Fig. 10.1
Bilateral severe valgus and varus knee deformity, (a) preoperative radiographs, (b) postoperative radiographs showing satisfactory correction
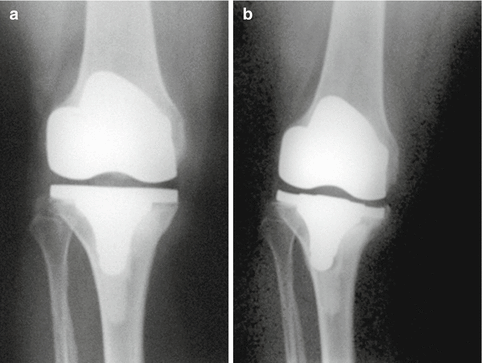
Fig. 10.2
The effect of varus placement of the tibial component, (a) immediate postoperative radiograph, (b) early aseptic loosening and varus drift of the component at 5 years follow up
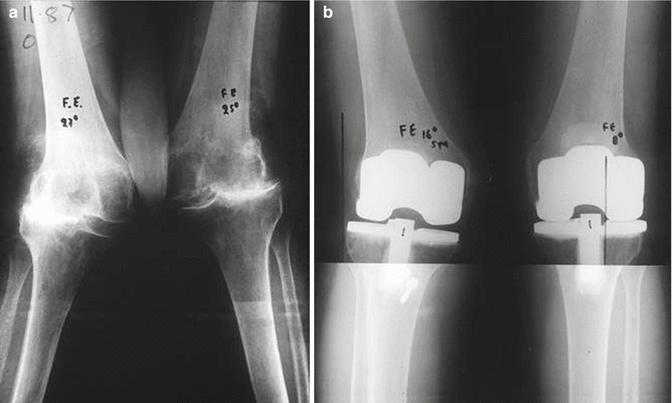
Fig. 10.3
Bilateral valgus knee deformities, (a) preoperative radiographs, (b) postoperative radiographs of well functioning TKA with valgus under correction of the right knee
The Effect of Preoperative Deformity and Soft Tissue Contractures
In varus knee deformity (Fig. 10.4), which is the most common of the osteoarthritic knee deformities, the medial structures are contracted. These structures include the deep and superficial medial collateral ligament, posterior and posteromedial capsule and posterior cruciate ligament, depending on the severity of the deformity. Whiteside has shown [25] that, when the knee is tight in flexion only, the superficial medial collateral ligament is mainly responsible for the contracture, while when it is tight in extension, the deep medial collateral ligament is the cause of contracture. When the knee is tight in both flexion and extension, all the above structures contribute to medial tightness and need balanced release [26]. In order to restore the tibiofemoral axis it is necessary to remove the osteophytes from the upper lip of the medial tibial condyle and the medial femoral condyle in combination with a balanced release of the medial structures. The deep medial collateral ligament is sub periosteally elevated from the upper medial tibial condyle and its detachment can be extended to the posteromedial part of the condyle (if needed). The superficial medial collateral ligament is next sub periosteally elevated with the use of an elevator or a curved osteotome and, finally, even hamstring insertion may be necessary to elevate it. In severe varus deformities the correction is facilitated by the removal of the posterior cruciate, or by detachment of its insertion from the posterior tibia, as it has been shown that a contracted posterior cruciate contributes to the deformity on the coronal plane [27]. The procedure is performed stage by stage and the alignment and stability is tested after each surgical step. The goal is to achieve a normal laxity of 2 mm as estimated by a symmetrical joint opening of both the medial and lateral sides. Fixed flexion deformity is corrected by careful removal of the posterior osteophytes using a curved osteotome, and further release of the posterior capsule from its insertion in the posterior part of the condyles.
