Introduction to Musculoskeletal Ultrasound Imaging
Gina A. Ciavarra, MD
INTRODUCTION TO ULTRASOUND PHYSICS
Ultrasound is distinct from other imaging modalities in that it uses sound waves rather than ionizing radiation to create images. One of the major components of the ultrasound machine is the transducer, or probe, which serves as the primary contact point between the patient and the sonologist as well as the ultrasound machine. A transducer is any device that converts one form of energy into another. In the case of the ultrasound transducer, electrical energy is converted to ultrasound waves, and vice versa.1,2 The ultrasound probe comprises multiple piezoelectric elements (crystals), which produce the piezoelectric effect that allows this conversion between electrical signal and ultrasound energy. When an electrical signal is transmitted to the transducer, the crystals vibrate at a particular frequency. As the crystals vibrate, a sound wave is created and transmitted into the patient. After the sound wave reflects back to the transducer from the patient, the crystals again vibrate, causing an electrical signal, which can then be converted into an ultrasound image. The crystals can be designed so that they will vibrate at a specified frequency. Within the patient, the sound waves may be reflected at soft tissue interfaces back toward the transducer as described, absorbed by the tissue interface, or refracted.1,3 Refraction is the bending of the sound wave as it passes through tissue interfaces that exhibit differing ultrasound propagation speeds (eg, fluid to muscle or soft tissue to bone).3 It is those ultrasound waves, or echoes, reflected back to the transducer that are converted into the electrical signals that ultimately become an ultrasound image. Ultrasound waves transmitted perpendicular to the surface of the object of interest will be reflected more than nonperpendicular waves. Therefore, for optimal imaging, the structure of interest should be imaged at an angle perpendicular to the probe.3
Coupling or acoustic transmission gel is used to promote the transmission of sound waves into the patient. The gel is positioned between the patient’s skin and the transducer to eliminate air between the 2 surfaces. The introduction of air between the transducer and patient impedes transmission of the sound waves because the air causes reflection of the sound waves at the skin’s surface, thus preventing the waves from entering the patient and resulting in a degraded image or no image at all.3
ULTRASOUND EQUIPMENT/PROBES
When selecting a transducer, the sonologist has to consider what is the optimal frequency of the transducer based on the depth of the structure of interest because the frequency determines the image quality and penetration. Each transducer possesses the capability to produce a range of sound frequencies measured in megahertz (MHz). Higher-frequency transducers are able to produce images of higher resolution. Unfortunately, this comes at the expense of penetration of the sound waves through the tissues. Thus, high frequency transducers are best suited to assess more superficial structures (eg, tendons of the hand and wrist). Lower-frequency transducers are better able to penetrate the soft tissues and therefore better suited to evaluate deeper structures (eg, hip joint); however, this results in lower resolution (Figure 1-1).1,3
Figure 1-1. Ultrasound images obtained with different transducers. (A) Hip injection with curvilinear probe. Note the conical shape of the beam and curvature at the surface (white arrow). (B) Patellar tendon (white arrow) obtained with a linear probe. Note the rectangular shape of the beam. (C) Ulnar nerve (N) perineural injection obtained with a hockey stick probe. The small surface and curvature of the bone (B) make this probe an ideal choice. Needle (white arrow) and anesthetic (a).
Additional considerations include the shape and size of the transducer. The 2 most common types of transducer designations are linear and curvilinear. The linear transducer is optimal for assessment of linear structures (eg, tendons and ligaments) and for use along relatively flat surfaces such as the extremities. The sound waves transmitted from the linear probe propagate parallel to the probe surface and result in a rectangular image. With a curvilinear probe, the ultrasound waves transmit in a radial path from the probe surface, resulting in a wider field-of-view (FOV) image as compared with the linear probe. An additional type of linear probe with a small footprint (sometimes called a hockey stick probe due to its shape) is especially useful in imaging small structures in the hand, wrist, foot, and ankle (Figure 1-2).1–3
Figure 1-2. (A) Curvilinear, (B) linear, and (C) hockey stick (small footprint) ultrasound transducers.
