Major risk factors
Minor risk factors
Vertebral compression fracture
Rheumatoid arthritis
Fragility fracture after age 40
Past history of hyperthyroidism
Family history of osteoporotic fracture
Chronic anticonvulsant therapy
Systemic glucocorticoid therapy >3 months
Low dietary calcium intake
Malabsorption syndrome
Smoking
Primary hyperparathyroidism
Excessive alcohol intake
Propensity to fall
Excessive caffeine intake
Osteopenia apparent on X-ray film
Weight <57 kg
Hypogonadism
Weight loss >10 % of weight at age 25
Early menopause (before age 45)
Chronic heparin therapy
In adults, DEXA is interpreted in terms of T-score. By the World Health Organization’s reference values, a T-score refers to how many standard deviations a patients’ bone density lies from the average bone density of a 20- to 29-year-old female. A T-score of ≤–2.5 at the femoral neck, total hip, or lumbar spine generally allows for the diagnosis of osteoporosis [8]. In contrast to the T-score, the Z-score is based on standard deviations from an age, gender, and race matched reference value. Z-scores are used for children and adolescents undergoing DXA testing [9].
In premenopausal women, treatment for early osteopenia/osteoporosis includes investigation for underlying causes as well as ensuring adequate intake of calories, calcium (1,000 mg daily from diet), and vitamin D (600 IU D3 supplement) [10]. For premenopausal women, evidence is lacking for common pharmacologic treatments used in postmenopausal women such as bisphosphonates, selective estrogen receptor modulators, teriparatide, and denosumab. Smoking cessation [11], normalization of body weight, avoiding excessive dieting and weight swings [12], and limiting alcohol consumption [13] have been shown to be of benefit in preventing or treating decreased bone mineral density.
In postmenopausal women initial osteoporosis treatment begins with ensuring adequate vitamin D and calcium intake. Along with dietary calcium, supplemental calcium should be taken in doses of 500–1,000 mg/day so that total calcium intake equals around 1,200 mg/day. Intake of vitamin D should equal around 800 IU daily. Exercise and cessation of smoking are also important in this population. The National Osteoporosis Foundation recommends pharmacologic intervention in postmenopausal women with a history of hip fracture or vertebral compression fracture or in those individuals with a T-score ≤–2.5 [14]. Bisphosphonates are considered the mainstay of pharmacologic intervention in postmenopausal osteoporosis [15]. Other effective agents include selective estrogen receptor modulators [16], parathyroid hormone [17], and denosumab [18, 19]. Evidence is lacking or contraindications exist for estrogen replacement, calcitonin, and combination therapy.
Treatment guidelines also exist for the treatment of osteoporosis in men. As with women, diet and lifestyle changes are important in the treatment of osteoporosis. Calcium intake should reach 1,000 mg/day in younger men and up to 1,200 mg/day in older men. Vitamin D supplementation should equal 600–800 IU per day. Continued exercise, smoking cessation, and limiting alcohol consumption are also important lifestyle changes in men. Testosterone therapy is recommended in younger hypogonadal men with no contraindications to testosterone therapy [20]. In addition to the above measures, pharmacologic intervention is recommended in men with a history of fracture or T-score of ≤–2.5. Pharmacologic intervention may also be necessary in men with a T-score of –1.0 to –2.5. As with women, bisphosphonate therapy is oftentimes the drug of choice. Studies have recommended weekly treatment with alendronate or risedronate [21]. Other alternatives include the IV bisphosphonate zoledronic acid, and secondline agents such as teriparatide [22] or denosumab [23].