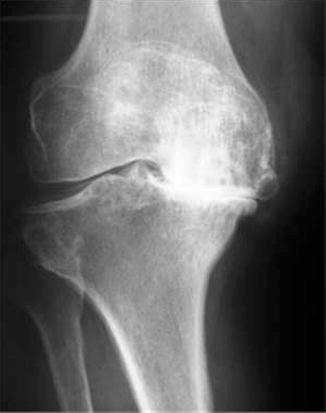
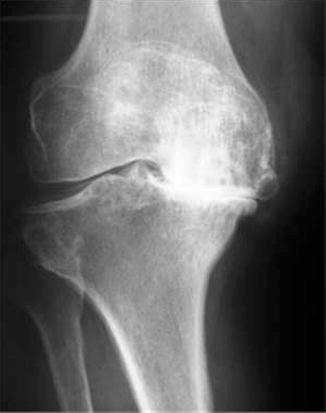
Fig. 10.4
Radiographs of a varus osteoarthritic knee joint
Valgus knee deformity (Fig. 10.5) is less common than varus. It accounts for 10–15 % of all knee deformities, and is more difficult to correct. It has been reported that TKA in knees with severe varus deformity results in superior outcome when compared to those of knees with severe valgus deformity. This superiority has been attributed to incomplete tibiomemoral axis correction in the valgus knees [28]. Bone deformities and soft tissues contractures are also encountered and are responsible for surgical technical difficulties during balanced restoration of the axis. In contrast to the varus knee, where the medial compartment is distorted, in valgus knees the lateral femoral condyle is usually mainly affected, being hypoplastic posteriorly and distally.
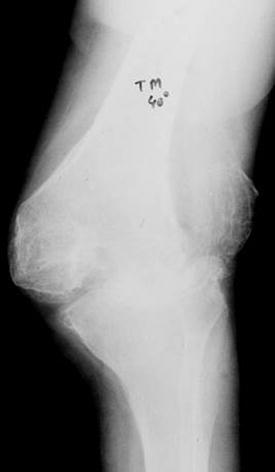
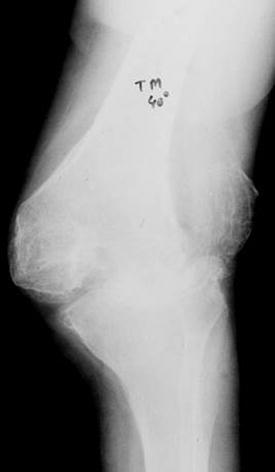
Fig. 10.5
Radiographs of a valgue osteoartheitic knee joint
The lateral stabilizing structures are contracted and require balanced release. These elements include capsule-ligament units (lateral collateral ligament, posterolateral and posterior capsule, and posterior cruciate ligament) and musculo-tendinous structures (iliotibial band, the popliteal tendon, lateral gastrocnemius and biceps tendon). Several techniques for release of the contracted elements have been suggested, but no standardized method has been established [25, 26, 29]. It has been shown that the iliotibial band and the posterolateral capsule are mainly responsible for tightness of the knee in extension, while the popliteal tendon and the lateral collateral ligament are mainly responsible for tightness in flexion [30]. During correction of the deformity and balanced release of the structures, the iliotibial band is first detached from Gerdy’s tubercle, if the knee is tight in extension, then the popliteal tendon and the lateral collateral ligament are subperiosteally elevated from the lateral femoral condyle in order to overcome tightness in flexion. The posterolateral capsule can be released using the “pie crust technique” or with a horizontal cautery cut at the level of the joint line, if further correction is necessary, in order to achieve balanced correction of the deformity [31]. The Posterior cruciate ligament can be released at its tibial insertion, or even resected, if further correction is required. Release of the posterior cruciate ligament further contributes to appropriate alignment in the coronal plane [31]. In order to restore the mechanical axis in severely deformed valgus knees, other techniques have also been proposed such as approaching the knee through the lateral side with or without tibial tubercle osteotomy. It is claimed that this procedure facilitates the balanced release of the contracted elements [32–34]. In severely deformed knees, the extensive release of the structures, which is required for correction of the deformity, may lead to instability. In such cases a constrained implant design should be used. In cases with an incompetent medial collateral ligament a constrained implant design should also be used. Sliding lateral femoral condyle osteotomy is another proposed technique for valgus correction, as the osteotomised fragment moves distally with the lateral contracted elements attached on it. Distal transposition releases tension and fixation with screws is then performed [35]. A thorough search of the literature has revealed that papers related to balanced release of the contracted structure describe the technique in only a relatively small number of patients and little data is given regarding the effect on the long term outcome of the TKA.
