Intraoperative imaging
1 The role of imaging in MIPO 77
2 Hazards of radiation exposure 77
2.1 Radiation and risks 77
2.2 Radiosensitivity 78
3 Mobile image intensification x-ray apparatus or C-arm 79
3.1 The x-ray apparatus 79
3.2 Imaging modes 80
4 Safety measures to ensure reduction of radiation from x-ray apparatus used for intraoperative imaging 80
4.1 Training 81
4.2 Protective equipment 81
4.3 Protective procedures 82
4.4 Positioning of patient, surgeon(s), ORP, and C-arm 82
4.5 Collimation 85
4.6 Positioning of the screen 86
4.7 Obtaining maximum image 86
4.8 Obtaining imaging in the correct orientation 86
4.9 Specific positioning for MIPO procedures 86
5 C-arm requirements and setup in the operating room 86
6 Documenting imaging 87
6.1 Memory modules 87
6.2 Hard copies 87
6.3 Picture archiving computing systems 87
6.4 Three-dimensional models 87
6.5 Navigation 87
6.6 Flat-panel 87
7 Conclusion 87
8 References 88
9 Further reading 88
The role of imaging in MIPO
The increased use of minimally invasive plate osteosynthesis (MIPO) has led to an escalation in the amount of radiation needed to execute a surgical plan to achieve fracture fixation. As surgeons learn better ways to achieve reduction and fixation without opening the fracture site, there is increasing reliance on intraoperative imaging. The advantages of achieving minimally invasive fracture fixation must be balanced against the principles governing the use of radiation.
In minimally invasive osteosynthesis (MIO) and MIPO, imaging is not only an essential requirement for preoperative diagnosis and planning, but an integral part of the surgical procedure itself. Without intraoperative imaging, MIO and MIPO are not feasible. With limited windows through the skin and no direct visualization of the fragments intraoperatively, image intensifiers become a key surgical adjunct. However, as with any surgical equipment, the risks, benefits, and limitations must be thoroughly assessed and appreciated.
Modern plain x-rays normally yield minimal radiation risk to the patient, but frequent, prolonged, and repetitive use of intraoperative image intensifiers greatly increase the amount of radiation exposure to the surgeons and the operating room personnel (ORP). The greater the exposure to radiation, the greater the risk of harmful damage to cellular function.
It is therefore essential for the MIPO surgeon to understand the type and dosage of x-rays generated at his or her institution, whether conventional or digital, and the type of magnification achieved. It is important to know the size and type of image intensifier used in the operating room and the most effective way to limit exposure of patient and staff to radiation. This knowledge will allow for maximum benefit by ensuring minimum exposure to everyone involved.
International radiation protection guidelines established by the International Commission on Radiation Protection (ICRP) recommend the following core principles:
Justification of x-ray application
According to international and European law a surgeon must justify every use of imaging in the operating room, taking into consideration the benefit for the patient in relation to the detriment to the health and safety of the ORP. This should be documented in every surgical report.
The ALARA principle: All radiation exposures must be medically justified and the individual dose rate should be as low as reasonably achievable (ALARA). The indication for every x-ray, even in the operating room, should be challenged each time. This is the principle of radiation exposure put forth by the National Committee on Radiation Protection in 1954. In practice this means keeping occupational and nonoccupational absorbed dose equivalents well below maximum allowed levels by observing proper safety procedures and avoiding unnecessary exposure.
Hazards of radiation exposure
It is the responsibility of every surgeon to be aware of the risks of ionizing radiation to all who may be exposed during surgery: surgeon, operating team (including the anesthesiologist), and patient. Any changes caused to the body by radiation are largely irreversible. Normal and abnormal variations in the host may result in undue susceptibility of cells and organs to certain forms of radiation. The only effective method of minimizing exposure is to scrutinize every indication for intraoperative radiation, reduce radiation emissions, promote awareness of radiation hazard among staff and ensure that physical protection is used.
Radiation and risks
X-rays are a type of electromagnetic radiation, like visible light, radio waves, and cosmic waves and they have characteristic wave properties defined as wavelength and frequency. All forms of electromagnetic radiation emit photons (packets of energy). X-rays have a short wavelength and a high frequency. Being high-energy, x-rays cause ionization (removal of electrons) by hitting atoms that make up organic molecules. Ionized atoms will not bond properly on a molecular level, therefore causing disruption to the molecule and disrupting cellular function.
This ionization effect from x-rays can be separated into:
Direct effects, which occur when molecules within the cell are disrupted due to ionization of their constituent atoms by direct energy transfer from the x-ray. This can disrupt any molecule in the cell involved in any cell process, including deoxyribonucleic acid (DNA).
Indirect effects of x-rays to biological organisms, which occur via the ionization of water to form free radicals, which in turn react with, oxidize, and damage molecules, including DNA. As human tissues contain approximately 80% water, radiolysis of water and its indirect effect on cellular molecules account for 95% of the effects of x-rays on the organism.
