FIGURE 30-1 Longitudinal meniscus tear associated with tibial eminence fracture.
Signs and Symptoms of Fracture of the Tibial Spine (Intercondylar Eminence)
Patients typically present with a painful, swollen knee after an acute traumatic event. They are usually unable to bear weight on their affected extremity. On physical examination, there is often a large hemarthrosis because of the intra-articular fracture and limited motion due to pain, swelling, and occasionally mechanical impingement of the fragment in the intercondylar notch. Sagittal plane laxity is often present, but the contralateral knee should be assessed for physiologic laxity. Gentle stress testing should be performed to detect any tear of the medial collateral ligament (MCL) or lateral collateral ligament (LCL) or physeal fracture of the distal femur or proximal tibia.
Patients with late malunion of a displaced tibial spine fracture may lack full extension because of a mechanical bony block. Patients with late nonunion of a displaced tibial spine fracture may have increased knee laxity, with a positive Lachman examination and pivot-shift examination.
Imaging and Other Diagnostic Studies for Fracture of the Tibial Spine (Intercondylar Eminence)
Radiographs typically demonstrate the fracture, seen best on the lateral and tunnel/notch views. The lateral radiograph is most useful in fracture classification. Radiographs should be carefully scrutinized, as the avulsed fragment may be mostly nonossified cartilage with only a small, thin ossified portion visible on the lateral view.
To guide treatment, important information to ascertain from the radiographs includes the classification type, amount of displacement, size of the fracture fragment, comminution of the fracture fragment, and status of the physes. Bone age radiographs may be obtained for patients around the time of skeletal maturity, if transphyseal screw fixation is being considered.
MRI is typically not required in the diagnosis and management of tibial eminence fractures in children, particularly because operative cases will undergo a thorough diagnostic arthroscopy to assess for possible concurrent intra-articular knee injuries, such as meniscal tears, but may be helpful to confirm the diagnosis in cases with a very thin ossified portion of the avulsed fragment or evaluate for suspected associated injuries.183,231,352
Classification of Fracture of the Tibial Spine (Intercondylar Eminence)
The classification system of Meyers and McKeever,279 which is based on the degree of displacement of the tibial spine fragment, continues to be widely used to classify fractures and guide treatment (Fig. 30-2).
FIGURE 30-2 Classification of tibial spine fractures. A: Type I—minimal displacement. B: Type II—hinged posteriorly. C: Type III—complete separation.
1. Type I—minimal displacement of the fragment from the rest of the proximal tibial epiphysis.
2. Type II—displacement of the anterior 1/3 to 1/2 of the avulsed fragment, which is lifted upward but remains hinged on its posterior border in contact with the proximal tibial epiphysis.
3. Type III—complete separation of the avulsed fragment from the proximal tibial epiphysis, with upward displacement and rotation.
Radiographs of these fracture types are shown in Figure 30-3. The interobserver reliability between type I and type II/III fractures is good; however, differentiation between type II and III fractures may be difficult.219
FIGURE 30-3 Stages of displacement of tibial spine fractures. A: Type I fracture, minimal displacement (open arrow). B: Type II fracture, posterior hinge intact. C: Type III fracture, complete displacement and proximal migration.
Zaricznyj421 further classified a type IV fracture to describe comminution of the tibial eminence fragment. An alternative classification scheme more broadly classifies tibial spine fractures as one of several different types of proximal tibial fractures, based on mechanism of injury, but has not gained wide acceptance or use.288
Outcome Measures for Fracture of the Tibial Spine (Intercondylar Eminence)
Although healing of the fracture on radiographs is important for decisions on timing of return to activities, the most important long-term outcome measures used to assess the results of tibial spine fracture fixation include functional knee scores, such as the Pedi-IKDC225 and Lysholm364 knee scores, and a patient’s ability to make a full return to activities of daily life and sports activities, which can be assessed using the Marx or Tegner activity scores.264 Asymmetry in the Lachman examination is not uncommon,39,408 even after anatomic fixation of fractures, but has not been shown to correlate with symptomatic instability or long-term clinic results.
PATHOANATOMY AND APPLIED ANATOMY RELATING TO FRACTURE OF THE TIBIAL SPINE (INTERCONDYLAR EMINENCE)
The intercondylar eminence is that part of the tibial plateau lying between the anterior poles of the menisci. It is triangular, with the base of the triangle running along the anterior border of the proximal tibia. In the immature skeleton, the proximal surface of the eminence is covered entirely with cartilage. The ACL attaches in the interspinous region of the eminence and just anteriorly to the tibial spines, with separate slips anteriorly and laterally as well (Fig. 30-4). The ligament originates off the posterior margin of the lateral aspect of the intercondylar notch. The anterior horn of the lateral meniscus is typically attached in the region of the tibial intercondylar eminence just adjacent to the ACL insertion. In 12 patients with displaced tibial spine fractures which did not reduce closed, Lowe et al.249 reported that the anterior horn of the lateral meniscus consistently remained attached to the tibial eminence fracture fragment. The posterior cruciate ligament (PCL) originates off the medial aspect of the intercondylar notch and inserts on the posterior aspect of the proximal tibia, distal to the joint line.
FIGURE 30-4 Anterior cruciate ligament insertion on the tibial eminence.
