Knee Anatomy
Wolfgang Fitz, MD
Jeffrey Lange, MD
Dr. Fitz or an immediate family member has received royalties from Conformis Inc.; serves as a paid consultant to or is an employee of Conformis Inc.; has stock or stock options held in Conformis Inc.; and has received research or institutional support from IGB. Neither Dr. Lange nor any immediate family member has received anything of value from or has stock or stock options held in a commercial company or institution related directly or indirectly to the subject of this chapter.
ABSTRACT
The anatomy of the knee has been described in depth, with the addition of the newly recognized anterolateral ligament. Knee kinematics is discussed, clarifying aspects of modern dynamic motion analysis. Advancements in knee imaging focus on simplifying routine radiographs by implementing fixed flexion series and introducing weight-bearing MRI, which is not currently available for routine clinical use. Future imaging improvements may assist in the diagnosis of unstable meniscal injuries and painful activities of the patellofemoral joint. Better three-dimensional imaging techniques may replace stress views for a more accurate assessment of all three parts of the knee joint when planning surgical treatment, such as high tibial osteotomies or unicompartmental knee arthroplasties.
Keywords: anatomy; knee kinematics and imaging; physical examination
Introduction
The human knee is composed of three articular compartments surrounded by a robust soft-tissue envelope, including synovium, joint capsule, muscle, tendon, bursa, subcutaneous tissue, and skin. The complex dynamic relationship between these structures confers the knee’s function as a major weight-bearing joint of the body, allowing for great flexibility, pivoting, and high-impact activities. The anatomy of the human knee could be a topic of an entire textbook in and of itself. We offer a brief overview below as a basis for understanding the clinically and orthopaedically relevant anatomy of the human knee.
General Knee Anatomy
Bone
The knee consists of articulations among three bones: the tibia, the femur, and the patella. The tibiofemoral articulation has two compartments, medial and lateral. Each of these two compartments houses the interaction between its respective tibial and femoral condyles. The patellofemoral compartment houses the interaction between the patella and the femur’s trochlear groove. In each articular compartment, the bone serves as a weight-bearing surface and scaffold for the overlying articular cartilage. Each articulation and the relevant bony anatomy are reviewed in the “Knee kinematics” section below.
Articular Cartilage
All articular surfaces of the knee joint consist of an articular cartilage cap that is supported by underlying bone. Articular cartilage is highly resistant to wear and allows for low-friction gliding motions under normal knee conditions.1 Articular cartilage is composed of chondrocytes and extracellular matrix. Extracellular matrix is largely composed of water, proteoglycans, and collagen (predominantly type II), with much smaller amounts of noncollagenous proteins and glycoproteins.2 Articular cartilage is avascular and receives its nutrition by diffusion through the extracellular matrix. It is a highly organized structure, consisting of four major zones: superficial zone, middle (transitional) zone, deep zone, and calcified zone (Figure 1). Each zone performs a unique function in the development and maintenance of the articular cartilage layer.
Synovium
The knee joint cavity is lined by tissue called synovium. The synovium has two layers—the intima, adjacent to the knee joint itself, and the subintima, which adheres to the joint capsule just superficial to it. The synovium
contributes to the production of synovial fluid, which provides joint lubrication and diffusion of nutrients to the articular cartilage. The intimal layer contains two types of specialized cells, or synoviocytes, that are responsible for production and maintenance of synovial fluid. Type A cells are macrophage-like cells that clear the joint of excess material or pathogens. Type B cells produce vital components of synovial fluid. The subintimal layer contains numerous cell types and acts as a conduit to the overlying joint capsule. This layer exhibits a rich vascular and nerve supply, which may be related to its function in both joint nutrition and biomechanical optimization.3,4
contributes to the production of synovial fluid, which provides joint lubrication and diffusion of nutrients to the articular cartilage. The intimal layer contains two types of specialized cells, or synoviocytes, that are responsible for production and maintenance of synovial fluid. Type A cells are macrophage-like cells that clear the joint of excess material or pathogens. Type B cells produce vital components of synovial fluid. The subintimal layer contains numerous cell types and acts as a conduit to the overlying joint capsule. This layer exhibits a rich vascular and nerve supply, which may be related to its function in both joint nutrition and biomechanical optimization.3,4
Meniscus
The knee has two menisci—medial and lateral. Each is composed of multiple collagen types and extracellular matrix.5 The meniscus functions as both a load distributor during weight bearing and as a secondary stabilizer of the knee joint throughout range of motion. The medial meniscus is bound more tightly to the joint capsule via the deep medial collateral ligament (MCL) and the meniscotibial and meniscofemoral ligaments. The medial meniscus demonstrates relatively little excursion during range of motion, while the lateral meniscus demonstrates relatively great excursion during range of motion. Each has anterior and posterior roots tethered to the anterior and posterior tibia, respectively. The posterior root of the lateral meniscus attaches to the posteromedial femoral condyle via the anterior and posterior meniscofemoral ligaments, otherwise known as the ligaments of Humphrey (anterior to posterior cruciate ligament) and Wrisberg (posterior to posterior cruciate ligament).5 The properties of the menisci contribute to the knee’s effective pivot on the medial side, as the medial femoral condyle remains relatively centered on the tibial plateau, while the lateral femoral condyle moves about more freely on the lateral tibial plateau during range of motion (see “Knee kinematics” section below).
