Fig. 16.1
Mechanistic model of skeletal muscle function. CC, SEC, and PEC represent contractile component, series elastic component, and parallel elastic component, respectively
It has been confirmed that tendon length changes as a function of the force applied to it (animals: Ker 1981; Woo et al. 1980—humans, in vivo: Fukashiro et al. 1995; Ito et al. 1998) (Fig. 16.2). Accordingly, it is generally accepted that tendons have elastic properties and play a significant role in storing and utilizing elastic energy (Magnusson et al. 2008). When researchers first studied SSC, the length changes in muscle fibers were not distinguished from those in MTUs (Cavagna 1977). However, because little change was noted for muscle fiber length in running cats (Hoffer et al. 1989), researchers started to distinguish the length change between MTUs and muscle fibers. Research involving ultrasonography revealed that stretch–shortening in the tendons accounts for that of the entire MTUs, and that length change of the fascicles was smaller than that of the MTUs (muscle–tendon interaction) during human movements such as walking (Fukunaga et al. 2001; Lichtwark et al. 2007; Ishikawa et al. 2005), running (Ishikawa et al. 2007; Lichtwark et al. 2007), and jumping (Kawakami et al. 2002; Kurokawa et al. 2003; Sousa et al. 2007; Ishikawa and Komi 2004) (Fig. 16.3).
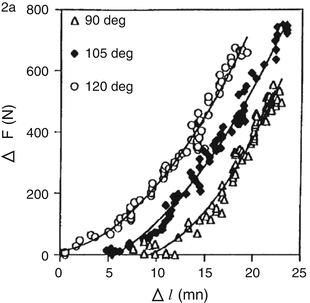
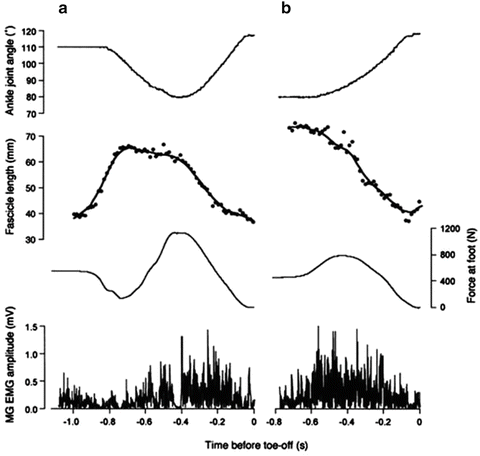
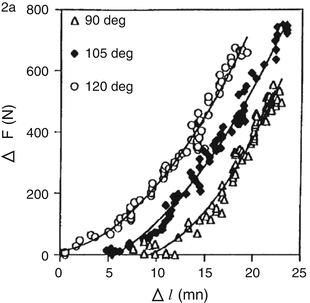
Fig. 16.2
Force–length relationship of the human tendon (the tibialis anterior muscle) in the 3 angles that were measured for the ankle joint (Fukashiro et al. 1995)
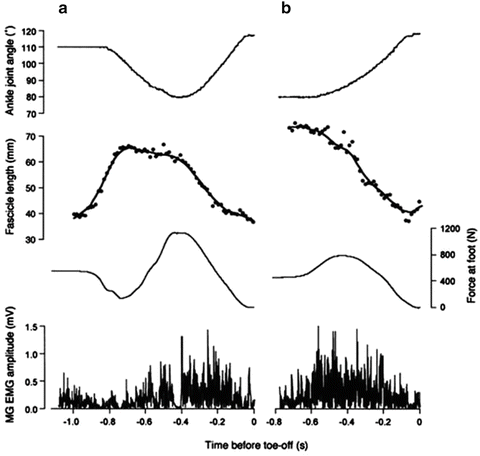
Fig. 16.3
Ankle joint angle, fascicle length of medial gastrocnemius muscle, reaction force at the foot perpendicular to the force plate, and EMGs from the medial gastrocnemius muscle during CMJ (A) and noCMJ (B). Both CMJ (A) and noCMJ (B) were performed with only the ankle joint. In CMJ (A), fascicle length started to increase (passive lengthening) then remained constant for most of the dorsiflexion phase, then began to decrease in the plantar flexion phase. In noCMJ (B), the fascicle length kept decreasing throughout the movement (Kawakami et al. 2002)
16.3 Mechanisms of the Countermovement Effect
The following concepts have been proposed as mechanisms for the increase in mechanical output produced by the SSC as compared with concentric contractions where SSC is not present.
1.
Stretch reflex
2.
Potentiation of contractile component
3.
Time available for active state development (preactivation)
4.
Utilization of elastic energy
5.