Meniscal or intermeniscal ligament entrapment under the displaced tibial eminence fragment has been reported and may be a rationale for considering arthroscopic or open reduction in displaced tibial spine fractures (Fig. 30-5).67,75,119,220 Meniscal entrapment prevents anatomic reduction of the tibial spine fragment, which may result in increase of a block to extension and/or eventual anterior laxity.151,187,272,311,408 Furthermore, meniscal entrapment itself may cause knee pain after fracture healing.75 Falstie-Jensen and Sondergard Petersen, Burstein et al., and Chandler and Miller67,75,119 have all reported cases of meniscal incarceration blocking reduction of type II or III tibial spine fractures in children. The prevalence of meniscal entrapment in tibial spine fractures may be common for displaced fractures. Although the anterior horn of the lateral meniscus may remain attached to the tibial eminence fracture fragment, it may instead remain attached to the intermeniscal ligament, generating a soft tissue complex that may become incarcerated between the elevated bony or cartilaginous fracture fragment and its underlying bony bed. Mah et al.255 found medial meniscal entrapment preventing reduction in 8 of 10 children with type III fractures undergoing arthroscopic management. In a consecutive series of 80 skeletally immature patients who underwent surgical fixation of hinged or displaced tibial eminence fractures which did not reduce in extension, Kocher et al.220 found entrapment of the anterior horn medial meniscus (n = 36), intermeniscal ligament (n = 6), or anterior horn lateral meniscus (n = 1) in 26% (6/23) of hinged (type II) fractures and 65% (37/57) of displaced (type III) fractures. The entrapped meniscus can typically be extracted with an arthroscopic probe and retracted with a retaining suture (Fig. 30-6).
FIGURE 30-5 Meniscal entrapment under a tibial eminence fracture.
FIGURE 30-6 Retraction of an entrapped anterior horn medial meniscus using a retaining suture.
TREATMENT OPTIONS OF FRACTURES OF THE TIBIAL SPINE (INTERCONDYLAR EMINENCE)
Treatment options include cast immobilization,240,286 closed reduction with immobilization,311,409,410 open reduction with immobilization,286 open reduction with internal fixation,289,311 arthroscopic reduction with immobilization,271 arthroscopic reduction with suture fixation,171,196,240,255,256 or suture mattress technique,259 and arthroscopic reduction with wire,36 screw fixation,46,240 percutaneous K-wire fixation,131,161,170 anchor fixation,397 or bioabsorbable fixation.349,416 Studies of the biomechanical strength of internal fixation suggest similar fixation strength between bioabsorbable and metallic internal fixation257 and increased fixation strength of suture fixation over internal fixation,61,111 with advocates of both bioabsorbable implant fixation and suture techniques emphasizing the advantage of avoiding subsequent hardware removal procedures.259,416
Nonoperative Treatment of Fracture of the Tibial Spine (Intercondylar Eminence)
Indications/Contraindications
Closed treatment is typically utilized for Type I fractures and for Type II or III fractures that successfully reduce with closed maneuvers (Table 30-1).
TABLE 30-1 Fracture of the Tibial Spine (Intercondylar Eminence)
Techniques
Closed reduction is usually performed with placement of the knee in full extension or 20 to 30 degrees of flexion. Aspiration of the intra-articular fracture hematoma, with or without the intra-articular injection of a short-acting local anesthetic, has historically been common practice prior to reduction, but is not required for a successful reduction and is performed less commonly today. Radiographs, most importantly the lateral view, are utilized to assess adequacy of reduction. If the proximal fracture fragment includes bony segments of the medial or lateral tibial plateau, extension may affect a reduction through pressure applied by medial or lateral femoral condyle (LFC) congruence (Fig. 30-7). Fractures confined within the intercondylar notch, however, are unlikely to reduce in this manner. Portions of the ACL are tight in all knee flexion positions; therefore there may not be any one position that eliminates the traction effect of the ACL on the fragment. Interposition of the anterior horn medial meniscus or intermeniscal ligament may further block reduction.
FIGURE 30-7 Reduction of type II tibial fracture with knee in 10 to 20 degrees of flexion.
Outcomes
Closed reduction can be successful for some type II fractures, but is infrequently successful in type III fractures. While Bakalim and Wilppula33 reported successful closed reduction in 10 patients, and Meyers and McKeever280 recommended cast immobilization with the knee in 20 degrees of flexion for all type I and II fractures, Kocher et al.220 reported successful closed reduction in only 50% of type II fractures (26/49). However, no type III fractures were able to be close reduced (0/57), in their series, and Meyers and McKeever280 similarly recommended open reduction or arthroscopic treatment for all type III fractures. Smillie362 suggested that closed reduction by hyperextension can be accomplished only with a large fragment.
Operative Treatment of Fracture of the Tibial Spine (Intercondylar Eminence)
Indications/Contraindications
Arthroscopic or open reduction with internal fixation of three tibial eminence fractures and type II which do not reduce has been advocated for a variety of reasons, including concern over meniscal entrapment under the fractured tibial eminence preventing anatomic closed reduction,67,75,119,255 the potential for instability and loss of extension associated with closed reduction and immobilization,151,187,272,311 the ability to evaluate and treat associated intra-articular meniscal or osteochondral injuries with surgery, and the opportunity for early mobilization following fixation. For displaced fractures, Wiley and Baxter408 found a correlation between fracture displacement at healing with knee laxity and functional outcome.
