, Ardiyansyah Syahrom2 , Muhamad Noor Harun2 and Mohammed Rafiq Abdul Kadir3
(1)
Department of Applied Solid Mechanics and Design, Universiti Teknologi Malaysia, Johor, Malaysia
(2)
Sport Innovation and Technology Centre (SITC), Universiti Teknologi Malaysia, Johor, Malaysia
(3)
Faculty of Biosciences and Medical Engineering, Department of Clinical Sciences, Universiti Teknologi Malaysia, Johor, Malaysia
Arthritis is the main issue that accounts for ankle joints to have operative (arthrodesis or ankle replacement) or non-operative management (analgesics and anti-inflammatory medication, activities modification, physiotherapy, orthotics (bracing) and intra-articular injections) [1, 2]. At the ankle joint, primary osteoarthritis is less frequent but secondary arthritis and trauma occurrences are more frequent compared to the knee and hip joints [1]. The earliest known treatment of end-stage arthritis of the ankle joint was arthrodesis, known as ankle fusion and considered as a ‘gold standard’ treatment for patients suffering from this condition [1, 3]. This treatment is increasing in popularity because of the arthroscopically assisted and minimally invasive [1, 3, 4]. An alternative to arthrodesis is the ankle replacement which is for selected patients. The advantage of ankle replacement using prosthesis is the installation of the physiologic motion of ankle activity. This will improvise gait activities which could also reduce limping and protect other joints [2]. The major complication related to failure of the ankle replacement is loosening of the component [2, 5–7].
Aseptic loosening of joint replacement is becoming a crucial factor of total ankle replacement (TAR) failures and revision. Nevertheless the expanding development of joint replacement is impressive and shows promising results. The main factor that limits the longevity of total ankle replacement (TAR) is particle induced osteolysis (bone resorption). Polyethylene wear particles are generated from relative movement between contacting components (soft-on-hard (SoH)). These wear particles stimulate an immune response that initiate a cascade of adverse tissue responses leading to osteolysis and the subsequent loosening of the implant component [8, 9]. The loosening of the ankle replacement will cause a greater impact to the patient such as severe pain around the ankle. When this happens, surgery is required in order to revise the ankle [9].
Over the decades, there were big improvements in designs for the first generation of ankle replacement since the 1970s until now. Studies have been done to come out with the design of TAR, which imitated the natural anatomy of the ankle in order to preserve human movements [10]. The mobile ankle-type was introduced to perform the physiological ankle mobility. The components of the mobile ankle consisted of a spherical convex tibial component, a talar component with radius of curvature in the sagittal plane longer than that of the natural talus and a corresponding meniscal component [11–13]. The new generation of ankle replacement is fully conforming and completely congruent in design to provide greater stability and resistance to wear. Another advantage of congruent surfaces is that the load from the body weight acts on the surfaces and is distributed well across those surfaces. This leads to decreasing wear due to reduced contact pressure [5].
The investigations of wear mechanism of UHMWPE of ankle joint replacement were reported by means of experimental testing. The laboratory study was carried out using simulators to install originality of realistic loading and kinematic conditions of the ankle joint. Preoperative in vitro wear predictions are useful and requires for implant design optimization of total ankle replacement (TAR). However, it is costly as well as time consuming. Currently, there is no wear prediction on total ankle replacement (TAR) by using finite element analysis.
1.1 Ankle Joint Anatomy
The lowest joint in the human body is the ankle joint. It has a complex mechanism which is a build-up of four bones namely tibia, fibula, talus, and calcaneous as shown in Fig. 1.1.
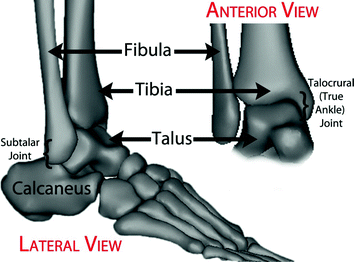
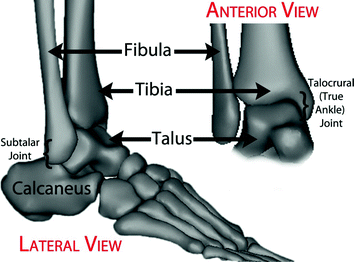
Fig. 1.1
Anatomy of the ankle joint
The ankle joint motions can be described in three orthogonal planes. These are the plantar/dorsi flexion (sagittal plane), inversion/eversion (frontal plane) and internal/external rotation (transverse plane) as shown in Fig. 1.2. The motions involved along the three planes are pronation/supination in which pronation involves dorsiflexion, eversion and external rotation while supination involves plantar flexion, inversion and internal rotation.
