Fig. 38.1
Measurement of anterior knee laxity with the KT-1000®
The KT-1000® was developed in the 1980s by Daniel et al. [22]. To date, this is the most common device to measure anterior knee laxity. Subjects are tested supine with both knees resting on a support at 30° of flexion. The device is secured to proximal and distal tibia with two Velcro® straps. The KT-1000® measures the anteroposterior displacement between two sensors: one is held by the examiner in contact with the patella, and the other is placed anterior on the tibial tuberosity. The examiner manually applies a force of 67, 89 and 134 N or to the maximal manual force on the shank. Although this device is widely used, its precision and reproducibility have been questioned. Several authors reported an intraclass correlation coefficient (ICC) above 0.8 [35, 65]. The reliability of the device however diminishes if the examiner is not experienced (ICC = 0.65) [14]. In ACL-injured patients, the inter-examiner ICC even decreases to 0.55 [80]. As the force is applied manually, the examiner [11] and its dominant hand [80] seem to critically influence laxity measurements. As such, in certain conditions, it seems that the reliability of the KT-1000® is lower than for the Lachman test [104]. However, with a maximal manual force, the sensitivity and specificity of the device are excellent (93 %) for the diagnosis of ACL injuries [101].
38.1.1.2 Rolimeter® (Aircast Europa, Neubeuern, Allemagne) [10] (Fig. 38.2)

Fig. 38.2
The Rolimeter®: a simple device to measure anterior knee laxity
The Rolimeter® is light, easily transportable, cheap and sterilisable. Force application is not calibrated and is done manually. This device is similar to the KT-1000®. The Rolimeter® is considered, along with the KT-1000®, to be the device that provides the best results for anterior laxity [72]. Furthermore, it is as reliable as the KT-1000® [10, 79] even when used by novice examiners [63]. Its sensitivity and specificity in the diagnosis of ACL injuries are similar to the KT-1000® [30] but have been reported to be lower in comparison to the Telos® device [70].
38.1.1.3 GNRB® (Genourob, Laval, France) [73] (Fig. 38.3)

Fig. 38.3
The GNRB®: an electric jack applies an anteriorly directed force while two skin electrodes record the activity of the hamstrings. Anterior displacement is measurement at the tibial tubercle
The GNRB® is the first device that proposes a mechanised application of the anterior force under standardised and controlled conditions. The patient lies on a standard examination table in the supine position with the arms placed along the body. The lower limb lies on a composite thermoformed support, adaptable to leg length, with the knee in neutral rotation. The knee is placed on the device so that the inferior pole of the patella corresponds to the lower border of the patellar support. The joint line is palpated and is placed between the support and the jack. An electronic jack applies an anterior force of 67, 89, 134, 150 or 250 N to the postero-superior part of the calf. Two skin electrodes record the hamstring muscles activity during the test to avoid any muscular contraction and exclude false negative results [25]. A motion sensor (precision: 0.1 mm) records the relative displacement of the anterior tibial tubercle with respect to the femur. Data obtained from the displacement sensor are collected on a computer. These data include measurement conditions (pressure applied to the patellar, forces) and results (differential laxity in mm, differential slope in μm/N) to reproduce the force-displacement curve (Fig. 38.4).


Fig. 38.4
Typical force-displacement curve obtained with the GNRB® in the healthy (green) and injured (red) knee of an ACL-injured patients with a complete tear
The GNRB® has been reported to have a greater reliability than the KT-1000® regardless of the examiner’s experience [20]. However, despite a high intra-examiner ICC, Vauhnik et al. reported a weak inter-examiner ICC inferior to 0.4 which may have been caused by variations in patient installation [102, 103]. To measure anterior knee laxity, an anterior force of 134 N has usually been advised as this represents the standard force with the KT-1000® and because it is well tolerated in acute injuries. For this amount of force and for a side-to-side difference of 3 mm between knees, the GNRB® reaches a sensitivity of 70 % and a specificity of 99 % to detect complete ACL tears [73]. Sensitivity increases to 92.2 % to detect complete ACL tears with a force of 200 N. A similar specificity of 98.1 % can be observed at this force [45]. The GNRB® may also be useful to detect partial ACL tears (Fig. 38.5). At 134 N and for a side-to-side difference of 1.5 mm, a sensitivity of 87 % and a specificity of 87 % could be observed for ruptures of the anteromedial bundle of the ACL [73]. For similar tears, a sensitivity of 72 % was observed by Di Ioro et al. [24]. At 250 N, a side-to-side difference of 2.5 mm provided a sensitivity of 81 % and a specificity of 87 % [49]. As such, the diagnostic power of the GNRB® seems to be similar, or even better, compared to radiographic laxity evaluation methods [41, 49].