Arthroscopic Reduction and Internal Fixation with Epiphyseal Cannulated Screws
Preoperative Planning (Table 30-2)
TABLE 30-2 Fracture of the Tibial Spine (Intercondylar Eminence)
Technique
General anesthesia is typically used. The patient is positioned supine on the operating room table. A lateral breakaway post is used. Alternatively, a circumferential post can be utilized. A standard arthroscope is used in most patients. A small (2.7 mm) arthroscope is used in younger children. An arthroscopic fluid pump is used at 35 Torr. A tourniquet is routinely used. Standard anteromedial and anterolateral portals are used. Prior to insertion of the arthroscope through the arthroscopic cannula in the anterolateral portal, the large hematoma should be evacuated, and use of up to 2 to 3 L of fluid for repetitive flushing of the joint prior to initiation of the diagnostic arthroscopy should be considered to optimize arthroscopic visualization. An accessory superomedial or superolateral portal can be later developed for guidewire and screw insertion.
A thorough diagnostic arthroscopic examination of the patellofemoral joint, medial compartment, and lateral compartment are essential to evaluate for concomitant injuries. Usually, some anterior fat pad must be excised with an arthroscopic shaver for complete visualization of the intercondylar eminence fragment. Entrapped medial meniscus or intermeniscal ligament is extracted with an arthroscopic probe and retracted with a retention suture (Fig. 30-5). The base of the tibial eminence fragment is elevated (Fig. 30-8A) and the fracture bed debrided with an arthroscopic shaver or hand curette (Fig. 30-8B). Anatomic reduction is obtained using an arthroscopic probe or microfracture pick with the knee in 30 to 90 degrees of flexion (Fig. 30-8C). Cannulated guidewires can be placed through portals just off the superomedial or superolateral border of the patella, using a spinal needle to determine the optimal inferiorly directed vector for fracture fixation and taking care to avoid injury to the chondral surfaces adjacent to the intercondylar notch. The guidewires are placed into the intercondylar eminence at the base of the ACL. Fluoroscopic assistance is utilized to confirm anatomic reduction, to guide correct wire orientation, and to avoid guidewire protrusion across the proximal tibial physis. A cannulated drill is used over the guidewires, taking care to drill the entire depth of the proximal fragment, but avoiding plunging through the proximal tibial physis. One or two screws are placed, based on the size of the tibial eminence fragment (Fig. 30-8D). Partially threaded 3.5-mm diameter screws (Fig. 30-8E) are used in children and either 4- or 4.5-mm diameter screws are used in adolescents. The knee is brought through a range of motion (ROM) to ensure rigid fixation without fracture displacement and to evaluate for impingement of the screw head(s) in extension (Table 30-3).
TABLE 30-3 Fracture of the Tibial Spine (Intercondylar Eminence)
FIGURE 30-8 Arthroscopic reduction and cannulated screw internal fixation of a displaced tibial spine fracture. A: Elevation of the tibial eminence fragment. B: Debridement of the fracture bed. C: Reduction of the tibial eminence. D: Drilling over the cannulated screw guidewire. E: Cannulated screw fixation.
Arthroscopic Reduction and Internal Fixation with Suture
Preoperative Planning (Table 30-4).
TABLE 30-4 Fracture of the Tibial Spine (Intercondylar Eminence)
Technique
Arthroscopic setup and examination is similar to the technique described for epiphyseal screw fixation. Accessory superomedial and superolateral portals are not used, though an accessory transpatellar working portal may be considered to facilitate fracture reduction and suture management. The fracture is elevated (Fig. 30-9A) and the fracture base debrided (Fig. 30-9B). The fracture is reduced, and an optimal reduction may be provisionally maintained with a small K-wire directed inferiorly, though reduction of a previously entrapped intermeniscal ligament or anterior meniscal horn over the anterior aspect of the fragment often maintains the reduction adequately. Two superiorly directed guidewires, approximately 2.7 mm in size, are then placed using the tibial ACL guide system from a small incision made just medial to the tibial tubercle and distal to the proximal tibial physis. Care is taken to create separate starting points for the two guidewires, to ensure a cortical bone bridge for later suture tying, and to place the intra-articular exit points through either side of the base of the intercondylar eminence fragment (Fig. 30-9D) right along the fracture line. Either suture passing devices or small wire loops (smaller than 2.7 mm in diameter) are then placed up the guidewire tracts, either concomitantly or sequentially, to retrieve a suture, which has been passed through the base of the ACL using a suture punch (Fig. 30-9C) or another suture passer. These transtibial suture passers or retrievers then feed the sutures out through the inferior incision (Fig. 30-9E), and the sutures are tied down onto the tibia over the bony bridge (Fig. 30-9F), using arthroscopic assessment to confirm maintenance of the optimal reduction. The procedure is generally repeated for 1 to 2 additional sutures, so as to space the position of the “suture bridge” over a large segment of the ACL footprint and improve rotational stability of the fragment. Resorbable monofilament suture may be used, though we favor heavier nonabsorbable braided sutures, and there are no published reports of complications associated with growth disturbance secondary to the bony bridging or prolonged nonabsorbable suture retention through the small transtibial guidewire tracts (Table 30-5).
TABLE 30-5 Fracture of the Tibial Spine (Intercondylar Eminence)
FIGURE 30-9 Arthroscopic reduction and suture fixation of a displaced tibial spine fracture. A: Elevation of the tibial eminence. B: Debridement of the fracture bed. C: Suture passing through the base of the ACL using a suture punch. D: Drilling of a tibial tunnel into the tibial eminence fragment using the ACL tibial guide. E: Retrieval of sutures using a suture passer. F: Appearance after suture fixation.