The Female Athlete Triad and Decreased Bone Mineral Density
Young athletes particularly vulnerable to insufficiency facture are those with the female athlete triad of amenorrhea, eating disorder, and osteoporosis. This triad is specifically observed in physically active females and is now defined as involvement of any one or more of the following components: (1) low energy availability with or without disordered eating; (2) menstrual dysfunction; and (3) low bone mineral density [24]. Risk factors for the triad that should be assessed in female athletes are menstrual irregularities, criticism of eating habits by coach, family, or peers, depression, dieting, obsessive personality, pressure to lose weight, early sport-specific training, overtraining, recurrent injuries, history of fracture, low BMI, and physical examination signs of an eating disorder [24]. In relation to triad risk factors, a 2014 study found that more triad risk factors are associated with a greater odds of bone stress injury than one factor alone [25]. Specifically, the authors found an increase in bone stress injury from 15 to 21 % for one risk factor to 30 % for two risk factors to 50 % for three triad risk factors [25]. Another 2014 study also found that multiple risk factors exhibit a cumulative risk of lower bone mineral density in young women [26].
Low energy availability in at-risk athletes often leads to menstrual dysfunction and can lead to deleterious effects on the musculoskeletal system. In cases of hypoestrogenism, increased reabsorption of calcium and decreased bone storage of calcium leads to decreased bone mineral density [27]. In terms of menstrual irregularities and bone mineral density, a 2003 study found that female runners experiencing less than 10 menstrual cycles per year had bone mineral densities 3–6 % lower than those female runners having greater than 10 menstrual cycles per year [28]. In addition to the musculoskeletal system, the reproductive, cardiovascular, endocrine, gastrointestinal, renal and neurological systems can be affected by the female athlete triad [24].
Diagnosis of the female athlete triad is multifaceted and involves a multidisciplinary approach. Low energy availability can be indicated by a BMI <17.5 kg/m2 or in adolescents <85 % of expected body weight. While a low BMI can be an indicator of low energy availability assessing energy availability is most often a much more complex measurement. In response to this issue the Female Athlete Triad Coalition provides an energy availability calculator on their website (http://www.femaleathletetriad.org/calculators/). They report that physically active women should aim for at least 45 kcal/kg fat-free mass/day of energy intake [24].
Assessing amenorrhea is a complex process that should be initialized by the primary care physician with appropriate consults to both gynecology and endocrinology specialists. Pregnancy and endocrine disorders such as thyroid dysfunction, hyperprolactinemia, primary ovarian insufficiency, hypothalamic and pituitary disorders, and hyperandrogenic conditions must be ruled out as the causes of the amenorrhea.
Because low bone mineral density is the direct contributing factor to an insufficiency fracture, criteria have been established for obtaining DEXA testing in young woman and girls. The Female Athlete Triad Coalition recommends DEXA testing in athletes with one or more high-risk factors or two or more moderate risk factors (Table 16.2). The Coalition also recommends DEXA testing in athletes with a history of two or more peripheral long bone traumatic fractures when 1 or more high or moderate triad risk factors are identified [24]. Results from DEXA scanning should be interpreted carefully and may need to be repeated every 1–2 years in individuals with ongoing indications for testing. The International Society for Clinical Densitometry (ISCD) provides guidelines for interpreting DEXA testing in children and adolescents. In their 2013 position statement the ISCD maintained that a vertebral compression fracture is indicative of osteoporosis in children and adolescents while densitometry alone is not adequate to diagnose osteoporosis. Total body less head and the posterior–anterior spine are the preferred skeletal areas when performing DEXA testing. In children and adolescents without vertebral compression fracture, osteoporosis is diagnosed with a significant fracture history and a Z-score of ≤–2.0 on densitometry. The ISCD also reports that a Z-score of >–2.0 does not necessarily preclude skeletal fragility [29, 30]. Young individuals with osteoporosis most often present with fracture before the diagnosis is confirmed; therefore early recognition and prevention of the female athlete triad is instrumental in avoiding insufficiency fractures.