The Effect of Restoring the Joint Line and Balancing the Extension and Flexion Gap
Joint line height should be corrected with appropriate restoration of both femoral and tibial bone defects and balanced release of contracted structures in both the coronal and sagittal plane. In order to achieve this excessive removal of bone during femoral and tibial osteotomies should be avoided. The attempt to balance large extension and flexion gaps with the use of thick polyethylene only is the usual cause of joint line elevation (Fig. 10.6). Mid and long term studies have shown inferior outcomes and a high incidence of complications in TKAs in which there is a deviation from the anatomic line of more than 5 mm [36]. Posterior offset of the femoral implant should ideally be equal to the preoperative posterior offset of the femoral condyles (sagittal plane), in order to restore the joint line in flexion (Fig. 10.7). In order to avoid residual instability it is very important to have symmetric balancing of the collateral ligaments and equal flexion and extension gaps (Fig. 10.8). Minimal mediolateral laxity of 1–2 mm in extension, and equal flexion and extension gaps (tested in extension and with the knee at 90° of flexion when lifting up the femur and rotating the tibia) are considered the ideal result which enhances long term outcome [37]. If flexion is tighter than the extension gap and a posterior cruciate retaining implant is used, an attempt to increase the posterior tibial slope may solve the problem. If a tight flexion gap is due to a tight posterior cruciate ligament only and not to tight collaterals, partial release of the posterior cruciate ligament insertion from the tibia could be an helpful intervention.
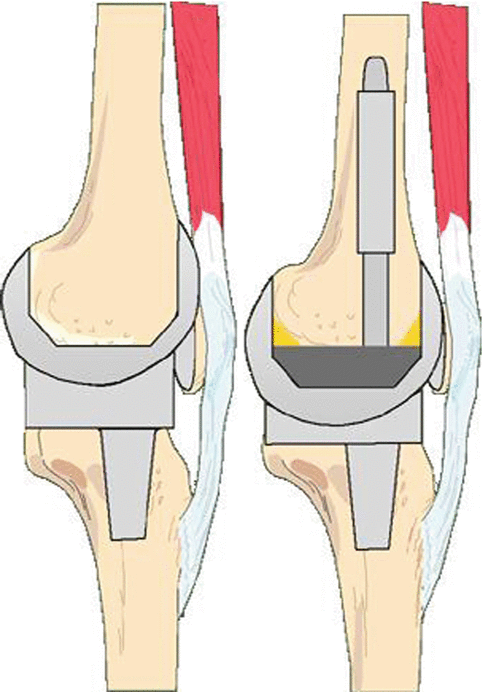
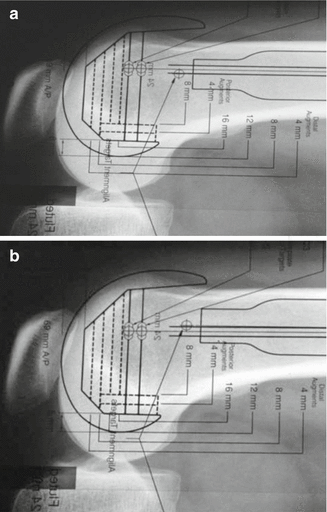
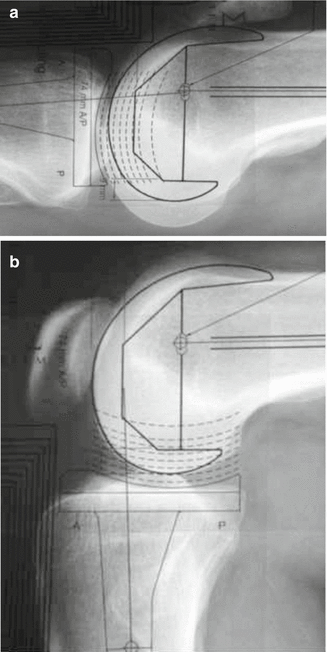
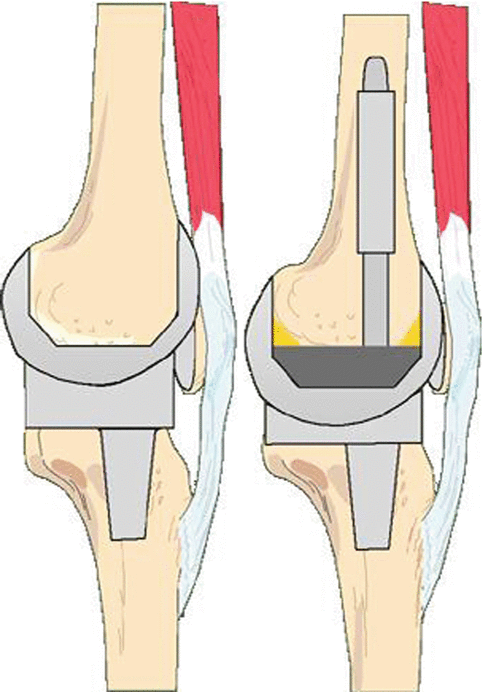
Fig. 10.6
Elevation of the joint line using a thick polyethylene liner (left). In order to avoid this effect, bone defect should be reconstructed (right)
