Introduction
Arthrofibrosis of the knee is a significant complication after intraarticular injury, including trauma, infection, surgical repair or reconstruction and knee arthroplasty. Arthrofibrosis leads to contracture of the joint, limiting joint mobility and substantially increasing functional impairment and joint pain.
Normal knee function includes movement in a combination of longitudinal axial, flexion/extension, varus/valgus and rotation planes. Full range of motion of the knee can be measured with a goniometer ( Fig. 35.1 ) and typically ranges from 5 degrees of hyperextension to full passive flexion averaging 143 degrees in women and 140 degrees in men. , Motion arcs ranging from 5 degrees of hyperextension to 10 degrees of flexion, also known as the arc of terminal extension , are important for the ‘screw home’ mechanism of knee stabilisation while standing at rest. Flexion to 125 degrees is typically considered sufficient for normal activities of daily living (ADLs) and normal gait, though greater than 125 degrees may be required for full squatting. ,

Knee arthrofibrosis is classically characterised by significant clinical loss of flexion and/or extension of the joint after injury or surgery. , Minor losses of range of motion (ROM) can be detrimental, with more than 10 degrees loss of flexion having a significant effect on running speed. Severe flexion deficits limited to less than 90 degrees can have a significant effect on ADLs, particularly with climbing stairs and sitting. Extension losses are more poorly tolerated than flexion losses, with losses of only 5 degrees resulting in a clinically apparent limp, along with producing significant strain on the quadriceps. ,
In select patient populations such as the elderly, arthrofibrosis significantly increases the risk of falls and pressure ulcers caused by reduction of mobility, contributing to poor long-term outcomes including progressive disability and even death. , Poor long-term outcomes are especially accentuated in this patient population, in whom risk factors for immobility are prevalent. Although advances have been made to prevent arthrofibrosis, motion loss remains a common sequela after intraarticular procedures and joint injuries, with incidences between 4% to 35% depending on types of procedures, injury severity and cause of injury (e.g., anterior cruciate ligament (ACL) reconstruction, total knee arthroplasty).
Pathophysiology
Though there is speculation on the exact cause of arthrofibrosis, several theories have been proposed. In normal tissues there is a baseline level of cytokine signalling that aids in coordination of multiple cellular functions, including growth, differentiation and apoptosis. Cellular signalling may occur through paracrine or autocrine signal regulation. With an injury, inflammation or surgery, inflammatory cytokines induce formation of a platelet clot, along with signalling for migration of neutrophils and macrophages into the site of inflammation. , Subsequently, inflammatory cells and inflamed synovium release platelet-derived growth factor (PDGF) and transforming growth factor-β (TGF-β), promoting fibroblast and myofibroblast activity and deposition of extracellular matrix (ECM). , , ECM deposition promotion by PDGF and TGF-β also leads to inhibition of proteolytic enzymes along with excessive production of collagen, further promoting tissue fibrosis. Aside from their presence in synovial fluid after joint trauma, these cytokines are also found in scar tissue and other fibrotic diseases involving the liver, kidney and lung, suggesting a similar mechanism.
Aside from PDGF and TGF-β, the cytokine vascular endothelial growth factor (VEGF) may also play a role in knee arthrofibrosis. VEGF is an important cytokine involved in multiple biological processes, including (1) vascular regulation, (2) facilitation of vessel permeability, (3) recruitment of inflammatory cells and (4) formation of ECM from fibrinous exudates. , More specifically, VEGF expression is associated with a multitude of inflammatory-induced fibrotic diseases, including rheumatoid arthritis, idiopathic pulmonary fibrosis and peritoneal and pelvic adhesions. In normal conditions in the knee, VEGF is commonly found within the infrapatellar fat pad. On inflammation of the fat pad, whether surgically or pathologically induced, there is subsequent release of VEGF, resulting in vascular proliferation and fibrosis. , Trauma and intraarticular haemorrhage associated with surgery can also lead to tissue fibrosis as a result of release of VEGF and other fibrogenic factors, with secondary maturation of intraarticular clots into fibrotic tissue. ,
Perhaps most importantly, immobilisation after injury or surgical procedures results in reduced connective tissue elasticity, whereas decreased mechanical stress within the joint leads to upregulation of ECM and proliferation of degradative enzymes. , This results in less elastic tissue and formation of acute fibrotic adhesions. , Over time, the connective tissue progresses to chronic fibrosis, with peak fibrosis observed after 8 weeks. , Thus development of arthrofibrosis is multifactorial, with inflammation and immobilisation both playing significant roles.