The availability of the different probes and capabilities of individual ultrasound machines vary based on size, power, and cost. Larger ultrasound systems have more powerful computing capabilities and allow for the use of ultrahigh frequency transducers, resulting in high-resolution images of superb quality. Smaller, more portable machines, which may be the size of a briefcase, are also available. These have the advantages of lower cost and portability but are more limited in their ability to produce high-resolution images and have fewer advanced applications (eg, real-time fusion with computed tomography scan/magnetic resonance imaging). As the technology continues to advance, the technological differences between the 2 types of machines will become less pronounced.2
SCAN TECHNIQUE/IMAGE APPEARANCE
When the transducer is placed on the patient and an image is created, the more superficial structures are those closest to the transducer along the superior aspect of the image. The deeper structures, farthest from the transducer, are along the inferior aspect of the image. Typically, when imaging a structure in long axis, the convention is to have the more proximal aspect of the structure to the left side of the image and the more distal aspect to the right. The most important point is to be consistent across imaging studies. The left and right sides of the image can be easily switched by using the invert button on the ultrasound machine or by rotating the probe 180 degrees.2
Once oriented, the sonologist should optimize the image to improve the resolution and visualization of the structure in question. This begins even before initiating the study with appropriate probe selection. As discussed, higher-frequency transducers are preferred for the evaluation of more superficial structures (eg, hand, wrist), whereas lower-frequency transducers are preferred for deeper structures (eg, hamstring origins, hip joint). Linear transducers are preferred unless evaluating a deeper structure, where the lower-frequency curvilinear probe is preferable.1,3
After selecting the appropriate probe, the image should be optimized using some of the buttons available on the ultrasound machine. First, the depth of the ultrasound beam should be adjusted. This is accomplished by ensuring that the object of interest is centered within the image. Second, the number of focal zones should be adjusted. An ultrasound beam is narrowed, or focused, not simply at one point but over a range of depths also known as a focal zone. More specifically, a focal zone is defined as a range of depths over which the ultrasound beams are most narrowed (or focused). The number of focal zones should be adjusted to include the fewest number of focal zones while encompassing the entire region of interest being scanned such that the ultrasound beam is most focused on the target structure, improving image resolution. Once the number of focal zones is selected, the depth should also be adjusted to optimize evaluation of the object of interest as previously discussed (Figure 1-3). Finally, the gain, or image brightness, should be modified to improve the image quality. Because different tissues attenuate the sound waves to varying degrees, adjusting the gain can optimize the image to account for these differences, resulting in a more uniform appearance of the image.2,3
Figure 1-3. Adjusting the depth and focal zones (median nerve). (A) Suboptimal depth (D) and focal zone (FZ) result in a blurry image with poorly centered median nerve (MN). (B) Depth (D) has been improved for better centering of the nerve (MN), but image remains blurry due to suboptimal focal zone (FZ). (C) Optimal focal zone (FZ) improves blurriness of the median nerve (MN), but poor depth (D) selection results in poorly centered nerve. (D) Both depth (D) and focal zone (FZ) are optimized with improved image quality of the median nerve (MN).