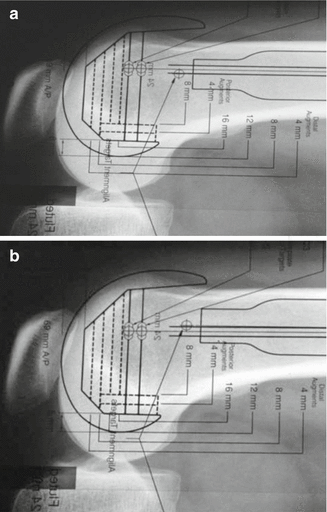
Fig. 10.7
The effect of anterior offset (a) and posterior offset (b) on the flexion gap and anterior patellofemoral overstuffing is shown
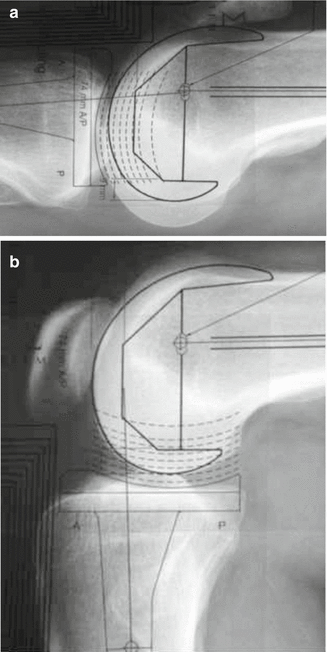
Fig. 10.8
The same component – polyethylene composite thickness in extension (a) and flexion (b) ensures equal soft tissue balancing and stability
Ligamentous imbalance or excessive posterior tibial slope has been shown to cause increased translational and rotational moments leading to a higher concentration of stresses on the joint surfaces and further subluxation [38]. Residual instability related to surgical technique may lead to high stress concentration and excessive polyethylene wear compromising clinical outcome and longevity of the TKA. Thus when dealing with severe knee deformities, surgeons should be prepared to use implants with higher degrees of constraint. In cases of inflammatory arthritis, such as rheumatoid arthritis, posterior cruciate retaining TKA implants should be used with caution, anticipating the possibility of later rupture of the posterior cruciate ligament due to disease activity resulting in pain, effusion and anteroposterior instability [39].
The Effect of Appropriate Anteroposterior and Rotational Placement of the Components
Bone resection from the distal and posterior femur and proximal tibia creates a flexion and an extension gap. Equalization and balance of these gaps is very important for the outcome of the procedure. Instrumentation systems used for femoral bone cuts are either anterior referencing, posterior referencing or both (Fig. 10.9). With posterior referencing systems, if implant size is to be changed, the anterior cut of the femur is altered while the posterior femoral condyle cut remains unchanged. Thus downsizing the component may cause anterior notching, while upsizing may lead to overstuffing of the patellofemoral space.