If the DNA molecule is affected because millions of photons pass through the human tissue during x-ray examination, the resulting damage may be permanent or reversible by DNA repair processes. Most DNA damage is repaired immediately. DNA damage from ionization is usually either a change in the nitrogenous base pair (changing the genetic code) or cross-linking of strands (interference with translation of the code). If DNA damage is not repaired, the alteration of DNA will be permanent (mutation), which may be the starting point of a tumor formation, sometimes with a latency of many years.
Damage to DNA may result in:
Genetic repercussions
Instant cell death (large doses cause DNA breakdown)
Reproductive death (cells unable to divide)
Interphase death (cell death without attempt at division)
Mitotic death (cell death after one or more divisions)
Mitotic delay (delay in cell division)
Radiation units are:
Gray (Gy) represents the energy deposited in the material (tissue). This reflects the physical effect (1 Gy = 1 Joule/kg).
Sievert (Sv) is a dose equivalent, which reflects the biological effect (1 Sv = 1 Joule/kg).
Radiosensitivity
The radiosensitivity of cells is directly proportional to their reproductive ability and inversely proportional to their degree of differentiation (law of Bergonié and Tribondeau). Muscle and bone are relatively radio resistant, but skin, reproductive cells, and hematopoietic cells are radiosensitive.
Radiation risk is also age related. Exposure to radiation in younger patients has a much higher multiplication factor for risk, because the tissues of children and adolescents are more radiosensitive. Furthermore, their life span is longer than the latent period of cancer development. Limiting x-rays in pregnant women is vital, and sometimes an open reduction should be performed instead of MIPO technique to reduce the radiation load to the patient and fetus.
The biological damage to a living organism from radiation exposure is known as a somatic effect. Early effects are due to high-dose radiation and not typical of diagnostic imaging radiation. This may occur from damaged or very old x-ray apparatus. Chronic effects appear months or years after exposure and can be seen with multiple low-dose radiation exposure over years, such as in diagnostic radiology. The main categories of these chronic somatic effects are:
Cancer induction (eg, skin cancers, leukemias)
Damage to the fetus
Cataractogenesis
Thyroid cancer (85% of papillary carcinomas are radiation induced from a carcinogenic dose of radiation. 100 mSv (millisieverts) is sufficient to induce thyroid carcinoma
Shortening of life span
Stochastic effects (no threshold) are effects in which the magnitude of risk is related or proportional to the cumulative dose. The severity of the effect is not related to the dose. These include genetic damage and carcinogenesis. Carcinogenesis includes skin cancers, especially on the hands of medical radiation workers, leukemia, and thyroid carcinomas. Cataract formation and an effect on fertility are other stochastic effects. Since the introduction of safety measures there has been no reported increase in rates of cancer in this subgroup relating to operative radiation exposure.
Deterministic effects (threshold limited effects) are effects that will not occur below a certain threshold and whose severity depends on the dose. Examples are radiation cataract, leukemia, thyroid cancer ( Fig 5-1 ), and reproductive cell damage. The threshold values per year are:
Thyroid: 300mSv
Eye: 150 mSv
Hand: 500 mSv

Mobile image intensification x-ray apparatus or C-arm
The image intensifier (or C-arm) is a mobile x-ray apparatus that has revolutionized the surgery of fracture fixation. Surgeons have become very dependent on image intensification when performing fixation, particularly MIO. Minimally invasive osteosynthesis without a C-arm is almost equivalent to operating in the dark. The surgical team receives, to a large extent, accurate images of fracture reduction and placement of implants. Every x-ray apparatus has different functions and modes. It is essential for every trauma surgeon to become familiar with the x-ray apparatus available in his or her operating room, even when in some hospitals a specific x-ray technician is on-site in the operating room.
The x-ray apparatus
The C-arm itself consists of a radiation source with a collimator screen (x-ray tube unit), which allows gamma rays to be beamed to the photosensitive receiver array (image intensifier). The image appears on the display screen ( Fig 5-2 ). The received image that has traversed the body will differ in intensity and the image captured is processed electronically to produce an enhanced picture on a digital screen. The quality of the image is dependent upon the relationship of the beam direction to the target that is being visualized. For high-quality images the beam of the x-ray should travel perpendicular to the bone, with the image intensifier as close to the patient as possible. The radiation power (kilovolt, kV) and exposure (milliampere, mA) are usually automatically set to produce an optimal image and vary with the mass through which the beam passes.
Obese patients or patients with large limbs produce more scattered radiation, reflected off the patient, because of the increased amount of energy needed to image bone tissue. Additional protective devices for surgeons and ORP should be considered and ORP should stand a safe distance away from the x-ray source during surgery.
The amplifier diameter normally varies from 11 to 32 cm. The direct beam width is constrained not to exceed this diameter, but the scatter is increased the larger the amplifier, due to a wider beam. A smaller image intensifier diameter can increase the patient entrance dose ( Table 5-1 ). Laser aiming devices on modern image intensifiers enable accurate targeting and allow for smaller image intensifier diameters to be used.
A common but unsafe technique is to position the amplifier upside down to use it as a “hand table.” This position allows beam scatter to reflect off metallic instruments toward the eyes and neck of the surgeon and should never be allowed.