AUTHOR’S PREFERRED TREATMENT OF FRACTURE OF THE TIBIAL SPINE (INTERCONDYLAR EMINENCE)
The author’s algorithm to decision making is shown in Figure 30-10. Type I fractures are treated with a locked hinged knee brace in an older child or adolescent or long-leg cast immobilization in a younger child, applied in full extension (0 degrees), to prevent loss of reduction and a flexion contracture, which is generally harder to treat than loss of flexion. The patient and family are cautioned to elevate the leg to avoid swelling. Radiographs are repeated in 1 to 2 weeks to ensure that the fragment has not displaced. The cast is removed 4 to 6 weeks after injury. A hinged knee brace is then used and physical therapy initiated to regain motion and strength. Patients are typically allowed to return to sports 3 to 4 months after injury if they demonstrate fracture healing and adequate motion and strength.
FIGURE 30-10 Algorithm for the management of tibial eminence fractures in children.
Type II fractures are usually treated with attempted closed reduction. Aspiration of hematoma and injection of local anesthetic under sterile conditions may be considered if the patient is in severe pain, but is not required for a successful reduction. Reduction is usually attempted at full extension, with radiographs taken to assess reduction, though if dynamic fluoroscopy is being used, assessment of reduction should also be performed at 20 degrees of flexion and casted in the optimal position. Follow-up radiographs are performed at 1 and 2 weeks postreduction to ensure maintenance of reduction. Length of casting and postcasting management is similar to type I fractures. If the fracture does not reduce anatomically or if the fracture later displaces, operative treatment should be performed to optimize outcomes.
Type III fractures may be treated with attempted closed reduction; however this is usually unsuccessful, and we favor primary operative treatment in the absence of significant medical comorbidities or surgical contraindications. The author’s preferred operative treatment is arthroscopic reduction and internal fixation. However, open reduction through a medial parapatellar incision can also be performed per surgeon preference/experience, or if arthroscopic visualization is difficult. The author’s preferred fixation is epiphyseal cannulated screws if the fragment is large or suture fixation if the fragment is small or comminuted.
Postoperative Care of Fracture of the Tibial Spine (Intercondylar Eminence)
Postoperatively, patients are placed in a postoperative hinged knee brace and maintained touch-down weight bearing for 6 weeks postoperatively. Motion is restricted to 0 to 30 degrees for the first 2 weeks, 0 to 60 degrees for the next 2 weeks, and then 0 to 90 for weeks 4 to 6, with full ROM after 6 weeks, provided early radiographic healing is seen. The brace is kept locked in extension at night for the first 6 weeks to prevent a flexion contracture. Radiographs are obtained at each postoperative visit to evaluate maintenance of reduction and fracture healing (Fig. 30-11). Cast immobilization for 4 weeks postoperatively may be considered in younger children if there is concern for inability to comply with protected weight bearing and brace immobilization. Early initiation of physical therapy is routinely utilized to optimize motion, strength, and sport-specific training. Patients are typically allowed to return to sports at 12 to 16 weeks postoperatively, depending on knee function. Screws are not routinely removed. Functional ACL bracing is utilized if there is residual knee laxity.
FIGURE 30-11 Type III tibial spine fracture in an 11-year-old male child treated with arthroscopic reduction and 3.5-mm cannulated screw fixation. Preoperative AP (A) and lateral (B) radiographs. Postoperative AP (C) and lateral (D) radiographs.
Potential Pitfalls and Preventative Measures of Fracture of the Tibial Spine (Intercondylar Eminence)
In the closed management of tibial eminence fractures, follow-up radiographs must be obtained at 1 and 2 weeks postinjury to verify maintenance of reduction. Late displacement and malunion can occur, particularly for type II fractures. Though generally not necessary, aspiration of hemarthrosis and injection of local anesthetic under sterile conditions can occasionally be helpful to minimize pain and allow for full knee extension at the time of closed reduction.
During arthroscopic reduction and fixation of tibial spine fractures, arthroscopic visualization can be difficult unless the large hematoma is evacuated and flushed prior to introduction of the arthroscope. Adequate inflow and outflow is essential for proper visualization. Careful attention to preparation of the fracture bed is important to provide optimal conditions for bony healing. Attempted epiphyseal cannulated screw fixation of small or comminuted tibial eminence fragments can fail as the screw may further comminute the fragment. In these cases, suture fixation is generally a better method. If epiphyseal cannulated screw fixation is used, fluoroscopy is necessary to ensure that the drill or screw does not traverse the proximal tibial physis, which may result in a proximal tibial physeal growth arrest.
Early mobilization is helpful to avoid arthrofibrosis which can occur with immobilization. However, in younger children (less than 7 years old), compliance with protected weight bearing and brace use can be problematic (Table 30-6).