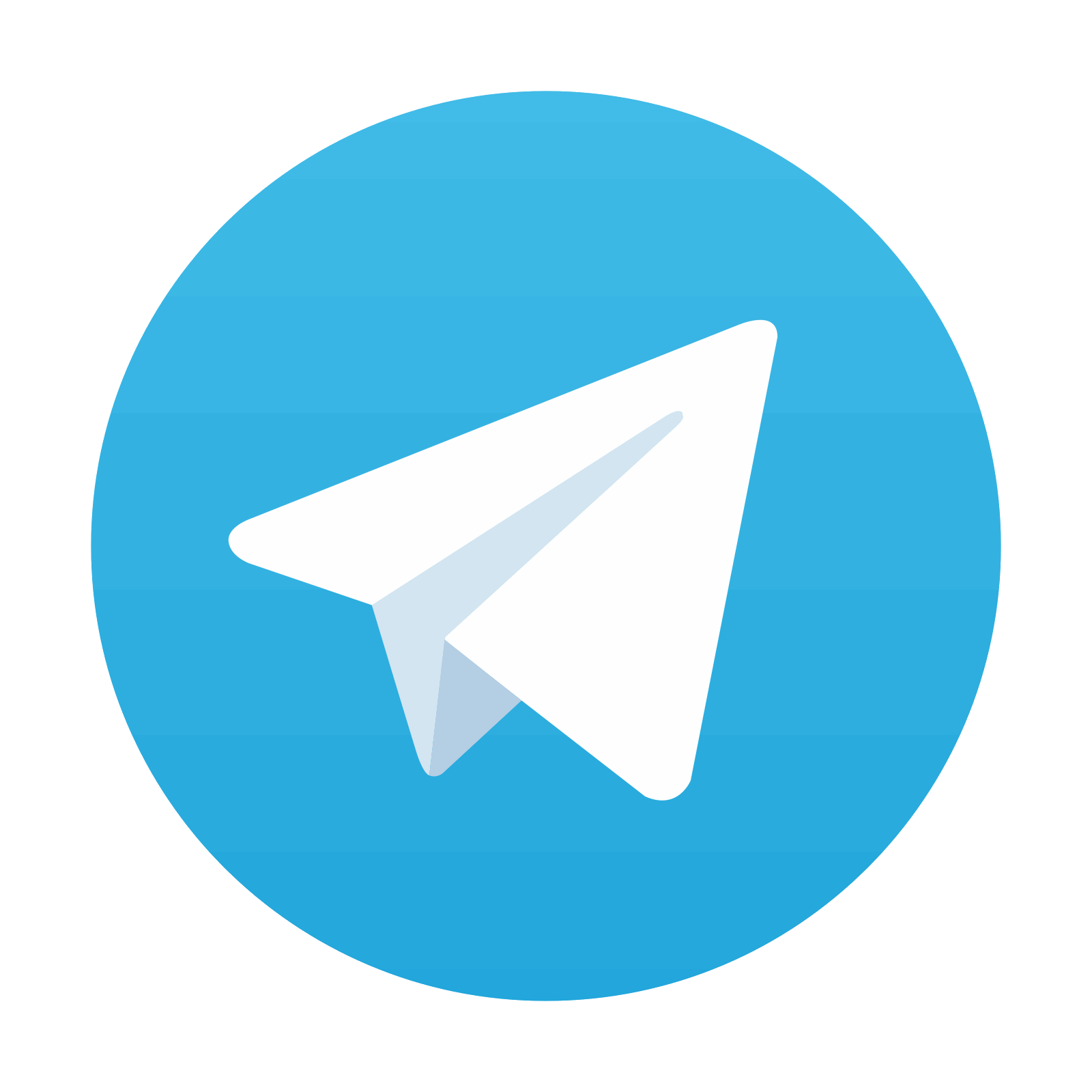
Stay updated, free articles. Join our Telegram channel

Full access? Get Clinical Tree
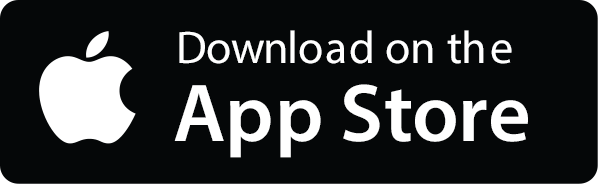
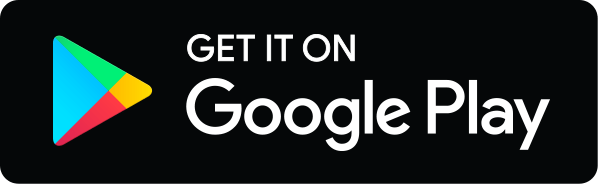