Intensifier diameter | Relative patient entrance dose | |
32 cm (12 in) | Dose 100 | |
22 cm (9 in) | Dose 150 | |
16 cm (6 in) | Dose 200 | |
11 cm (4.5 in) | Dose 300 |

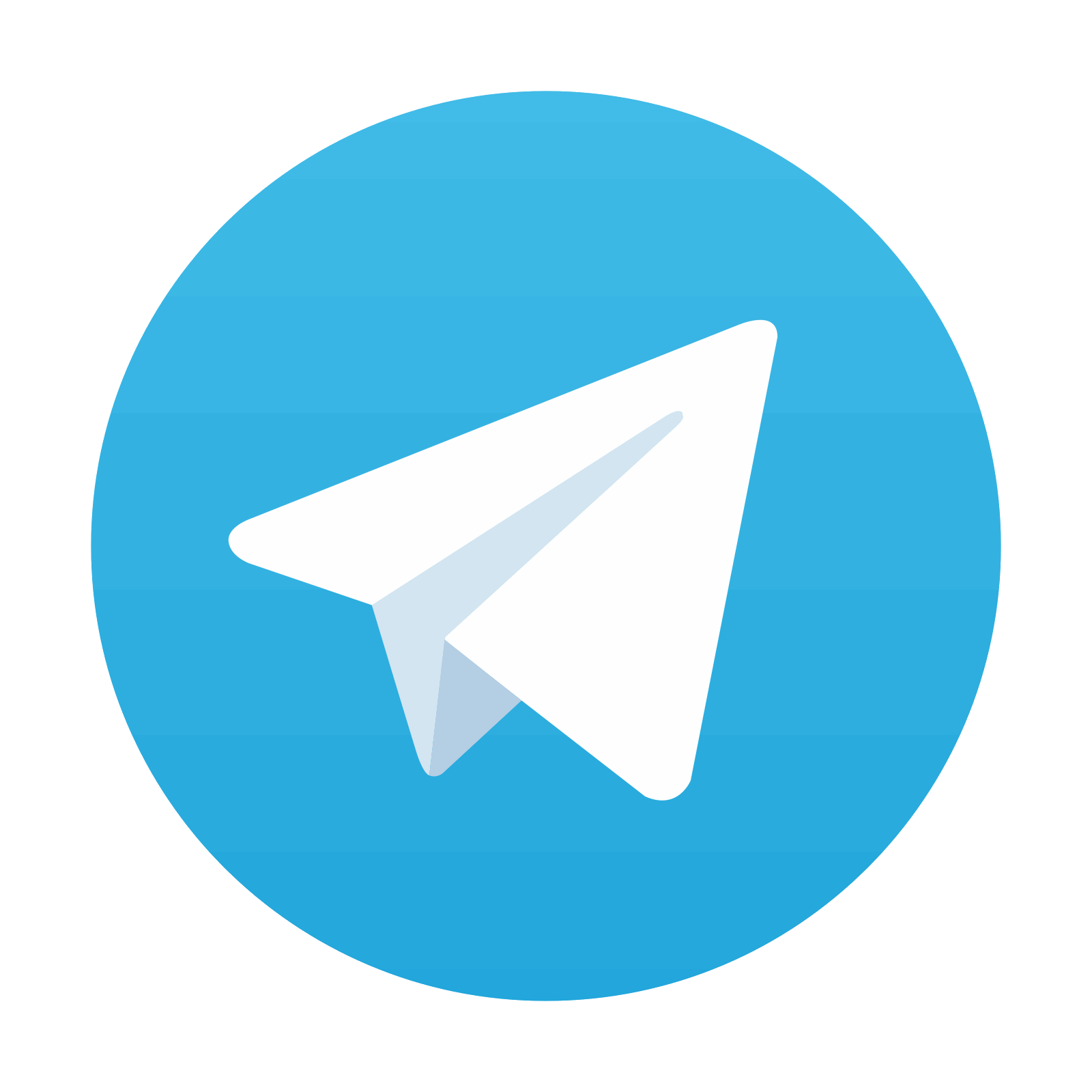
Stay updated, free articles. Join our Telegram channel

Full access? Get Clinical Tree
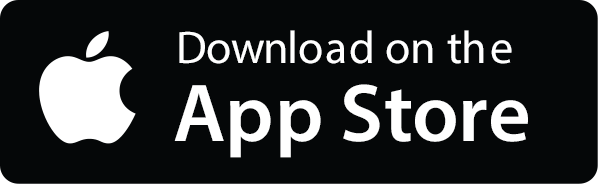
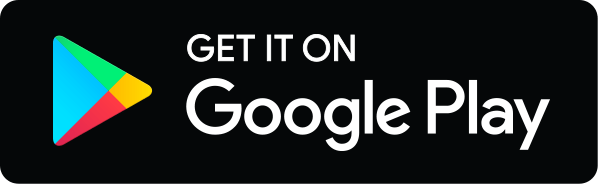