High risk | Moderate risk |
---|---|
• History of a DSM-V diagnosed eating disorder | • Current or history of disordered eating for ≥6 months |
• BMI ≤17.5 kg/m2, <85 % estimated weight, or recent weight loss of ≥10 % in 1 month | • BMI between 17.5 and 18.5, <90 % estimated weight, or recent weight loss of 5–10 % in 1 month |
• Menarche at ≥16 years of age | • Menarche between 15 and 16 years of age |
• Current or history of <6 menses over 12 months | • Current or history of 6–8 menses over 12 months |
• Two prior stress fractures, 1 high-risk stress fracture, or a low energy nontraumatic fracture | • One prior stress reaction or stress fracture |
• Prior Z-score of <–2.0 (after >1 year from baseline DXA) | • Prior Z-score between –1.0 and –2.0 (after >1 year from baseline DXA) |
Osteomalacia/Rickets
Osteomalacia, termed rickets in children, is defective bone mineralization most often caused by a chronic deficiency in vitamin D or phosphate. Consequently, individuals with osteomalacia have a softening of bones which predisposes to fracture. While it is extremely rare in athletes, it should be considered as an underlying cause of insufficiency fracture in athletes with generalized osteopenia. A 2013 study found that DEXA scanning may detect osteoporosis in up to 70 % of individuals with osteomalacia [31]. Patients with osteomalacia often present with generalized bone pain and osteopenia. Generally the best way to prevent osteomalacia induced insufficiency fracture is ensuring adequate vitamin D intake throughout life. In a 2013 position statement, The Society for Adolescent Health and Medicine recommended vitamin D supplementation of 600 IU daily in healthy adolescents and at least 1,000 IU for adolescents at risk for vitamin D insufficiency, in addition to dietary intake. Other guidelines for vitamin D supplementation and management for adolescents are presented in Table 16.3. Some conditions that are potential factors associated with vitamin D deficiency are increased skin pigmentation, frequent use of sunscreen, obesity, specific diets such as vegan, cultural body coverage requirements, chronic GI diseases, amenorrhea, pregnancy or lactation, immobilization, bariatric surgery, chronic kidney or liver disease, certain medications such as steroids, anticonvulsants, and HIV medications, and known low bone density status [32]. In older adults the International Osteoporosis Foundation (IOF) states that, on average, 800–1,000 IU of vitamin D are required per day to maintain a serum level of 25-hydroxyvitamin D (25(OH)D) of 30 ng/mL. The required intake also varies per individual as 800 IU per day may be sufficient in healthy individuals with regular sun exposure. On the other hand, obese individuals, those with low sun exposure, with osteoporosis, malabsorption, and in populations such as those of Middle Eastern or Southern Asian decent may need upward of 2,000 IU of vitamin D intake per day [33]. The IOF advises that 100 IU of vitamin D will increase the serum 25(OH)D by about 1.0 ng/mL.
Table 16.3
Vitamin D guidelines as recommended by the Society of Adolescent Health and Medicine [32]
• Vitamin D supplementation of 600 IU daily in healthy adolescents |
• Vitamin D supplementation of at least 1,000 IU daily for adolescents at risk for vitamin D insufficiency |
• Serum 25(OH)D concentration in at-risk adolescents |
• Serum 25(OH)D concentration of 30–50 ng/mL is optimal in adolescents |
• In adolescents with <20 ng/mL 25(OH)D, supplement 50,000 IU of vitamin D once per week for 8 weeks |
• In adolescents with 20–29 ng/mL 25(OH)D, supplement 1,000 IU per day for at least 3 months |
• Use a vitamin D3 preparation if available |
• Vitamin D supplementation should be taken with dinner if possible |
Paget’s Disease of Bone
Paget’s disease of bone is generally a disease of older individuals and is characterized by abnormal bony remodeling and resultant disorganized bony architecture. The disorganized bone growth associated with Paget’s disease of bone can initially lie clinically silent and may lead to bone pain, bone deformity, fracture, osteoarthritis, spinal stenosis, cranial nerve compression, tinnitus, deafness, and in a small number, osteosarcoma. Paget’s disease of bone is thought to have genetic influences and potentially environmental triggers such as viral infection and low calcium and vitamin D intake. The most common bones affected by Paget’s disease of bone are the pelvis, femur, lumbar spine, skull, and tibia [34, 35]. Patients with Paget’s disease of bone often have normal calcium, phosphate, and PTH levels on laboratory testing. Variable but often elevated levels of alkaline phosphatase may be observed and depend on the stage of the disease [35]. Another potential presentation finding of Paget’s disease of bone is an abnormal radiograph while investigating for other pathologies. Pseudofractures on the convex aspects of affected bones also should raise suspicion of Paget’s disease of bone [35]. Patients may also report pain with use of the affected area, with rest, and at night [35]. Other factors useful in diagnosing Paget’s disease of bone are localized pain in areas with continued uptake on bone scan and pain improvement with a bisphosphonate trial. Pain that originates in joints is less likely to be due to Paget’s disease of bone. While Paget’s disease of bone is an extremely rare cause of insufficiency fracture in younger individuals, it should be ruled out as a potential underlying cause of insufficiency fracture in aging athletes as treatment of Paget’s disease involves medical and surgical management.