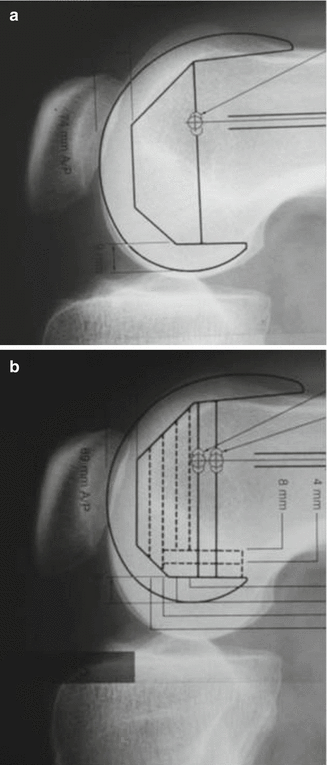
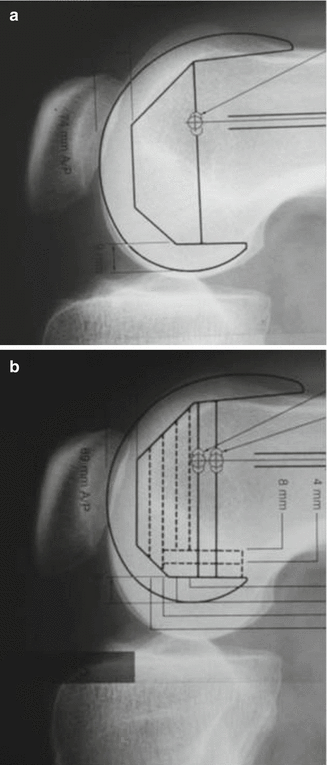
Fig. 10.9
The effect of up (a) and down (b) sizing of the femoral component on the flexion gap is shown
With anterior referencing systems, the selected anterior femoral cut is not affected by up or downsizing the guide, while the thickness of the bone to be resected from the posterior condyles is changed thus affecting the flexion gap.
Equally important is also the appropriate rotational placement of both components. Malrotation of either the tibial or femoral component will cause abnormal patellofemoral tracking and asymmetrical gaps, with undesired consequences. Correct rotation of the femoral component will allow the patella to slide in the groove without high strain, from full extension to full flexion. The transepicondylar axis is suggested and commonly used as a guide for this purpose, as it has been shown that it is 3–5° externally rotated in relation to the posterior condylar line (Fig. 10.10). However, the centers of the epicondyles are often difficult to define, because of the bulk of soft tissues and their broad bases [40–42]. More accurate positioning can be achieved by using the anteroposterior axis, which is the line joining the deepest point of the trochlear groove and the most lateral edge of the posterior cruciate ligament femoral insertion, as described by Hanada and Whiteside and called “Whiteside line” [43, 44] (Fig. 10.11). Insall and Scott have proposed the flexion gap technique, consisting of cutting the posterior condyles in a fashion parallel to the tibial cut, with the knee flexed at 90°, after balancing the collateral ligaments [45]. With this technique, the femoral component is routinely implanted in slight external rotation of the femur [46]. Another drawback is that ligament release is performed in extension and bony cuts in flexion. If the posterior condylar line is used as a guide for rotational positioning of the femoral implant, asymmetric wear of the posterior parts of the condyles often seen in valgus deformed knees should be taken into consideration in order to avoid undesirable internal rotation of the component and subsequent patellar instability [47]. Tibial tray rotation also affects patellofemoral alignment and thus excessive internal rotation should be avoided (Fig. 10.12). The central point of the tray must be in line with the junction of the inner and medial third of the tibial tuberosity [48].
