without navigation [42, 43].
Computer assisted navigation systems have been designed in order to increase precision of the implantation of TKAs [46, 49, 68]. This could not explain the fact that the improvements in coronal alignment (with fewer outliers) have not produced improved clinical knee scores, implant survival, better TKA function, or durability. It may be attributed to three potential causes: (1) better alignment in two planes is mitigated by the remaining errors in the axial (rotational) plane either because of an incorrect definition of the navigation system landmarks or by the shear malalignment of the components in the axial plane; (2) alignment goals of a neutral mechanical axis are not the correct target, and individual adjustments need to be made based on each patient’s anatomic variability; and (3) the groups studied are too small (insufficient power) and/or the clinical scoring systems measuring functional status are not refined enough and suffer early ceiling effects, not allowing to prove superiority.
Navigated TKA requires additional steps to be taken in the operative room for computer processing, pin and tracker placement, array registering of data points, and analysis of intraoperative data. This increase in operating room time is variable and ranges between an increase of 8–63 min and to a nearly double or more than double the procedure time, with a higher incidence of complications because of small but consistent errors in navigation landmarking, compared to conventional TKA [46, 69, 72, 73]. Surgeons may rely on the navigation, perform minimal or not enough bone resections, and prolong operating room times even further. Soft tissue balancing can not be directed from computer assisted navigation systems. Another potential source of error is when the use of the navigation system is limited to the bone cuts and is stopped before final cementing of the implants. Finally, the volume of the operations performed by the surgeon and the experience in using computer navigation technology might be contributing factors [69].
Several authors have studied major complications related to computer navigation and TKA. They present different results: Bauwens et al. [46] found no difference in infection and thromboembolic events. Church et al. [74] performed a double blind randomized study to compare the incidence of fat embolic phenomena between navigated and non navigated knee prosthesis and demonstrated a significant reduce in embolic events in the CAS group. Fat and bone marrow is a potential activator of the clotting system and is thought to be an important factor for deep venous thrombosis.
In all studies comparing navigated TKA to conventional TKA, the cost of using a navigated TKA system is a factor that is well recognized yet difficult to quantify. Cost is often addressed indirectly with an increase in operative and procedure time for navigated TKA [75]. The use of computer assisted navigation causes costs, including the cost of the navigation system and a prolonged operative time [76]. These costs are justified if there is a benefit for the patient. It has been suggested that the use of a navigation system might be cost effective if there are decreased revision rates [77]. However, improved long term function, lower revision rates and/or survival, are not supported by any currently available evidence data [69].
TKA is one of the most successful procedures in terms of functional improvement, quality of life and cost effectiveness [78, 79]. It is therefore difficult for any new technique to further improve these results. Although many of these studies do show improvement in radiographic outliers, they correctly suggest that these improvements have not yet translated to improved knee function, quality of life, and survival of the implant [71]. One can argue that it may take a longer time to show a possible difference [71]. These findings are not sufficient enough in order to conclude that surgical navigation has to be abandoned. Surgeons, who perform relatively few TKAs, should also be cautious about adopting navigated TKA. Navigation is not a substitute for meticulous intraoperative surgical technique and training in TKA.
The established roles for navigated TKA include use in patients with extra articular deformity or retained implants and hardware that does not allow for traditional extra or intramedullary alignment guides. In addition, its use in resident teaching to provide immediate feedback regarding the accuracy of cutting guide placement may be helpful. In order to effectively evaluate clinical outcomes of navigated TKA, future clinical trials should be designed in order to follow patients in short and mid terms documenting improved clinical function and in long terms establishing whether lower revision rates are achieved. For the above reasons the main questions for knee arthroplasty surgeons still remaining to be answered is how to create, modify, and identify knee functional assessment tools, imaging techniques, and reliable component alignment parameters to determine the benefits of navigated TKA. Therefore, more sensitive evaluation tools may be necessary.
References
1.
Lombardi AV, Berend KR, Walter CA, Aziz-Jacobo J, Cheney NA. Is recovery faster for mobile-bearing unicompartmental than total knee arthroplasty? Clin Orthop. 2009;467:1450–7.PubMedCentralPubMedCrossRef
2.
Horwitz MD, Awan S, Chatoo MB, Stott DJ, Powles DP. An 8- to 10-year review of the Rotaglide total knee replacement. Int Orthop. 2009;33:111–5.PubMedCentralPubMedCrossRef
3.
Parsch D, Krόger M, Moser MT, Geiger F. Follow-up of 11–16 years after modular fixed-bearing TKA. Int Orthop. 2009;33:431–5.PubMedCentralPubMedCrossRef
4.