Risk Factors
Several risk factors have been described that may increase the risk or incidence of arthrofibrosis. These include traumatic injury to the knee, surgical treatment of pathologic conditions of the knee, graft choice/tensioning and tunnel placement during knee ligament reconstruction, timing of surgery after injury and genetics.
After traumatic injury to bone or soft tissue, local inflammatory responses have been shown to increase the risk of fibrosis and subsequent motion loss. In cases of trauma, including acute ligament tears such as ACL ruptures, knee dislocations or motor vehicle accidents, severe or multiple traumatic insults can have an impact on a multitude of factors, including disrupted wound healing, delayed joint mobility and subsequent arthrofibrosis. The incidence of arthrofibrosis in isolated ACL reconstruction has been reported between 4% to 28%, and this risk increases with increased trauma or damage to the knee. , In patients with multiligamentous injuries involving the ACL and MCL, Harner et al. reported that associated medial collateral ligament (MCL) repair in patients undergoing ACL reconstruction could lead to extensive disruption of the medial capsule and an elevated fibrogenic response, with an increased risk of arthrofibrosis. In general, a greater number of extraarticular procedures around the knee will result in an increased risk of arthrofibrosis. Traumatic knee dislocation, which produces variable patterns of ligamentous instability, results in the highest incidence of motion loss. Sisto and Warren reported motion problems in 6 of 20 patients (30%), whereas Shapiro and Freedman reported an even higher 57% incidence of postdislocation motion loss in this patient group.
With regards to concomitant meniscal pathologic conditions with ACL tears, Nwachukwu et al. reported that female sex, joint immobilisation and meniscal repair in ACL reconstruction were related to increased risk of fibrosis development. In contrast, Cosgarea et al. found that there was no increased risk of arthrofibrosis in ACL reconstruction with either meniscal repair or a partial meniscectomy, although advancements of ACL reconstruction and other arthroscopic procedures may account for the discrepancy in findings. Further postoperative complications, including postsurgical infection and complex regional pain syndrome (CRPS), have also been identified as independent risk factors for arthrofibrosis.
Several technical aspects of ACL reconstruction have been studied to determine their respective roles as risk factors for arthrofibrosis, including (1) graft choice, (2) tunnel placement and (3) graft tensioning techniques. First, with regards to graft choice, Pinczewski et al. investigated differences in 5-year incidences of arthrofibrosis in patients receiving either patellar tendon or hamstring autografts for ACL reconstruction. The incidence of arthrofibrosis (described as extension deficits) was reported as 31% in the patellar tendon cohort and 19% in the hamstring cohort, though these results were not statistically significant. Sajovic et al. also assessed for differences in ROM in a prospective, randomised trial comparing the use of either patellar tendon or hamstring allografts, and found no significant difference between the two groups. Thus graft choice is not considered a potential risk for fibrosis and/or motion loss. , ,
Second, in contrast to graft choice, improper graft placement during ACL reconstruction has been established as a significant risk factor for motion loss. Graft placement in a location anterior to the native ACL insertion point on the tibial side can lead to significant loss of ROM as a result of impingement of the graft on the roof of the notch in full extension and should be accounted for during surgery. , On the femoral side the most common error is graft placement too far anterior, which causes excessive strain on the graft, leading to limited flexion and potential graft failure. ,
Third, ACL graft tensioning techniques have been a source of debate as to their effect on postoperative stiffness and subsequent arthrofibrosis. Many surgeons elect to tension the ACL at 30 degrees of flexion with a posterior drawer force applied to the knee. Interestingly, evidence now demonstrates that the ACL lengthens 1 to 3 mm in the terminal 30 degrees of extension, and biomechanical data suggest that fixation at 30 degrees with a posterior drawer force increases the risk of overconstraint of the ACL graft. Additional studies of anatomical intraarticular ACL reconstructions in cadaver knees have confirmed these findings, demonstrating that tensioning at 30 degrees of flexion overconstrains the knee regardless of the amount of force used during tensioning. Therefore most authors now recommend tensioning and securing the graft in full extension rather than 30 degrees of flexion.