Radiation-Induced Fractures
While fracture associated with tumor is termed pathological fracture, insufficiency fracture can occur in previously irradiated bone in which a tumor has since resolved. Ionizing radiation is effective as a treatment means for cancer because it causes cell death through DNA strand breaks. This radiation disrupts the bone’s blood supply and decreases the number of osteoblasts while increasing the activity and number of osteoclasts. This leads to bone marrow suppression and abnormal bony remodeling, which in effect lowers bone mass and density predisposing affected individuals to insufficiency fracture. These effects are dose dependent and can remain permanent with higher radiation dosages. Therefore, athletes with a history of radiation therapy must be monitored for the development of insufficiency fractures [36].
Presentation of Insufficiency Fractures
Patients with insufficiency fractures often present with acute pain in a commonly affected area such as the back, groin, or foot. A history of trauma is usually lacking. Depending on the severity of the fracture, the patient may present in a non-ambulatory state. Physical examination of a suspected insufficiency fracture involves localization of pain and inspection of the area for warmth and swelling and palpation for tenderness. Range of motion, the fulcrum test, flexion–abduction–external rotation (FABER test), and Flamingo test may assist in evaluation of areas not readily accessible to direct palpation [3].
Imaging of Insufficiency Fractures
Obtaining proper imaging is instrumental in early recognition and treatment of suspected insufficiency fractures. As with any suspected skeletal injury, plain radiographs should be obtained. Plain radiographs may assist in the diagnosis of various insufficiency fractures though magnetic resonance imaging (MRI), computed tomography (CT), or bone scintigraphy may be necessary in cases where plain radiographs are inconclusive and a patient’s pain persists.
Radiography
The most common radiographic imaging finding in patients with insufficiency fracture is a sclerotic band in the affected bone. Other imaging findings on radiography of insufficiency fractures include bone resorption along the fracture line, bony expansion, callus, and osteolysis. Radiography is often more successful in detecting insufficiency fractures in long bones, pubic rami, and peripheral bones while sacral and pelvic insufficiency fractures are more often elusive on standard radiographs [37].
Multidetector CT Scanning
Multidetector CT (MDCT) scanning may be the imaging modality of choice in the detection of insufficiency fractures though the high dose of ionizing radiation limits its usage to some extent. MDCT allows for thin slices and detection of otherwise non-visible fracture lines. Callus development can also be readily observed. While bony edema on MRI can be suggestive of fracture as well as other processes such as tumor, high resolution CT scanning has the ability to rule out lytic lesions and those extending into the adjacent soft tissue [37].