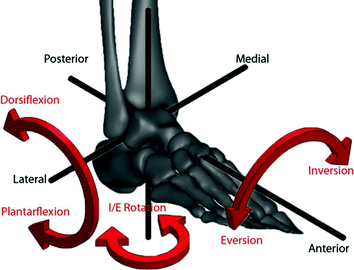
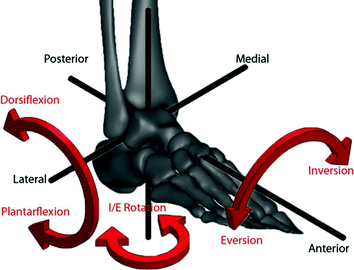
Fig. 1.2
Motions of the ankle joint
Two types of joints in an ankle complex joint are the talocrural (true ankle) joint and the subtalar joint. The talocrural joint is formed when lower leg bones of the tibia and the fibula, meets the anklebone, called the talus. This joint actively supports the primary motion, the flexion/extension motion which is the dorsiflexion that allows the foot to move up whilst the plantar flexion permits opposite direction of movement. It also supports a portion of the total ankle rotation movements in extreme condition, such as the patients stumble or fall. The talocrural joint could also support a little inversion and eversion movement. Meanwhile, the subtalar joint is formed between two bones which are the talus and the heel bones called the calcaneous. The talus rests on the calcaneous. This joint supports mainly inversion and eversion and also a portion of the ankle rotation movement [14].
Ligamentous structure on the ankle joint is the most important part in providing stability while performing any physical activity [15]. Ligaments of the ankle joint connect the bones together while tendons connect muscle to bone. Five ligaments provide main support to the ankle joint. There are many tendons located on the ankle to move the ankle and the toes. The largest and strongest tendon in the foot is known as the Achilles tendon and essentially supports activities such as walking, running and jumping. It is located at the back of the ankle. The joints in human bodies are covered by cartilage which is a flexible connective tissue and allows the bones to move efficiently. The cartilage in lower limb joints carries body weight and allows for shock absorption such as at the ankle, knee and hip. Even though it looks thin, it is not as hard and rigid as the bone but is stiffer and less flexible than the muscle to last for a lifetime, as long as it is not damaged.
Besides ligamentous structures, talocrural joints too help in contributing to ankle stability. Morphometry of talus on ankle joint is necessary due to its function and importance in providing better stability and mobility on the human ankle joint. In previous reports, the morphometry of talus was studied using various methods [14, 16]. One of the most significant morphometric parameters is the trapezium shape of trochlea tali. The superior articular surface of trochlea tali is typically measured using 3D imaging [14]. The articulating surface of talus particularly contributes to the stability of 70 % of the antero-posterior, 50 % of the inversion/eversion and 30 % of the internal/external rotation [7, 17]. Regularly, the measurement method for ankle morphometry is by using 2D plain radiography as a reference in understanding the human ankle joint. Currently, there is a new measurement method using 3D morphometric study of the trapezium shape of the trochlea tali. The morphological parameters are anterior width, posterior width, trochlea tali length and angle of the trapezium shape, measured to compare between the male and the female and between left and right bones. All four morphometric parameters have shown greater values in the male group although there were only small differences between the left and right talus bone [13].
1.1.1 Ankle Biomechanics
The biomechanics of the lower extremities is considered complex especially for the hip joint and knee joint. It was found that there was a lack of study carried out on the ankle joint. However, the data was obtained from various techniques and approaches. There are inverse dynamic models, computer optimization models included with ligamentous structure, studies of gait cycle using fluoroscopes and even with instrument implants to encounter precisely the loads in a given joint. Recently, the attention at the ankle joint is growing especially due to diagnosis and treatment of complications occurring.