Timing for surgery after intraarticular injury has mixed results with regards to the association with arthrofibrosis. Shelbourne et al. conducted a study of 169 young athletes undergoing acute ACL reconstruction and assessed for the incidence of fibrosis based on the timing of repair. An increased risk of fibrosis was observed in those undergoing repair within 1 week of injury compared with those where surgery was postponed more than 21 days. This coincides with findings of Harner et al., where arthrofibrosis was observed in 27% of patients who underwent acute ACL reconstruction compared with 5% after chronic reconstruction. Further studies reported similar results, where postponing reconstruction for at least 4 weeks after injury was associated with decreased rates of fibrosis compared with those undergoing earlier treatment, , although no differences in the rates of arthrofibrosis were identified after 4 to 6 weeks of delay. , , Conversely, Sterett et al. found no association between the timing of surgery and the incidence of fibrosis. Potential risks of early surgical intervention should be considered in preparation of intraarticular repair or reconstruction, and the authors generally recommend a delayed approach to surgical fixation of most traumatic intraarticular injuries, most notably in those undergoing ligament reconstruction.
Finally, genetics may play a role in arthrofibrosis. A twin concordance study by Hakim et al. demonstrated that there was a significant hereditary association in development of shoulder arthrofibrosis. Further, a study conducted by Skutek et al. analysed blood samples and the DNA of 17 patients with arthrofibrosis after autograft ACL reconstruction for human leucocyte antigens (HLA). , Compared with the control group, patients with arthrofibrosis were more likely to have the HLA-Cw∗08 allele and less likely to have the HLA-Cw∗07 allele. , Considering the small sample size, further studies are required to determine the significance of these alleles and if there is increased risk for arthrofibrosis in these populations, whether because of a decreased inflammatory defence or increased fibrotic susceptibility. ,
Prevention of Arthrofibrosis
Prevention of arthrofibrosis is typically the best treatment for this pathologic process and can be implemented with multiple approaches after injury and surgical procedures. An important first step in prevention of arthrofibrosis includes management and/or prevention of immobility. Studies conducted with animal models demonstrated that joint contractures developing within the first 2 weeks of immobilisation are associated with muscular limitations and can be resolved with remobilisation of the joint. , , If remobilisation is not restored within 4 weeks, the contractures may become irreversible as a result of involvement of connective tissues and fibrosis. , , The time frame associated with these pathophysiologic alterations in joint contractures highlights the importance of implementing protocols for early mobilisation.
A prospective study conducted by Noyes et al. investigated the rates of arthrofibrosis in patients after ACL reconstruction alone or concomitantly with other procedures (e.g., meniscal repair) if an early program for joint mobilisation was implemented. The study of 443 knees found that 436 (98%) had normal ROM at follow-up, with only minor losses of extension found in the remaining knees. There were no cases of permanent arthrofibrosis in the cohort. Thus early mobilisation plays a vital role in prevention of fibrosis. Coincidentally, elderly populations are at increased risk of joint arthrofibrosis, particularly as a result of nonmodifiable factors, including progressive vision loss, dementia and previous lower extremity injuries, or other theoretically modifiable factors, including depression, fear of falling and excessive use of restraints or sedation in care facilities resulting in prolonged immobilisation. , , If present, management of these risk factors can also aid in prevention of fibrosis.