Magnetic Resonance Imaging
Due to the absence of ionizing radiation, MRI is commonly used as the imaging modality of choice in the diagnosis of insufficiency fracture when plain radiographs prove inconclusive. Hypointense signal to adjacent bone is seen on T1 weighted images while hyperintense signal is observed on T2 weighted images with the possibility of observing the fracture line within bony edema. A commonly cited radiographic and MR grading system of stress fractures can be found in Table 16.4 [38]. Care must be taken as to not confuse an insufficiency fracture with underlying avascular necrosis or tumor.
Table 16.4
Radiographic grading of stress fracturesa
Grade | Radiograph findings | MRI findings |
---|---|---|
Normal | Normal | Normal |
1 | Normal | Positive STIR image |
2 | Normal | Positive STIR, plus positive T2-weighted |
3 | Periosteal reaction | Positive T1 and T2 weighted, STIR without definite cortical break visualized |
4 | Injury or periosteal reaction | Positive injury line on T1 or T2 weighted scans |
Bone Scintigraphy
Bone scintigraphy, also termed radionucleotide scanning or bone scanning is very sensitive for insufficiency fractures though generally it is nonspecific. Various uptake patterns can be difficult to interpret and can remain positive well after a fracture occurs. Bone scintigraphy has clinical utility in the diagnosis of insufficiency fractures of the sacrum and pelvis as two or more areas of increased uptake in the sacrum and another in a pelvic site are diagnostic of insufficiency fractures [37]. Grading of stress fractures on bone scans has been proposed as grade 1, a small, ill-defined cortical area of mildly increased activity; grade 2, larger well-defined cortical area of moderately increased activity; grade 3, wide to fusiform involvement, cortical-medullary area of highly increased activity; and grade 4, transcortical area of intensely increased activity [39]. This grading system has also been correlated to MRI findings as Grade 1, mild to moderate periosteal edema on T2 with normal marrow; grade 2, moderate to severe periosteal edema on T2 with marrow edema on T2; grade 3, moderate to severe periosteal edema on T2 with marrow edema on T1 and T2; and grade 4, moderate to severe periosteal edema on T2 with a clearly visible fracture line and marrow edema on T1 and T2 images [40]. It should be noted that a more recent study made note that periosteal edema is often observed on MR but not always present in a bone stress injury [41].
Specific Sites of Insufficiency Fractures and Management
Pelvis
Pelvic insufficiency fractures are more often seen in elderly individuals with osteoporosis though any condition that leads to premature osteoporosis can predispose to the development of an insufficiency fracture. Pelvic insufficiency fractures are also associated with a history of radiation therapy in postmenopausal women. In younger women, a pelvic insufficiency fracture is most often observed in the inferior pubic rami [42]. Insufficiency fractures in the pelvis can often present as low back pain making the proper diagnosis more difficult to determine. Because plain radiographs oftentimes do not visualize these fractures with certainty, MRI of the pelvis is often necessary to confirm the diagnosis. Patients may have previously had an extensive lower back workup with the causative fracture escaping previous imaging attempts. In a 2008 study of MRI and CT imaging of 145 patients with pelvic and proximal femur stress fractures, it was found that 70.3 % of patients had a stress fracture at more than 1 site. In patients with pubic stress fractures 89.2 % had concomitant stress fractures, most commonly in the sacrum and acetabulum. In patients diagnosed with acetabular stress fractures, 76 % had concomitant fractures [43].
Pelvic insufficiency fractures are generally considered low-risk for malunion or nonunion in active individuals, therefore treatment generally consists of conservative management [44]. As mentioned previously, any underlying medical cause must be investigated and treated appropriately. A period of rest with gradual return to activity is usually utilized for pelvic insufficiency fractures. Limited or non-weightbearing is prescribed for 2–6 weeks with gradual progression to full weightbearing. Prolonged low-impact activities should be achieved without pain before resumption of high-impact exercises [44].