TABLE 30-6 Fracture of the Tibial Spine (Intercondylar Eminence)
Treatment-Specific Outcomes for Fracture of the Tibial Spine (Intercondylar Eminence)
The prognosis for closed treatment of nondisplaced and reduced tibial spine fractures and for operative treatment of displaced fractures is good. Most series report healing with an excellent functional outcome despite some residual knee laxity.36,39,46,196,215,240,255,256,271,272,286,289,365,387,408,410 Potential complications include nonunion, malunion, arthrofibrosis, residual knee laxity, and growth disturbance.36,39,46,196,215,240,255,256,271,272,286,289,365,391,408,410
Mild residual knee laxity is seen frequently, even after anatomic reduction and healing of tibial eminence fractures. Baxter and Wiley39,408 found excellent functional results without symptomatic instability in 17 pediatric knees with displaced tibial spine fractures, despite a positive Lachman examination in 51% of patients and increased mean instrumented knee laxity of 3.5 mm. After ORIF of type III fractures in 13 pediatric knees, Smith365 identified patient-reported instability in only two patients, despite a positive Lachman examination in 87% of patients. In a group of 50 children after closed or open treatment, Willis et al.410 found excellent clinical results despite a positive Lachman examination in 64% of patients and instrumented knee laxity of 3.5 mm for type II fractures and 4.5 mm for type III fractures. Similarly, Janarv et al.187 and Kocher et al.215 found excellent functional results despite persistent laxity even in anatomically healed fractures. More recent long-term follow-up studies have replicated these findings.72,387 Despite four patients demonstrating signs, but no symptoms, of instability, Tudisco et al.387 recently reported good results in 13 of 14 knees followed for a mean of 29 years postinjury, with the one suboptimal result reported in a type III fracture treated nonoperatively.
Persistent laxity despite anatomic reduction and healing of tibial spine fractures in children is likely related to plastic deformation of the collagenous fibers of the ACL occurring in association with tibial spine fracture. At the time of tibial spine fixation, the ACL often appears hemorrhagic within its sheath, but grossly intact and in continuity. In a primate animal model, Noyes et al.305 found frequent elongation and disruption of ligament architecture despite gross ligament continuity in experimentally produced tibial spine fractures at both slow and fast loading rates. This persistent anteroposterior laxity despite anatomic reduction may be avoided by countersinking the tibial spine fragment within the epiphysis at the time of reduction and fixation. However, ACL injury after previous tibial spine fracture is rare.
MANAGEMENT OF EXPECTED ADVERSE OUTCOMES AND UNEXPECTED COMPLICATIONS IN FRACTURE OF THE TIBIAL SPINE (INTERCONDYLAR EMINENCE)
Poor results may occur after eminence fractures associated with unrecognized injuries of the collateral ligaments or complications from associated physeal fracture.278,365,376 In addition, hardware across the proximal tibial physis may result in growth disturbance with recurvatum deformity or shortening.290
Malunion of type II and III fractures may cause mechanical impingement of the knee during full extension (Fig. 30-12).132,255,256 For symptomatic patients, this can be corrected by either osteotomy of the fragment and fixation in a more recessed, anatomic position or excision of the manumitted fragment with anatomic suture repair of the ACL to its bony footprint. Alternatively, excision of the fragment and ACL reconstruction can be considered in adults and older adolescents.
FIGURE 30-12 Lateral radiograph of a malunited displaced fracture of the intercondylar eminence of the tibia with an extension block.
Nonunion of type II and III tibial spine fractures treated closed can usually be managed by arthroscopic or open reduction with internal fixation.204,247,394 Technically, debridement of the fracture bed and the fracture fragment to fresh, bleeding bone is essential to optimize bony healing. Bone graft may be required in cases of chronic nonunion. Similarly to management of malunions described above, excision of the fragment and ACL reconstruction can alternatively be considered in adults and older adolescents, and may be preferable, given the increasingly favorable reports of outcomes of pediatric ACL reconstruction techniques.
Stiffness and arthrofibrosis can be a challenging problem after both nonoperative and operative management of tibial eminence fractures. The milieu of a major traumatic intra-articular injury, a large hemarthrosis, and immobilization can predispose to arthrofibrosis. Vander Have et al. reported on 20 cases of arthrofibrosis out of 205 patients (10%) from four institutions over a 10-year period who had undergone surgical intervention for tibial spine fracture, as well as 12 additional cases referred from other institutions. Of the 32 total cases, 25 (78%) had been immobilized for 4 to 6 weeks postoperatively without motion, and 24 (75%) required additional operative treatment within 6 months to address debilitating loss of knee motion.391 The authors concluded that for fractures that undergo fixation, early mobilization utilizing physical therapy can minimize the risk of arthrofibrosis, an approach supported by subsequent analyses.318 If stiffness is detected, dynamic splinting and aggressive physical therapy can be employed during the first 3 months from fracture. If significant stiffness remains after 3 months from fracture, patients should be managed with manipulation under anesthesia, but only in conjunction with arthroscopic lyses of adhesions, an approach shown to be successful in resolving the stiffness in majority of cases.391 Overly vigorous manipulation should be avoided to avert iatrogenic proximal tibial or distal femoral physeal fracture, which may lead to growth arrest or deformity requiring further treatment (Table 30-7).391
TABLE 30-7 Fracture of the Tibial Spine (Intercondylar Eminence)
INTRODUCTION TO OSTEOCHONDRAL FRACTURES
Osteochondral fractures in skeletally immature patients are more common than once thought. They are typically associated with acute lateral patellar dislocations. The most common locations for these fractures are the inferior aspect of the patellar median ridge, the inferior medial patellar facet, or the lateral aspect of the LFC (Fig. 30-13). The osteochondral fracture fragments may range from small incidental loose bodies to large portions of the articular surface. The prevalence of osteochondral fractures associated with acute patella dislocation ranges from 19% to 50%.15,54,120,266,297,302,371 Matelic et al.266 found 67% of one series of children presenting with an acute hemarthrosis of the knee were found to have an osteochondral fracture.
FIGURE 30-13 Osteochondral fractures associated with dislocation of the right patella. A: Medial facet. B: Lateral femoral condyle.