Extracorporeal shockwave therapy (ESWT), a form of noninvasive therapy used for various tendinitis and fibrotic disorders, has been investigated as a possible preventative mechanism of arthrofibrosis after intraarticular procedures. A 2019 study by Zhou et al. examined the effectiveness of ESWT therapy on prevention of extensive arthrofibrosis in rabbits with induced intraarticular adhesions after femoral cortical shaving and cast immobilisation. The experimental group was exposed to shockwave therapy 5 days per week for 4 total weeks, whereas no shockwave therapy was administered to the control group. At 4 weeks the control group had significantly higher levels of arthrofibrosis and a greater contracture angle compared with the experimental group. Although its use is known as a treatment method after various fibrotic disorders develop (e.g., capsular contracture, Dupuytren contracture), , further evidence is necessary to determine its use in prevention of arthrofibrosis.
Pharmacological measures have also been studied for prevention of arthrofibrosis, most notably bevacizumab and losartan. Bevacizumab, a recombinant humanised monoclonal IgG1 antibody, functions by targeting VEGF, a cytokine previously described to play vital roles in angiogenesis and fibrogenesis. , In vitro models of collagen gel assays demonstrated that bevacizumab can significantly inhibit gel contraction ability, along with inducing fibroblast death. , In vivo assays by Emami et al. using rabbit models demonstrated that intraarticular injection of bevacizumab in the experimental group significantly prevented loss of ROM along with decreasing levels of fibroblasts, vascularity and ECM deposition compared with the control. Aside from inhibition of VEGF, bevacizumab also plays a role in reducing expression of TGF-β, another fibrogenic cytokine, posing as a beneficial therapy for overall fibrotic inhibition.
Losartan, an angiotensin II type 1 receptor blocker (ARB), is a medication commonly used as an antihypertensive agent, commonly in patients with chronic disease of several organ systems including the heart, lungs, kidneys and liver. Interestingly, another substantial benefit of this drug is related to the prevention of fibrotic remodelling of these organ systems secondary to elevated levels of angiotensin II. Particularly of interest to orthopaedic surgery, several studies using animal models have also demonstrated the benefit of losartan in musculoskeletal healing postinjury. Two separate animal studies conducted by Kobayashi et al. , concluded that early administration of losartan significantly improved myofibre healing and reduced fibrosis formation after muscular injury. In addition, a randomised and blinded experimental study by Baranowski et al. demonstrated a significant decrease in joint myofibroblast levels after administration of losartan to rats with joint injury and immobilisation; however, no significant difference in ROM was observed between the losartan or placebo groups after joint recovery. The antifibrogenic effects of losartan are theorised to be due alteration of the TGF-β pathway secondary to angiotensin receptor blockade, blunting the proliferation of fibroblasts and myofibroblast. , Thus losartan poses as a promising agent for arthrofibrosis prevention.
Furthermore, there are several other pharmacologic or procedural approaches that may be useful preemptive therapies. Antiinflammatory drugs, including nonsteroidal antiinflammatory drugs (NSAIDs), can reduce inflammation and subsequent fibrosis. Intraarticular agents, including hyaluronan, chitosan, botulinum toxin, mitomycin C and proteoglycan decorin, have all shown efficacy in reducing fibrotic adhesion formation/ progression. Additionally, the use of hyaluronic and amniotic membranes as a barrier effect to fibrosis was also demonstrated in animal models. Though these methods are promising, further analyses are necessary to fully understand their efficacy.
Clinical Presentation
Clinically a thorough examination should be performed to evaluate for full ROM of the knee. As noted, arthrofibrosis classically involves a loss of flexion, extension or both. In a normal knee, flexion and extension are typically categorised into three subarcs: terminal extension, active function and passive flexion. The arc of terminal extension begins at the limit of passive extension. This arc moves from 10 degrees of flexion to 5 degrees of hyperextension and is rarely used in normal gait but is thought to allow for quadriceps muscle relaxation during the stance phase. The arc of active function ranges from 10 degrees to approximately 120 degrees, which covers the range needed for most ADLs. The arc of passive flexion begins at approximately 120 degrees and continues to the passive limit of an applied external force, to approximately 140 degrees.
The functional effects of knee motion loss vary depending on patient activity. In general, flexion to at least 125 degrees is adequate for most patients to complete ADLs and usually does not adversely affect normal gait. Flexion less than 125 degrees, however, may inhibit the patient’s ability to squat or perform deep flexion activities. In athletes, loss of flexion of 10 degrees or more can affect running speed, whereas severe flexion deficits less than 90 degrees affect the ability of even the most sedentary patient to sit or climb stairs.