The motions along the three axes were based on the period of time from one heel strike to the next heel strike of the same limb, otherwise known as gait cycle. Normally, the gait cycle is divided into two major groups as shown in Fig. 1.3. The two phases are the stance phase (a phase whereby the leg is in contact with the ground during walking) and the swing phase (a phase whereby the leg swings freely during walking). The stance phase which is also known as the active flexion phase can be classified into five subcategories which are heel strike or initial contact, foot flat, mid-stance, heel-off or push off, and toe-off [18].
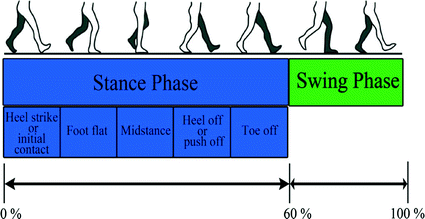
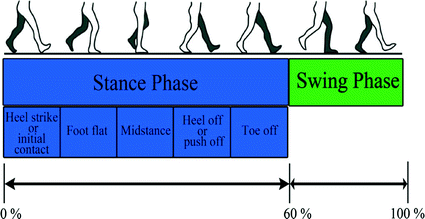
Fig. 1.3
Schematic of normal gait
1.1.2 Loading on the Ankle
The data of loading on the ankle was obtained through walking gait analysis from several experimental setups. The ground force that acted on the foot passed through to the ankle via loading that acted upon the talus and the tibia/fibula. The tibia/fibula is assumed as one segment in most inverse dynamic models because the load transfer through the fibula is very low compared to the tibia. Therefore, the tibio-talar joint between the talus and the tibia bone interface remains as the primary load transfer [19]. The load predicted regarding to their model pattern has been agreed vigorously by electromyography (EMG) activation pattern during walking gait that the maximum compressive load on the ankle was about 5.2 times body weight (BW) which illustrates on Fig. 1.4.
Another research has been developed on the joint force profile at the ankle joint with different subjects as shown in Fig. 1.5 [21]. This study was based on three different subjects which were normal/healthy patients, patients with disabling joint disease (preoperative) and patients with enduring total ankle replacement (TAR) (postoperative) to measure actual forces. The kinematics data and ground force reaction was converted using the 2D inverse dynamic model to determine the joint force profile on the ankle joint. The maximum compressive load was to be 4.5–5.5 of BW for the healthy/normal patients and 3.0 of BW for the preoperative and postoperative patients. This maximum load on the ankle joint can be about five times of the body weight while on the hip and the knee about three times of the body weight.
Procter P and Paul JP developed a loading profile of the ankle joint using 3D analysis of stance phase via a camera station and force plate system as shown in Fig. 1.6 [22]. They developed the model with ligamentous structure and then assigned into four functional groups. This study was admitted with other EMG studies and the maximum load was determined to vary from 2.9 to 4.7 times of body weight. The extent of the load was affected since every patient had different characters.
Another researcher has studied the previous data using finite element models to determine the contact pressure, muscle and ligament forces, and also displacement of ankle replacement in two different conditions of gait cycle which were stance phase and swing phase (passive flexion) [23]. Another used joint force profile as shown in Fig. 1.4 that reduced the load to 2.3 times of the body weight rather than 5.2 BW. They identified the differences when compared to the load data on the hip in vivo analysis measurement by another researcher [24] and proportionally scaled the factor by 2.25.
Even though the maximum load on the ankle joint differed, the ankle joint reaction profile was consistent with the difference model. It was necessary to extend the study of the loading on the ankle joint in various conditions and models because it will be valuable to researchers, as they would refine the study to benefit others.
1.1.3 Kinematics of the Ankle
The ankle joint motions are plantar/dorsi flexion (sagittal plane), inversion/eversion (frontal plane) and internal/external rotation (transverse plane). The previous work of the ankle joint kinematics as in Fig. 1.7 showed that in the sagittal plane, the range of motion was 16° of plantar flexion and 15.2° of dorsi flexion while in the frontal plane, the range of motion was 10.4° of inversion and 5° of eversion. Furthermore, in the transverse plane, the range of motion was 8.5° of external rotation and 2.2° of internal rotation [25, 26].