Muscle–tendon interaction
The stretch reflex is a muscle contraction in response to stretching; when muscle fibers are rapidly lengthened, the muscle spindle detects the lengthening and increases alpha motor neuron activity, resulting in muscle fiber contraction (Jones and Watt 1971). Potentiation of the contractile component is force potentiation derived from alteration in the properties of the contractile machinery during eccentric contraction (Bosco et al. 1981; Herzog and Leonard 2000). During the braking phase (last half of the countermovement), muscles develop an active state if time is available; this results in a higher active state of the muscle at the beginning of the main movement (Bobbert et al. 1996; Bobbert and Casius 2005; Chapman et al. 1985; Svantesson et al. 1994; Arakawa et al. 2010). Utilization of elastic energy is implemented by a series of elastic components (Komi and Bosco 1978; Komi 2003; Arakawa et al. 2010). Muscle–tendon interaction creates a favorable situation for muscle fibers to exert force by decreasing muscle fiber length change (which results in decreased contraction speed and adjusts the sarcomere length to optimal) by utilizing the elastic behavior of the tendon (Kawakami and Fukunaga 2006).
Kawakami et al. (2002) reported that although gastrocnemius fascicles were not lengthened during the braking phase of the countermovement jump (CMJ), which is performed with the ankle joint alone, the mechanical output (e.g., mechanical power and work) was increased compared with jumping without countermovement (noCMJ) (Fig. 16.3). On the basis of this result, Kawakami and Fukunaga (2006) pointed out that there are cases where the countermovement effect can be achieved without the stretch reflex and potentiation of contractile components that occur during forceful lengthening of the muscle fibers. Although Sugisaki et al. (2005) observed a slight fascicle lengthening during the braking phase of a drop jump, they also indicated that most of mechanical work of MTUs was assumed by tendons. In addition, they reported that the mean amplitude of electromyographic activity (mEMG) of the triceps surae muscle during a drop jump did not exceed that during noCMJ. According to these findings, it would be reasonable to suggest that just muscle–tendon interactions and the corresponding utilization of elastic energy, as well as the time available for active state development, play significant roles as mechanisms underlying the countermovement effect (at least for the gastrocnemius muscle).
Through simulation studies of vertical jumps that involved the analysis of the 3 major joints (hip, knee, and ankle) of the lower extremity, Bobbert and Casius (2005) reached the conclusion that the time available for active state development of the contractile component is the only mechanism underlying the countermovement effect. Conversely, Arakawa et al. (2010) conducted a simulation study on a single MTU and pointed out that both active state development and elastic energy contribute to the countermovement effect and that the degree of contribution of these factors varies with length of the series elastic component (a longer series elastic component makes the elastic energy contribution higher). Bobbert and Casius (2005) utilized a model involving the lower extremity that included muscles with relatively short tendons, such as the gluteus maximus muscle. This likely explains why their results indicated that elastic energy did not contribute to the countermovement effect. In addition, the degree of utilization of elastic energy is affected by the intensity of the countermovement (Sugisaki et al. 2004; Ishikawa and Komi 2004). With regard to CMJ from a standing position, elastic energy may not play a significant role for the following reasons: (1) The fall length of the center of gravity was relatively short, resulting in a lower intensity of countermovement (than drop jumps). (2) The stretching load was distributed over the MTUs of the lower extremity. When combined, the contribution of each mechanism of the countermovement effect varies with situations such as tendon length of the intended MTUs and/or intensity of the countermovement.
16.4 Factors Related to Interindividual Differences in the Countermovement Effect
Because mechanisms involved with the countermovement effect vary according to the particular situation (i.e., characteristics of recruited MTUs and/or intensity of countermovement), it is difficult to summarize the factors that relate to the interindividual differences of the countermovement effect for every case. This section thus focuses on the utilization of elastic energy and tendon elasticity which is responsible for the utilization of elastic energy.
In an ultrasonography-based study, Kawakami et al. (2002) pointed out that several interindividual differences were observed in fascicle behavior during CMJ performed with a single joint. This finding implies that there must have been interindividual differences in tendon behavior as well. Some studies have estimated the degree of utilization of elastic energy by using an integrated EMG, which is reported to highly correlate with oxygen consumption during jumping (Bosco et al. 1987). Sugisaki et al. (2004) reported that there was a positive correlation (r = 0.84–0.86) between the extent of the countermovement effect and the contribution of elastic energy. Ito and Saito (1989) indicated that gymnasts accustomed to utilizing elastic energy can use a greater amount of elastic energy during a rebound jump than can swimmers. Belli and Bosco (1992) reported that there is a strong positive correlation (r2 = 0.92) between rebound jump performance and amount of elastic energy utilization measured through an analysis of expired gas. These reports suggest that the amount of elastic energy utilization might have significant impacts on interindividual differences in the countermovement effect.