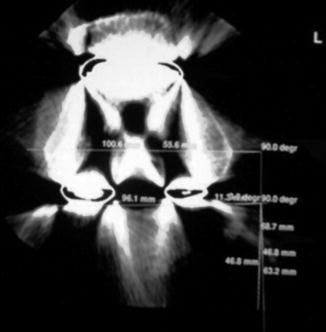
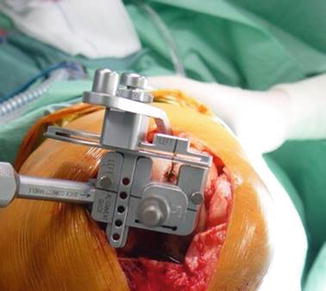
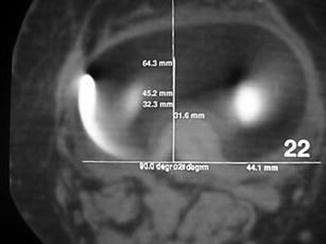
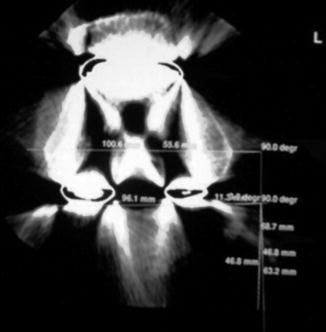
Fig. 10.10
Transverse CT-scan section showing that femoral component is rotationally aligned parallel to the transepicondylar axis
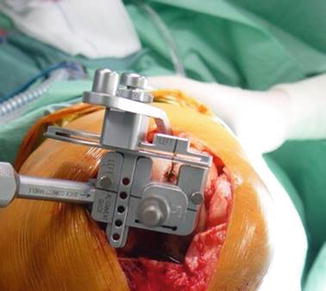
Fig. 10.11
Femoral cutting jig aligned parallel to the (Whiteside) line
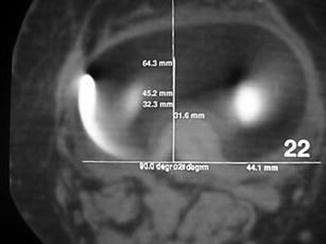
Fig. 10.12
Transverse CT-scan section showing the appropriate rotational placement of the tibial tray
The Effect of Instrumentation for Femoral and Tibial Cuts
For appropriate femoral alignment and component placement intramedullary systems have been shown to offer superior accuracy and are always used [49]. Concerning tibial alignment, there is considerable debate as to whether intramedullary or extramedullary systems provide more accurate reproductions of anatomical axes [50–52]. Extramedullary alignment systems are based on bony landmarks which may be obscured in obese patients or covered by bulky surgical drapes (Fig. 10.13). Therefore most authors suggest that the intramedullary systems are more accurate and reproducible, especially in obese patients and they also reduce surgical time [53–55]. However, in cases with bowed tibia or other extra articular tibial deformities, extramedullary systems are more accurate [56, 57]. The extramedullary tibial guide should be set to engage the ankle 3–6 mm medial of the center, as the center of the talus is medial to the line bisecting the distance between the malleoli [58] (Fig. 10.14). If a intramedullary guide is chosen (Fig. 10.15), the starting hole should be placed approximately one third the distance from anterior to posterior surface of the tibia and slightly medial to the midline [54] (Fig. 10.16). The significance of the entry point on the tibial surface has been emphasized while the deformity of the axis should be taken into consideration [55]. In severe varus knees, it is suggested that the entry point should be placed slightly externally in order to avoid the rod engaging the diaphyseal cortex. Simmons et al. [59] have suggested that the entry point of the rod is an important factor affecting the orientation of the tibial cut and they also found that the 8 mm rod could be inserted in nearly all tibias. In the same study, a 90° tibial component angle was achieved in 83 % of varus knees, but only in 37 % of the valgus knees. The authors conclude that the intramedullary system is less accurate in valgus than in varus knees. Reed et al. [60] has conducted a prospective randomized trial and found that the intramedullary system was more accurate in determining proximal tibial cut in both the coronal and sagittal plane, with a mean deviation of 1.6° only (no outliers were detected). Few other studies have addressed the issue of tibial cut accuracy on the sagittal plane. Most of these have concluded that intramedullary systems can accurately determine tibial cut in the sagittal plane, within 3° or less [16, 52, 61]. However, other authors have found wider deviations and have questioned the accuracy of intramedullary instrumentation [59, 62].