Sacrum
Like the pelvis, sacral insufficiency fractures can be difficult to diagnose. Bone scanning is generally very sensitive for detecting sacral insufficiency fractures as a characteristic “H” pattern or the combination of concomitant sacral and parasymphyseal uptake being typical of fractures in this region [45]. Sacral insufficiency fractures are also more common in elderly individuals and can be a cause of significant back pain. As with other insufficiency fractures, osteoporosis and conditions that are associated with osteoporosis such as hyperparathyroidism and renal osteodystrophy are the most common underlying causes though a history of radiation therapy and Paget’s disease of bone are other possibilities [46, 47]. It is also important to rule out tumor as a cause of the fracture. A 2013 study found that menstrual irregularities were noted in 75 % of female athletes with trabecular bone injuries, which includes the sacrum [41]. This presents further evidence that female athlete triad risk factors are associated with insufficiency fractures normally found in elderly women.
Similar to pelvic insufficiency fractures, sacral insufficiency fractures are generally low-risk in terms of malunion/nonunion [44]. As such, many authors advocate initial conservative management consisting of rest, pain control, and modified weightbearing. Conservative management is usually the preferred treatment method in younger individuals with sacral insufficiency fracture and return to sport has been reported between 3 and 6 months in distance runners with stress fracture, many of whom exhibited characteristics consistent with the female athlete triad [48, 49]. Resolution of symptoms can be a lengthy and risky process with conservative management in older individuals; therefore, surgical treatment techniques are sometimes the preferred treatment method. Surgical management is usually only considered in younger athletes after failed conservative management. A variation to vertebroplasty, sacroplasty, is one possible surgical treatment method which involves injection of polymethylmethacrylate cement under fluoroscopic guidance into the fracture. The benefits of sacroplasty as compared to conservative management include earlier mobilization and symptom relief as well as reduction of risks associated with prolonged immobilization [47, 50, 51]. Techniques of this procedure vary per surgeon as posterior approach, long axis approach, and midline approach have been advocated. The most significant complication is extravasation of cement outside of the fracture which may cause neurological sequelae [52].
Spine
Spinal insufficiency fractures can occur in various regions of the spine and in different areas of the vertebrae but are most often associated with the vertebral body. While spinal insufficiency fractures such as wedge or burst fractures are a common cause of back pain in the elderly with osteoporosis, literature is lacking in terms of vertebral insufficiency fractures in the younger athlete. Theoretically any condition that leads to decreased bone density in an athlete could predispose an athlete to sustain spondylolysis or a vertebral insufficiency fracture similar to an elderly osteoporotic patient. As such, care must be exercised in evaluating an athlete with back pain and risk factors for decreased bone density.
Hip and Femur
Insufficiency fractures of the femur can occur at several locations including the femoral head, femoral neck, femoral diaphysis, and femoral condyles. An insufficiency fracture of the hip can be a catastrophic injury in an athlete and as with other insufficiency fractures prevention and treatment of underlying risk factors are of monumental importance in minimizing time-lost to these injuries. Patients presenting with stress fractures in the hip often report pain in the anterior groin and pain with internal and external rotation of the hip [53, 54]. Radiographs and oftentimes MRI are of great importance in distinguishing these conditions because hip pathologies such as femoroacetabular impingement often present with similar anterior groin pain and pain with hip internal rotation.