In contrast to flexion loss, even a small loss of extension is poorly tolerated and can be difficult to manage. As little as 5 degrees of extension loss can produce a noticeable limp during ambulation, strain the quadriceps muscle and contribute to patellofemoral pain. During weightbearing on a flexed knee, the quadriceps muscle force required to stabilise the knee is 75% of the load on the femoral head at 15 degrees of flexion, 210% at 30 degrees and 410% at 60 degrees. With increased joint contact pressure, the clinical consequences are increased quadriceps muscle activity and fatigue and, ultimately, patellofemoral arthrosis.
On physical examination a patient presenting with knee joint arthrofibrosis may demonstrate diffuse oedema, warmth, pain, limitation of patellar mobility and limitation of both extension and flexion. A late presentation of arthrofibrosis may result in patella infera. Proper treatment is dependent on accurate diagnosis of motion loss. The most common and reproducible diagnostic method involves the use of a goniometer over the lateral knee joint line in the midsagittal position, using the greater trochanter and lateral malleolus as reference points (see Fig. 35.1 ). A second method involves measuring the heel-height difference, which is performed with the patient in the prone position. In general, 1 cm of heel-height difference correlates to 1 degree of knee flexion contracture. This technique may be helpful in detecting subtle degrees of motion loss (<10 degrees).
The classification of motion loss is somewhat arbitrary, but attempts have been made to develop formal classification systems for arthrofibrosis. For example, Del Pizzo et al. graded motion loss by evaluating deviation from full flexion and extension, with severe motion loss considered to be more than 10 degrees from full extension and less than 90 degrees of flexion ( Table 35.2 ). Blauth and Jaeger graded motion loss based on full arc of motion as grade I (mild, ROM >120 degrees), grade II (moderate, ROM 80 to 120 degrees), grade III (severe, ROM 40 to 80 degrees) and grade IV (extreme, ROM <40 degrees). Another classification system was introduced in 1996 by Shelbourne et al., who compared motion loss on the affected side with the normal contralateral limb. The authors identified four types: (1) normal flexion with extension loss less than 10 degrees; (2) normal flexion with extension loss greater than 10 degrees; (3) flexion loss greater than 25 degrees and extension greater than 10 degrees; and (4) flexion loss greater than 30 degrees and extension loss less than 10 degrees with patella infera ( Table 35.1 ).
Extension Loss | Flexion |
---|---|
Posterior capsular contractures | Suprapatellar adhesions |
Anterior interval scarring | Patella baja/infera |
Intercondylar notch fibroproliferative cyclops lesions | |
ACL/PCL contracture or malpositioning |
Del Pizzo ET AL. | Blauth & Jaeger | Shelbourne ET AL. | |||
---|---|---|---|---|---|
Severity | Extension Loss | Flexion | Full-Arc ROM | Extension Loss | Flexion Loss |
Mild/type 1 a | <5 degrees | >110 degrees | >120 degrees | <10 degrees | None |
Moderate/type 2 | 5–10 degrees | 90–110 degrees | 80–120 degrees | >10 degrees | None |
Severe/type 3 | >10 degrees | <90 degrees | 40–80 degrees | >20 degrees | >25 degrees |
Extreme/type 4 | <40 degrees | >10 degrees | >30 degrees b |
a Motion loss grades of mild to extreme were used by Del Pizzo et al. and Blauth et al., whereas Shelbourne et al. categorised by type.