The diagnosis can be difficult to make because even a large osteochondral fragment may contain only a small ossified portion that is visible on plain radiographs. MRI has therefore emerged as having a critical role in identifying associated osteochondral fractures or chondral-only fragments in cases of traumatic patellar dislocation. Acute osteochondral fractures must be differentiated from acute chondral injuries, which do not involve subchondral bone, and osteochondritis dissecans (OCD),124,221 which is most often a repetitive overuse lesion of the subchondral bone, which may result in a nonhealing stress fracture that can progress to fragment dissection.
Treatment of osteochondral fractures includes removal of small loose bodies and fixation of larger osteochondral fragments. In cases associated with patellar dislocation, lateral retinacular release, medial retinacular repair, medial patellofemoral ligament (MPFL) repair, or primary reconstruction may be performed adjunctively.
ASSESSMENT OF OSTEOCHONDRAL FRACTURES
Mechanisms of Injury for Osteochondral Fractures
There are two primary mechanisms for production of an osteochondral fracture.15,54,,81,120,122,201,266,273,297,371 First, a direct blow to the knee with a shearing force applied to either the medial or LFC can create an osteochondral fracture. The second mechanism involves a flexion-rotation injury of the knee in which an internal rotation force is placed on a fixed foot, usually coupled with a strong quadriceps contraction. The subsequent contact between the tibia and femur or patella and LFC causes the fracture. This latter contact mechanism occurs during an acute patellar dislocation. When the patella dislocates laterally, the medial retinaculum and the associated medial MPFL tears, while the extensor mechanism still applies significant compressive forces as the patella shears across the LFC. The medial border of the patella then temporarily becomes impacted on the prominent edge of the LFC before it slides back tangentially over the surface of the LFC because of pull of the quadriceps. Either the dislocation or the relocation phase of this injury can cause an osteochondral fracture to the LFC, the medial facet of the patella, or both (Fig. 30-14). Interestingly, osteochondral fractures are uncommon with chronic, recurrent subluxation, or dislocation of the patella. In this situation, the laxity of the medial knee tissues and decreased compressive forces between the patella and the LFC prevent development of excessive shear forces. With more acute or traumatic dislocations, even if a frank osteochondral fracture does not occur, bone bruising is generally seen on MRI on both the patella and LFC, and chondral injuries, such as fissuring of the articular surface of the medial facet and median ridge, are also common.116,203,300,302
FIGURE 30-14 Osteochondral fractures associated with dislocation of the patella. A: Medial facet of patella. B: Lateral femoral condyle.
Ahstrom10 reported on a series of 18 osteochondral fractures, 14 of which occurred during sports-related activities. Most patients give a history of a twisting injury consistent with acute patellar dislocation, but a few report a direct blow to the lateral or medial femoral condyle, accounting for a shear injury. The prevalence of osteochondral fractures associated with acute patella dislocation ranges from 19% to 50% in the literature.15,54,120,266,297,302,371 Nietosvaara et al.297 reported that of 69 acute patellar dislocations in children and adolescents, 62 (90%) of which occurred during or as a result of a fall, 39% had osteochondral fractures.
Associated Injuries with Osteochondral Fractures
As described above, common injuries associated with osteochondral fractures caused by patellar dislocation include MPFL tear and bone bruises or impaction injuries to the LFC and medial aspect of the patella. Other osteochondral fractures may occur in association with severe cruciate or collateral ligament tears, as well as knee dislocation.
Signs and Symptoms of Osteochondral Fractures
Acutely, osteochondral fractures present with severe pain, swelling, and difficulty in weight bearing.2,10,11,32,38,45,81,82,109,142,166,176,177,189,201,242,273,297,308,334,335,348,362,368,411,412 On examination, tenderness to palpation is often most severe over the medial patella and lateral aspect of the LFC, though medial femoral condylar tenderness may also be exhibited, either from a femoral-sided tear of the MPFL from the adductor tubercle region or because of a partial MCL sprain, which is not uncommon in association with patellar dislocation. The patient will usually resist attempts to flex or extend the knee and may hold the knee in 15 to 20 degrees of flexion for comfort. The large hemarthrosis is due to an intra-articular fracture of the highly vascular subchondral bone. Joint aspiration may reveal fatty globules or a supernatant layer of fat if allowed to stand for 15 minutes indicating an intra-articular fracture. Similarly, fluid–fluid levels may be seen on MRI, from the separation of fat and blood. Late examination findings may be similar to those of a loose body with intermittent locking or catching of the knee.
Imaging and Other Diagnostic Studies for Osteochondral Fractures
Radiographic assessment of a possible osteochondral fracture should begin with anteroposterior, lateral, and skyline plain radiographs. However, a roentgenographic diagnosis can be difficult because even a large osteochondral fragment may contain only a small ossified portion that is visible on plain radiographs. A tunnel view may help locate a fragment in the region of the intercondylar notch. Because the osteochondral fragment may be difficult to see on plain radiographs, radiographs should be carefully assessed for even the smallest ossified fragment (Fig. 30-15).
FIGURE 30-15 Osteochondral fracture of lateral femoral condyle after patellar dislocation. A: Fragment seen in lateral joint space. B: Lateral view.