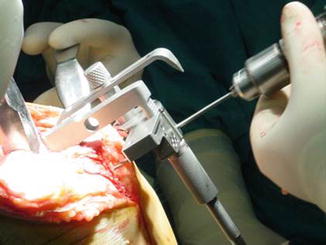
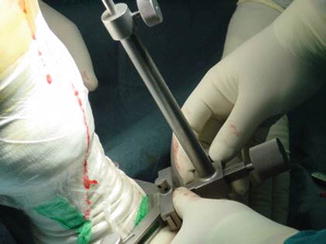
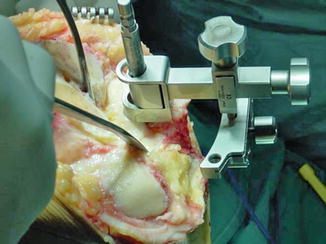
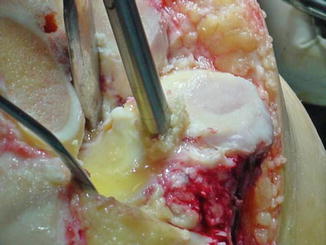
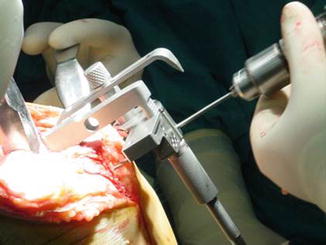
Fig. 10.13
Extramedullary tibial cutting jig is shown
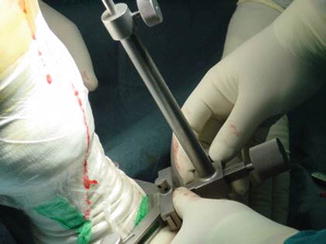
Fig. 10.14
Distal alignment of an extramedullary tibial cutting jig is shown
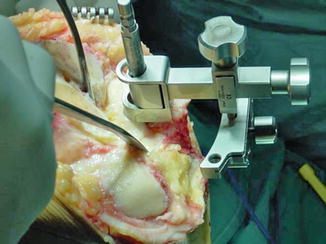
Fig. 10.15
Intramedullary tibial cutting jig is shown
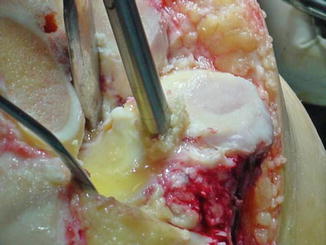
Fig. 10.16
The entrance point of an intramedullary cutting jig is shown
DeKroon et al. [63], in a prospective randomized study of intramedullary versus extramedullary instrumentation, reported better restoration of the posterior tibial slope with the extramedullary system. Finally, in a comparative study between intramedullary and extramedullary guiding systems, the intramedullary system was found to be more accurate for the coronal, while the extramedullary system was more reliable for the sagittal osteotomy, though the mean difference from the planned posterior slope was only 1° [64]. One should keep in mind that when the tibial guide is set to cut at some degrees of posterior slope, it should be set at the center of the tibia on the coronal plane, otherwise the cut will not be set to be horizontal, but in varus (with external rotation of the guide), or valgus (with internal rotation of the guide).
The association of the use of intramedullary systems with systematic effects such as embolism has been questioned (as the insertion of the rod increases medullary canal pressure) [65]. In order to avoid the high elevation of intramedullary pressure, contemporary rods are fluted to allow egress of the medullary material [54]. Transesophageal echocardiography during intramedullary instrumented TKA has demonstrated that a shower of fat or intramedullary embolic particles enter the right atrium of the heart, however, this is not clinically relevant [66]. The peak of the detectable embolization effect occurs shortly after tourniquet release, but no clinical manifestation of fat embolism has been reported and the risk of venous embolism is not increased using intramedullary alignment techniques [67, 68].