Ang DC, Tahir N, Hanif H, Tong Y, Ibrahim SA. African Americans and Whites are equally appropriate to be considered for total joint arthroplasty. J Rheumatol. 2009;36:1971–6.PubMedCrossRef
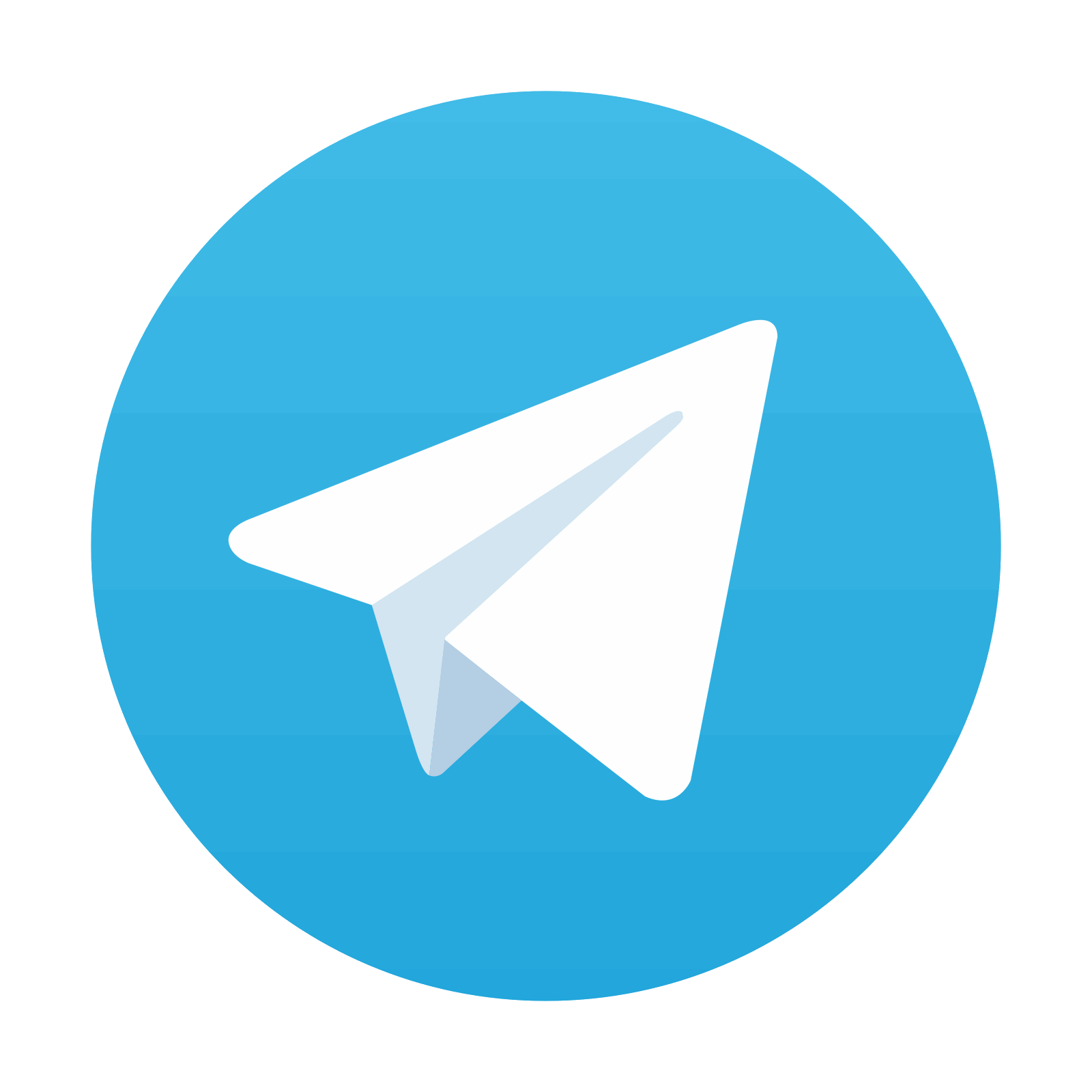
Stay updated, free articles. Join our Telegram channel

Full access? Get Clinical Tree
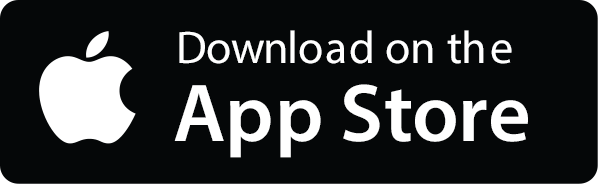
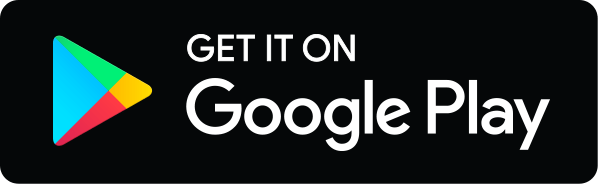