Matelic et al.266 report that standard radiographs failed to identify the osteochondral fracture in 36% of children who had an osteochondral fracture found during arthroscopy. For this reason, MRI is recommended in most cases, due to the importance of identifying a possible osteochondral fracture despite negative radiographs60,214,406 or a large chondral fragment. Such cases usually occur in the setting of an acute traumatic patellar dislocation in a patient with a large hemarthrosis, whereas ligamentously lax patients with chronic, recurrent, atraumatic patellar instability are less likely to sustain osteochondral fractures. A high-riding patella may also have a protective effect against associated intra-articular osteochondral fractures. Patients with an Insall index > 1.3 have a decreased chance of sustaining an osteochondral fracture compared with patients who have an Insall index within normal limits.60 An arthrogram effect is usually present during MRI, given the large hemarthrosis.
Classification of Osteochondral Fractures
The classification of osteochondral fractures of the knee is based on the site, the type, and the mechanism of injury. The classification outlined in Table 30-8 is based on the descriptions of osteochondral fractures by Kennedy201 and Smillie.362
TABLE 30-8 Mechanism of Osteochondral Fractures
Outcome Measures for Osteochondral Fractures
Healing of osteochondral fractures must be followed closely with radiographs, as healing is the most important predictor of outcome. Once healed, standard outcome measures, such as functional knee metrics (the Pedi-IKDC225 and Lysholm364 knee scores), can be used to assess the results and, paired with the Marx or Tegner activity scores,264 ascertain a patient’s ability to make a full return to activities of daily life and sports activities.
PATHOANATOMY AND APPLIED ANATOMY RELATING TO OSTEOCHONDRAL FRACTURES
The patella tracks in the trochlear groove between the medial and LFCs during flexion and extension of the knee.142,177 With increasing knee flexion, the contact area on the articular surface of the patella moves from the distal to the proximal aspect of the articular surface of the patella. Between 90 and 135 degrees of flexion, the patella glides into the intercondylar notch between the femoral condyles. The two primary areas of contact are the medial patellar facet with the medial femoral condyle and the superolateral quadrant of the lateral patellar facet with the LFC. Soft tissue support for the patellofemoral joint includes the quadriceps muscle, the MPFL, the patellar tendon, and the vastus medialis and lateralis muscles.
Dislocation of the patella may tear the medial retinaculum and MPFL, but the rest of the quadriceps muscle–patellar ligament complex continues to apply significant compression forces as the patella dislocates laterally. These forces are believed to cause fracture of the medial patellar facet, the LFC articular rim, or both (Fig. 30-13).200,201,305,306,308,332,333,335 Osteochondral fractures are uncommon with chronic recurrent subluxation or dislocation of the patella because of relative laxity of the medial retinaculum and lesser compressive forces on the patella and the LFC.
A histopathologic study by Flachsmann et al.122 helps to explain the occurrence of osteochondral fractures in the skeletally immature at an ultrastructural level. They noted that in the joint of a juvenile, interdigitating fingers of uncalcified cartilage penetrate deep into the subchondral bone providing a relatively strong bond between the articular cartilage and the subchondral bone. In the adult, the articular cartilage is bonded to the subchondral bone by the well-defined calcified cartilage layer, the cement line. When shear stress is applied to the juvenile joint, the forces are transmitted into the subchondral bone by the interdigitating cartilage with the resultant bending forces causing the open pore structure of the trabecular bone to fail. In mature tissue, the plane of failure occurs between the deep and calcified layers of the cartilage, the tidemark, leaving the osteochondral junction undisturbed. Although the juvenile and adult tissue patterns are different, they both provide adequate fracture toughness to the osteochondral region. As the tissue transitions, however, from the juvenile to the adult pattern during adolescence, the fracture toughness is lost. The calcified cartilage layer is only partially formed and the interdigitating cartilage fingers are progressively replaced with calcified matrix. Consequently, the interface between the articular cartilage and the subchondral bone becomes a zone of potential weakness in the joint which may explain why osteochondral fractures are seen frequently in adolescents and young adults.
TREATMENT OPTIONS FOR OSTEOCHONDRAL FRACTURES
Nonoperative Treatment of Osteochondral Fractures
Nonoperative treatment of osteochondral fractures is reserved for small fragments, 5 mm or less, that have failed to cause or are unlikely to cause symptoms associated with loose body fragments. Every osteochondral fracture and the injury through which it occurred are different, but treatment should be individualized, to some degree, based on a patient’s age and activity level. However, as general principles, the larger the fragment, the more bone attached a given fragment, and the more central the weight-bearing zone from which the fragment was detached, the more consideration should be given to attempted refixation.