The Clinical Relevance of Posterior Tibial Slope
There is great variability of the posterior slope of the native upper tibia in different populations and ethnic groups, often exceeding the conventional figure of 5–10° [69–71]. Tibial posterior slope angle affects flexion gap, tension of the posterior cruciate ligament and knee stability following TKA. Several authors suggest that increased posterior slope after TKA improves maximum knee flexion, but clinical studies have not always confirmed this, as it is well known that flexion achieved after TKA depends on several factors such as quadriceps length, capsular tightness, surgical technique, implant design and rehabilitation. Excessive posterior slope can lead to a slack posterior cruciate, anterior subluxation of the tibia, changes in the loading pattern of the knee and increased polyethylene wear, thus compromising the longevity of the TKA. In contrast, an inadequate posterior tibial slope, or even worse, creation of an anterior slope, is bound to concentrate high stresses on the weak anterior cancellous bone during weight bearing, increasing the possibility of anterior subsidence of the tibial tray. Flexion may also be compromised, due to the tight posterior cruciate and flexion gap [72].
Singh et al. [71] have shown that restoring the preoperative tibial slope to within a range of 2° maximizes the range of movement and flexion angle in posterior stabilized TKA. Bellemans et al. [73], observed an average gain of 1.7° for every degree of extra tibial slope in a cruciate retaining implant. Kim et al. [74] found no significant correlation between postoperative tibial slope and maximum flexion angle in a series of 79 patients, but Shi et al. [75] reported a 1.8° flexion increment with 1° increase of tibial slope in a cohort of 56 patients. Similarly, increased posterior slope was not correlated with increased flexion angle in a comparative study between two groups of patients, one with tibial posterior slope of 5° and another of 0° [76]. An advantageous effect of posterior slope set at 10° compared to neutral is the improvement of the quadriceps lever arm, which might have a positive effect on postoperative mobilization [77]. Seo et al. [78] evaluated clinical outcomes in relation to the posterior tibial slope before and after the operation. Significant improvement was seen in all patients, but it was most notable when the change of the slope was within the range of +3° to −1° [78].
It seems it is important to determine the proper posterior slope angle, taking into consideration the preoperative inclination of the joint surface, but exact limits have not been established. It is recommended, though, that the slope should not exceed 10° [79]. Moreover, using a posterior stabilizing implant and sacrificing the posterior cruciate ligament leads to a slightly bigger flexion gap which, combined with excessive posterior tibial slope, may cause instability. Therefore, while using a cruciate retaining design, a posterior tibial slope of 6–9° is recommended, a maximum of 3° should be achieved in posterior substituting implants in order to avoid instability [80].
The Clinical Relevance of Restoration of Joint Line
Both incorrect femoral and tibial cuts and malpositioning of the components can alter the level of the joint line resulting (if it exceeds 5 mm) in malfunction of the extensor mechanism and anterior knee pain [36, 81]. Impingement of the inferior pole of patella against the tibial insert and impingement of the patellar tendon against the tibial component may occur (Fig. 10.17). Parrington et al. [82] have noted, in a study of 99 revision knee arthroplasties, a statistical difference in clinical scores when the elevation of the joint line was more than 8 mm. Midflexion instability is a relatively new concept, indicating symptomatic medio-lateral laxity in between 30° and 45° of flexion. Joint line elevation has been shown to contribute to the appearance of this form of instability [83, 84]. Removal of excessive bone from the distal part of femur is an important factor responsible for significant elevation of the joint line [85]. An attempt to replace bone loss using a thick polyethylene insert will cause significant elevation of the joint line with subsequent functional effect. Therefore correction of fixed flexion deformity should be attempted by elevation of the posterior capsule from the posterior part of the femoral condyles and not by excessive distal bone removal. During surgery, bony landmarks, like the medial epicondyle or fibular head, are used as guides for joint line calculation but it is not always easy to palpate and clearly see these landmarks. The use of a navigation system has been shown to enable surgeons to restore the joint line with more accuracy than conventional instrumentation systems [86].