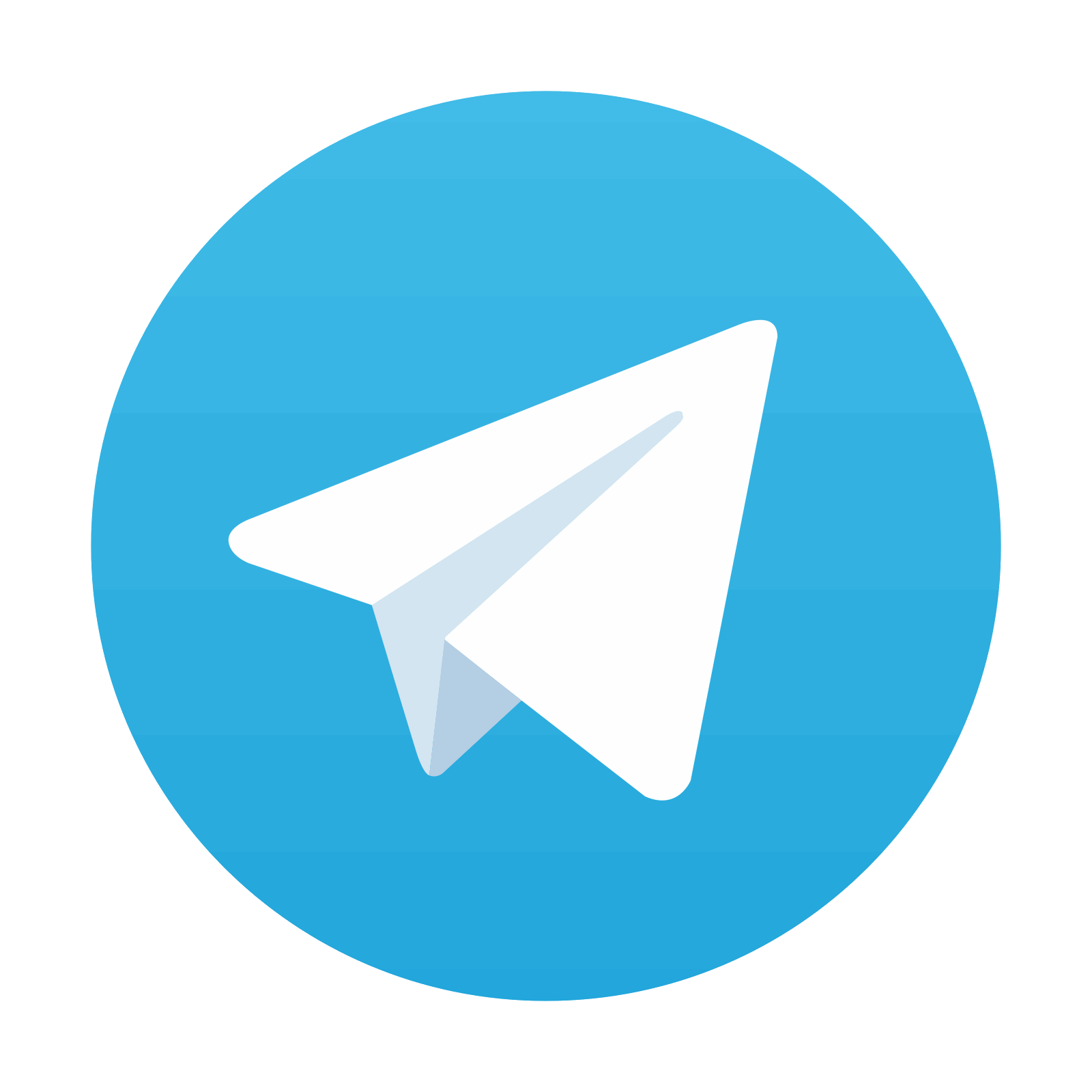
Stay updated, free articles. Join our Telegram channel

Full access? Get Clinical Tree
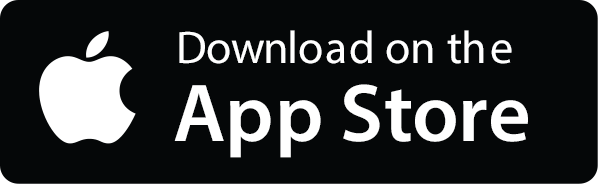
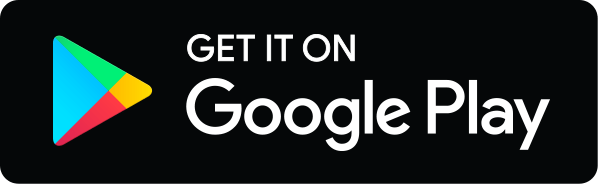