Ligaments
There are four major ligaments of the knee and multiple lesser ligaments. The four major ligaments are the anterior cruciate ligament (ACL), the posterior cruciate ligament (PCL), the medial collateral ligament (MCL), and the lateral collateral ligament (LCL). The ACL is an intra-articular structure, finding its attachments on the tibial eminence anteriorly, as well as the lateral aspect of the femoral notch of the lateral femoral condyle. The PCL finds its attachments on the medial aspect of the femoral notch and the midportion of the posterior tibial plateau. The superficial portion of the MCL originates at the medial femoral epicondyle and inserts on the proximal medial tibia, arising deep to the quadriceps’ aponeurosis and superficial to the knee’s joint capsule. The deep portion of the MCL, located in the midsagittal plane, is a confluence of the meniscofemoral ligament extensions, meniscotibial ligament extensions, and the knee capsule on the medial side. The LCL originates at the lateral femoral epicondyle and inserts on the fibular head.6,7
Recently, the anterolateral ligament (ALL) has been recognized as a distinct structure separate to the LCL.8 Originally described by Segond in 1879 as a “pearly, resistant, fibrous band,” the ALL has not been fully recognized as a distinct structure until recently.8 In their cadaveric dissection analysis, Claes et al. identified the ALL as a distinct structure in 97% of specimens.8 Brockmeyer et al corroborated these findings in a separate dissection analysis of 23 knees, identifying a distinct ALL structure in 100% of specimens.9 The authors describe a reproducible course of the ALL from its femoral insertion overlapping the LCL slightly proximal and posterior to the lateral femoral epicondyle, and coursing distal and anterior, inserting on the tibia between Gerdy tubercle and the fibular head.9 Others have noted similar findings after dissection in separate cadaveric cohorts.10,11
There are several other ligaments that surround the knee joint and contribute to its stability throughout range of motion. These ligaments can be visualized in Figure 2.
Muscles and Tendons
The quadriceps tendon is the confluence of four major tendons originating from four distinct muscles—the vastus medialis, vastus lateralis, vastus intermedius, and rectus femoris—and inserts onto or around the superior portion of the patella, creating the midportion of the superior arm of the extensor mechanism. Inferiorly, the patellar tendon originates at the inferior pole of the patella and attaches to the tibial tubercle. In addition, medial and lateral sleeves of tissue invest the patella and contribute to the function of the extensor mechanism. These tissue sleeves represent a confluence of the three layers of the knee. Most superficially is the arciform layer; deep to that layer is the retinacular layer, including the patellofemoral and patellotibial ligaments; and deep to that layer is the joint capsule.6
The hamstring muscles include the semimembranosis, semitendinosis, biceps femoris, and gracilis. These muscles course along the posterior aspect of the thigh. The long and short heads of the biceps femoris insert in part on the fibular head, among a myriad of other lateral structures.6 The remaining hamstring tendons insert on the tibia medially. The semimembranosis tendon has a broad attachment that invests a large portion of the posteromedial tibia. The semitendinosis, gracilis, and sartorius tendons course toward an anteromedial insertion on the tibia and lie beneath the pes anserine bursa.
The popliteus muscle originates from the posterior aspect of the tibia and inserts on the lateral aspect of the femur. The popliteus tendon is unique as it courses through the knee joint itself, between the posterolateral knee capsule and the lateral meniscus, creating the “popliteus hiatus.” The popliteofibular ligament arises from the musculotendinous junction of the popliteus and inserts on the fibular head.