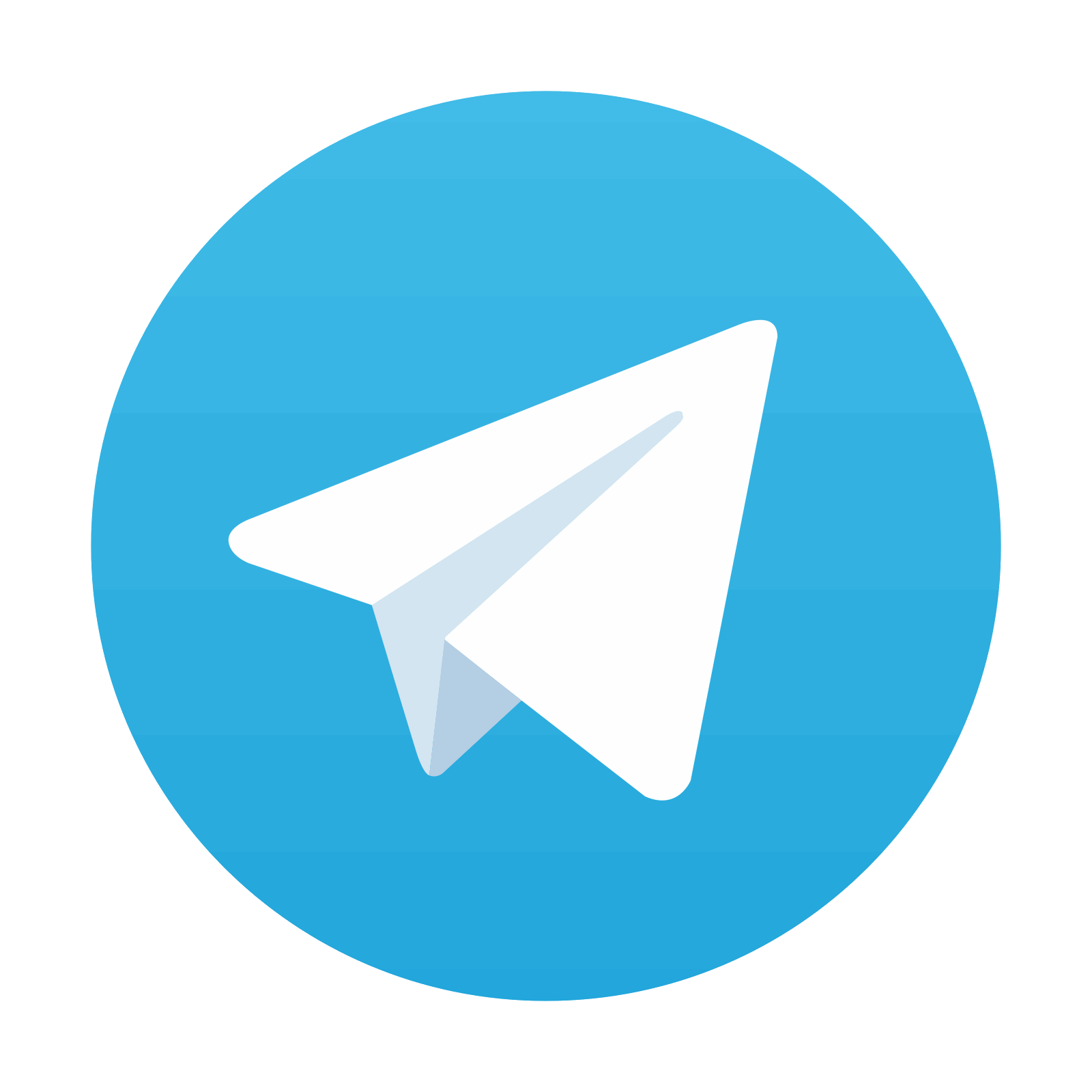
Stay updated, free articles. Join our Telegram channel

Full access? Get Clinical Tree
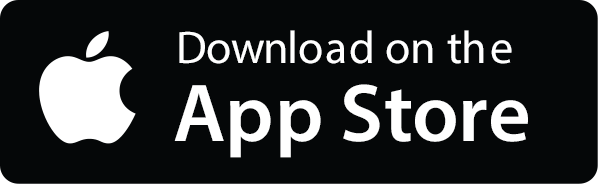
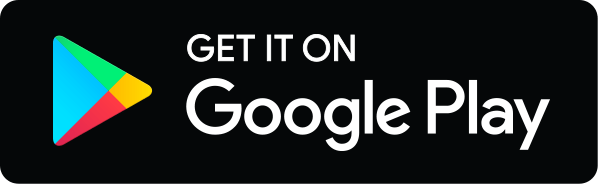