Operative Treatment of Osteochondral Fractures
Indications/Contraindications and Surgical Procedure
The recommended management of acute osteochondral fractures of the knee is either surgical removal of the fragment or fixation of the fragment, depending on its size and the quality of the tissue.213,220 In patients with an osteochondral fracture after acute patellar dislocation, concomitant repair of the medial retinaculum and MPFL, either at the patellar or femoral insertion sites of the ligament, or through an intrasubstance imbrications, at the time of fragment excision or fixation, may decrease the risk of recurrent patellar instability,7,70,82,334 though there is conflicting evidence whether this repair improves the ultimate redislocation rate.78,298,299,314
Fixation
If the lesion is large (≥5 mm), easily accessible, involves a weight-bearing area, and has adequate cortical bone attached to the chondral surface then fixation should be considered.45,201,362,368,411,412
Preoperative Planning (Table 30-9)
TABLE 30-9 Fixation of Osteochondral Fractures
Surgical Approach and Technique
Fixation can be performed via arthroscopy or arthrotomy. Fixation options include K-wires, Steinmann pins, cannulated or solid metal screws, variable pitch headless screws, or bioabsorbable pins,76,138,401 tacks, or screws, which have recently increased in popularity and have the advantage of not requiring implant removal.103 For nonbioabsorbable implants, hardware removal is typically performed after fracture healing, though headless compression screws may be buried beneath the superficial level of the cartilage and may be retained.242 Traditionally, fixation of chondral fragments with no bone attached was not considered amenable to refixation, because of concerns regarding poor healing capacity. However, new reports have suggested that large chondral-only fragments may be able to heal in children or adolescents if early refixation is pursued (Table 30-10).291,388
TABLE 30-10 Fixation of Osteochondral Fractures
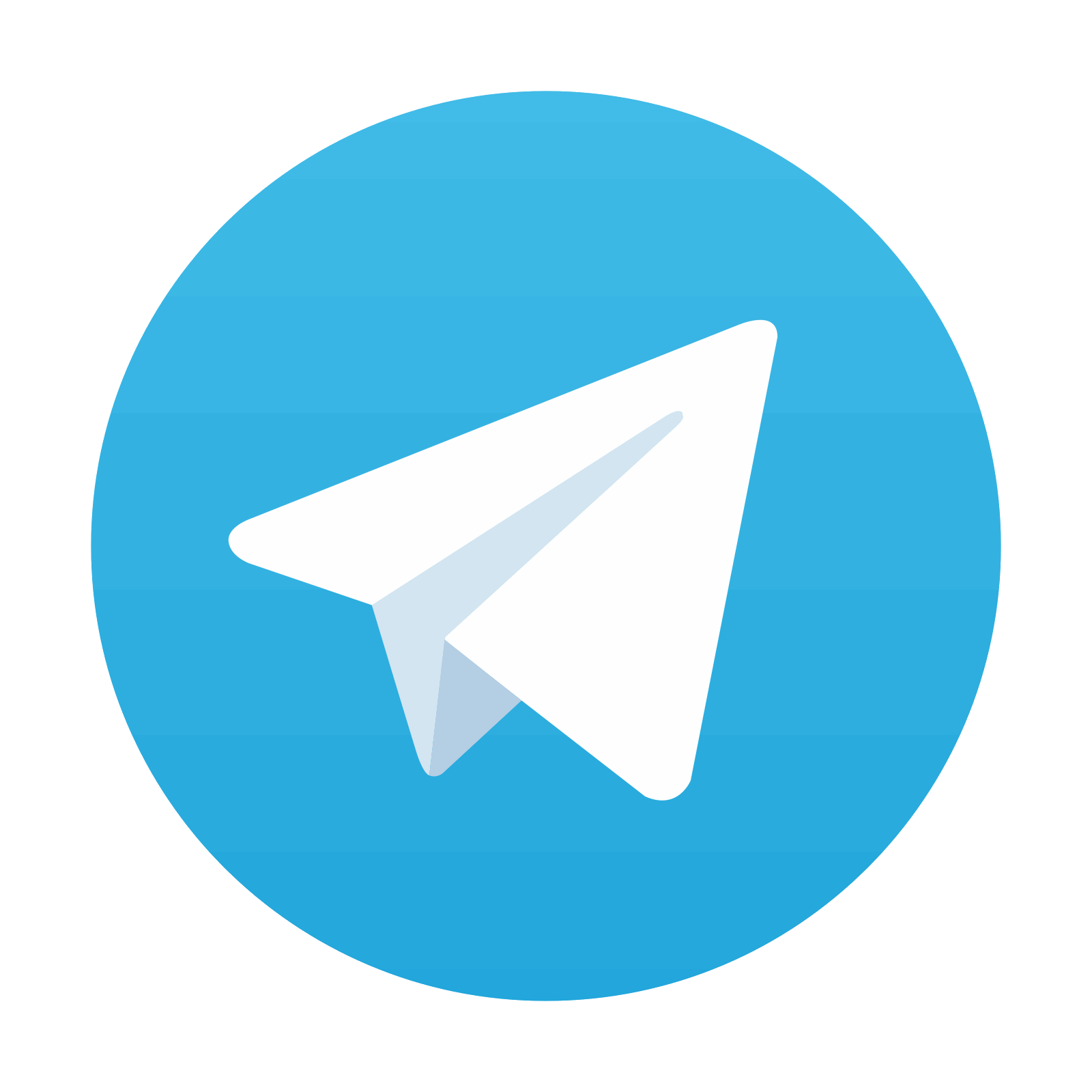
Stay updated, free articles. Join our Telegram channel

Full access? Get Clinical Tree
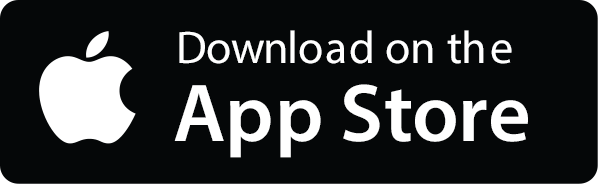
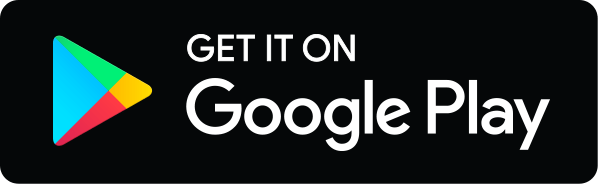