Some research has been done to examine the relation between the countermovement effect and the mechanical properties of tendons in order to evaluate the likelihood that they are responsible for elastic energy utilization. Kubo et al. (1999) reported that there is a negative correlation between the extent of the countermovement effect achieved through multi-joint vertical jump and tendon stiffness of the vastus lateralis muscle (subjects with stiffer tendons achieve smaller countermovement effects). In contrast, Bojsen–Møller et al. (2005) showed that there is no correlation between these parameters. Subjects with higher tendon stiffness exhibited a higher rate of torque development, which resulted in a higher jump (both noCMJ and CMJ) performance. Burgess et al. (2007) supported this argument: Higher tendon stiffness enabled higher jumps. Kubo et al. (2007a) reported a negative correlation between Achilles tendon stiffness and the countermovement effect by employing a single-joint (ankle joint) jump exercise that eliminated the influence of coordination on the jump. We (Hirayama et al. 2010) employed single-joint (ankle joint) jump exercises (noCMJ and CMJ) for the task and various athletes (sprinters, long distance runners and weightlifters) as subjects, and examined the relationship between Achilles tendon stiffness and jump performance as well as countermovement effect. Our results showed that tendon stiffness neither correlated with jump performance nor the countermovement effect. That is, our results did not support the findings of Kubo et al. (1999/2007a), Bojsen–Møller et al. (2005), nor Burgess et al. (2007). Tendon behavior during SSC exercise is influenced not only by tendon elasticity as measured under static conditions, but also neuromuscular activity and corresponding muscle fibers’ behavior. Moreover, Huijing and Ettema (1988/89) report that the force–length relationship of tendons varies with the manner of muscle contraction. We conclude that the lack of accord in the results of research of tendon stiffness and jump performance as well as the countermovement effect implies that interindividual differences in neuromuscular activity may have a greater influence on jump performance or the countermovement effect than tendon elasticity, at least as measured under static conditions.
16.5 Improvements of the Countermovement Effect
To improve exercise performance by an effect on countermovement, plyometric exercise (exercise with SSC of MTUs) is often employed in training programs (Potach and Chu 2000; Radcliffe and Farentinos 1999). In a systematic review of plyometric training, Markovic (2007) found that the training improved CMJ performance to a greater degree (8.7 %) than for a noCMJ performance (4.7 %). This implies that plyometric training increases the countermovement effect.
According to studies that compare the training effect of traditional resistance exercises and plyometric exercises (or combined training utilizing both types of exercises), the latter creates a greater CMJ performance improvement (Kubo et al. 2007b; Toumi et al. 2004). Plyometric training increases joint stiffness during the braking phase more than traditional resistance training, which is associated with greater jump performance enhancement (Kubo et al. 2007a; Toumi et al. 2004). Toumi et al. (2004) reported increased neuromuscular activity during the switching phase from countermovement to the main movement as a reason for the increase in joint stiffness and CMJ performance. Similar changes in neuromuscular activity were also noted by Chimera et al. (2004), Häkkinen et al. (1990), and Kyröläinen et al. (2005). Ishikawa et al. (2003) utilized ultrasonography and observed that even if the subject jumped from the same height, muscle–tendon behavior during drop jumps varied with the intended jump height (i.e., maximal or submaximal); the greatest tendon shortening was observed when the subject jumped as high as possible. The results of the above studies lead to the following hypothesis: Changes in neuromuscular activity and the corresponding muscle–tendon behavior can be engaged to achieve a greater CMJ performance enhancement after plyometric training than after traditional resistance training.
We (Hirayama et al. 2012) examined neuromuscular activities and muscle–tendon behavior during CMJ and noCMJ. These activities were performed with the ankle joint, before and after a single practice session of plyometric (CMJ) exercise. The results indicated that: (1) An earlier onset of neuromuscular activity of the triceps surae (agonist) muscle occurred after the practice; (2) The muscle–tendon interactions became more apparent after the practice (i.e., fascicle lengthening and shortening decreased with increases in tendon length change, and shortening velocity of the fascicle during the main movement decreased) (Fig. 16.4); and (3) CMJ performance (countermovement effect) improved after the practice (the noCMJ performance was not improved). In most cases, the EMG onset of agonist muscles followed that of the ground reaction force. This result suggests that the ground reaction force started to increase with the passive lengthening of MTUs, and muscle fibers subsequently began to actively contract. In the passive condition, muscle fibers are more easily lengthened than tendon fibers (Herbert and Crosbie 1997). The decrease in time lag from the onset of the passive force to that of muscle activation would decrease the magnitude of muscle fiber lengthening. This phenomenon made the sarcomere length closer to its optimal length. In addition, earlier muscle activation during the braking phase increased the time available for the muscle to develop force. In the main movement, muscle fibers could have exerted a higher force in conjunction with slower shortening by taking advantage of the force–velocity relationship. These phenomena could be the mechanisms for CMJ performance (countermovement effect) improvement after the plyometric exercises. Our findings suggest that muscle–tendon behavior is neurally controlled and that changes in neuromuscular activity and muscle–tendon behavior are involved in jump performance (countermovement effect) improvement.