The medial and lateral heads of the gastrocnemius muscle insert on the medial and lateral posterior femoral condyles, respectively, via their tendinous extensions. The gastrocnemius muscle contributes to active knee flexion and can contribute to knee flexion contracture when abnormally tight.
Blood Supply
The human knee has a rich collateral blood supply. The femoral artery courses posterior to the thigh above the knee. As the femoral artery reaches the level of the knee
and becomes the popliteus artery, it gives rise to the superolateral and the superomedial geniculate arteries. As the popliteus artery courses distally, it gives rise to the middle geniculate artery and then, further distally, the inferomedial and inferolateral geniculate arteries. Each of the four medial or lateral geniculate arteries course circumferentially around the knee from posterior to anterior and ultimately form the anterior patellar anastamosis. In addition, further anastamoses are created by branches arising from the lateral circumflex femoral artery, the femoral artery, and the more distal tibial arteries.12
and becomes the popliteus artery, it gives rise to the superolateral and the superomedial geniculate arteries. As the popliteus artery courses distally, it gives rise to the middle geniculate artery and then, further distally, the inferomedial and inferolateral geniculate arteries. Each of the four medial or lateral geniculate arteries course circumferentially around the knee from posterior to anterior and ultimately form the anterior patellar anastamosis. In addition, further anastamoses are created by branches arising from the lateral circumflex femoral artery, the femoral artery, and the more distal tibial arteries.12
Knee Kinematics
The anatomy and function of the knee have fascinated anatomists and surgeons for over 160 years.13 Normal knee kinematics has been historically defined by describing the movement of both femoral condyles relative to anatomically defined axes involving the femur, tibia, and patella. Multiple axes have been described, and no single set of axes has been used as a benchmark.14 Many studies have defined axes by looking at images of various flexion angles under static conditions. This method has been described as a “stop-motion technique.” With the introduction of fluoroscopy and three-dimensional (3D) image matching, this technique has evolved from initially using 3D templates of total knee replacements to 3D models of segmented individual bone structures utilizing surface contact points of the anatomic structures.15,16 These methods have been derived from CT or MRI to describe not only the kinematics of total knee arthroplasty, but also that of normal knees. Lately, static motion analysis of the normal knee has moved to in vivo dynamic motion analysis with the help of CT. The CT of the knee is performed with a high-resolution (1 mm intervals) technique and a 3D model of the femur and tibia are individually generated. Modeling software is utilized with a specifically described anatomic coordinate system (21) by referencing bony landmarks in 3D. Tnaifuji et al17 analyzed in vivo knee kinematics of the normal knee through full range of motion via the 3D-to-2D registration technique including the geometric femoral condylar axis (GCA) to compare findings with prior published stop-motion techniques on healthy young volunteers without mechanical systems or history of knee injury. They confirmed the findings of prior publications that the normal knee during range of motion results in tibia internal rotation with flexion relative to the GCA and both condyles moved posteriorly on the tibia above 120° flexion, representing the so-called bicondylar roll back motion.
They also noted that 25% of their patients experienced a lateral pivot motion going from full extension to flexion because of anterior translation of the medial femoral condyle during this motion. This echoed others’ previous findings and represents the “screw home mechanism.”18 The screw home mechanism occurs as follows. In full extension, the knee slightly hyperextends with slight tibial external rotation while collateral and cruciate ligaments are tightened to lock the knee in extension to decrease muscle forces while standing.19 In this position, the lateral femoral condyle sits slightly more anterior than the medial femoral condyle on the tibial plateau. The knee is unlocked with the popliteus muscle initiating flexion by pulling the lateral femoral condyle backward while the medial femoral condyle slides forward resulting in tibial internal rotation.20 This initiates flexion, leaving the medial condyle more or less central on the medial tibial plateau while the lateral condyle rolls back, explaining the medial pivot kinematic pattern.
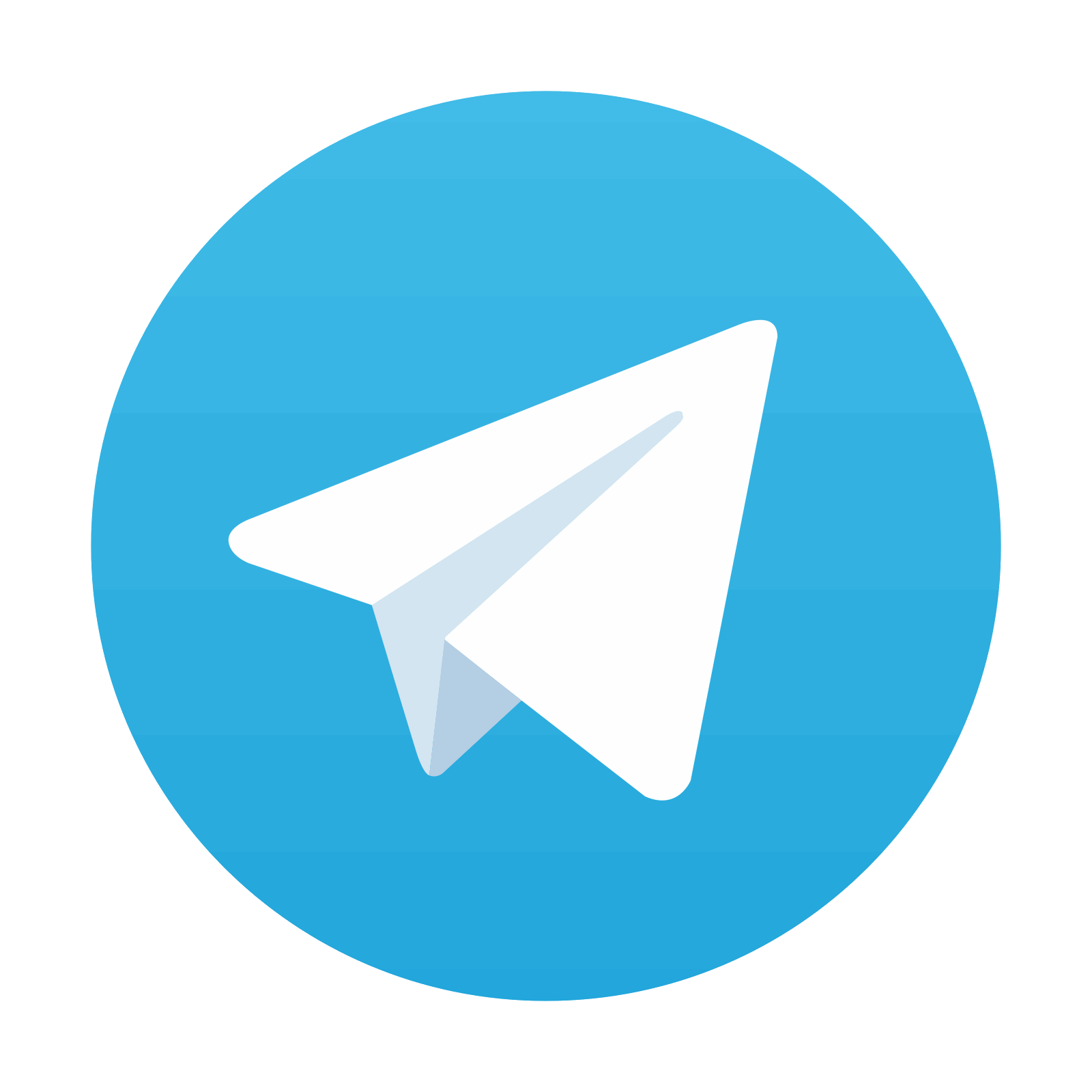
Stay updated, free articles. Join our Telegram channel

Full access? Get Clinical Tree
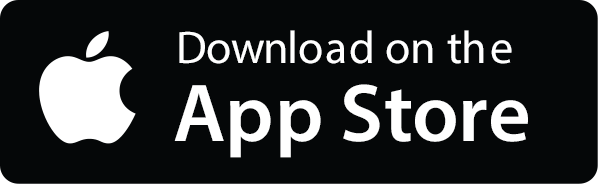
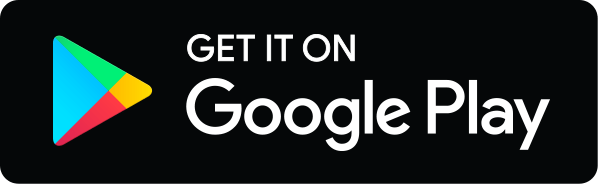