Fig. 38.5
Typical force-displacement curve obtained with the GNRB® in the healthy (green) and injured (red) knee of an ACL-injured patient with a partial tear (anteromedial bundle)
38.1.1.4 Radiostereometric Analysis (RSA)
RSA was developed 40 years ago in Sweden by Göran Selvik. This technique is both the most precise and the most invasive method as it requires the surgical implantation of intraosseous tantalum beads of a diameter of 0.8–1.6 mm [93]. They are implanted into the patient’s knee at the distal part of the femur and proximal part of the tibia. Two radiographs are performed simultaneously, and the anatomical position of the markers is determined with the help of a calibration cage. This tridimensional technique has a precision of 0.1 mm [96] and has the advantage not to be influenced by skin movement artefacts [95]. It is more discriminant than the KT-1000® in the post-operative follow-up of ACL patients. The KT-1000® indeed reported lower side-to-side differences than the RSA thus probably overestimating the stabilisation brought by the reconstruction of the ACL [42].
38.1.1.5 Telos Stress Device® (Telos GmbH, Hungen-Obbornhofen, Germany) (Fig. 38.6)

Fig. 38.6
Knee laxity measurements with the Telos Stress Device®. On the picture, analysis of posterior knee laxity at 90° of knee flexion
The patient is lying on the side to study anterior knee laxity and supine to assess varus-valgus. The tested leg is placed within two fixed bars inducing 25° of knee flexion. An anterior force is applied at the proximal posterior part of the shank. A dynamometer displays the amount of applied force: 9 or 15 kg, and a lateral radiograph is realised in this constraint position. Anterior displacement is represented by the distance of two parallel lines: the first line is tangent to the posterior corner of the medial condyle and perpendicular to both tibial plateau; the second is tangent to the posterior border of the medial tibial plateau and perpendicular to both tibial plateau. The distance between both lines is measured in millimetres by the radiologist. Sensitivity of the Telos® is inferior to the KT-1000® (72 % for Telos®; 91 % for KT-1000®) [16]. As such, some authors do not recommend the use of the Telos in the diagnosis and follow-up of ACL injuries [91].
38.1.1.6 Lerat’s Method [50]
Lerat’s method is easy to use and inexpensive. The patient is lying supine with the hips at the border of a radiological table. The knee is placed on an adapted support inducing 20° of knee flexion. A mass of 9 kg is attached to the patient’s thigh above his patella to induce a posterior translation of the femur compared to the tibia. This technique is reliable (intra-tester ICC > 0.9) [51]. The method allows for an individual evaluation of the anterior displacement for the medial and lateral tibial plateau [51]. This method has been reported to be less sensitive than the Telos® or the GNRB® to detect the different types of ACL remnants [13].
38.1.2 Rotational Laxity Measurements
First attempts to measure rotational knee laxity were made in the beginning of the 1980s [48, 59, 67]. Much more complex than anterior knee laxity measurements, rotational knee laxity measurements are not yet used in the daily clinical practice and are still at an experimental stage. In ACL injuries, internal rotation is of main interest even if both internal and external rotations are usually measured. Rotational knee laxity is highly influenced by the patient’s position and by the location of rotation measurement. Knee rotation is higher if the knee is flexed at 90° compared to 20° and if the hip is extended compared to flexed at 90° [84]. In ACL injuries, it is usually advised to evaluate patients at 30° of knee flexion. Cadaver studies indeed revealed that the increase in rotation induced by the ACL tear is not detectable anymore above this degree of knee flexion [7, 83, 106]. Regarding the location of the measure of rotation, if the rotation angle is measured at the foot, the tibiofemoral rotation will be overestimated [3]. Foot rotation can represent up to two-thirds of the final measure [84]. To avoid these artefacts, some devices use electromagnetic sensors placed on the tibia [3], which is the most precise method, or assure a good fixation of the ankle and foot (Rotam).
38.1.2.1 Rottometer® [4]
The patient is sitting on a modified chair with knees and hips flexed to 90°. To target tibiofemoral rotation, the thigh is fixed above the knee with clamps. Two screws at the calcaneus and four screws placed at the medial and lateral malleoli fix the ankle. Rotation is measured at the foot with a graduated protractor. A comparative study between the Rottometer® with the RSA technique showed a systematic overestimation of knee rotation which increases with the applied torque [4]. Inter-rater ICC of the Rottometer® reached from 0.69 for a torque of 9 Nm and a knee flexed at 30° [6].
38.1.2.2 Rotameter® [55]
Two prototypes of the Rotameter exist. In both versions, the subject is lying prone to reproduce the dial test position. Thighs are fixed in half cones with two Velcro® strap bands. Hips are extended and knees flexed at 30°. The subject is wearing boots (home-made boot in the first version and ski boots of appropriate size in the second version). They are attached to the handle bar that allows both applying the torque and measuring the degree of rotation. In vivo, inter-rater ICC for the first version of the device has been reported to be greater than 0.88 [56]. A cadaver study comparing the Rotameter with a navigation system showed that the Rotameter overestimated up to 5, 15 and 25° the total range of rotation at 5, 10 and 15 Nm, respectively, but was highly correlated to the navigation system [54, 55].
38.1.2.3 Rotam (Genourob, Laval, France) [77] (Fig. 38.7)

Fig. 38.7
The Rotam: the torque application is motorised, and the rotation is acquired with the help of a gyroscope
The Rotam is currently under development. It measures knee internal and external rotation induced by a motorised torque between 3 and 10 Nm. The lower limb lies on a composite thermoformed support inducing 30° of knee flexion. The thigh is fixed with a strap and the clamping force is monitored. Foot and ankles are fixed together with two straps into a custom-made boot, which immobilised them with the tibia. The starting position of the knee requires the patella to be at the highest, vertical position. The boot is adjusted to the patient’s natural position (usually slightly in external rotation) and calibrated to avoid any constraint on the boot sensors. All internal and external measurements are taken from this neutral position with the help of a gyroscope (precision: 0.1°). Data are acquired continuously, usually under a torque of 5 Nm, to reproduce the torque/displacement curve and calculate the side-to-side differences (Fig. 38.8).


Fig. 38.8
Typical torque-displacement curve obtained with the Rotam before (green) and after section of both anterior and anterolateral ligaments (red)
38.1.2.4 Device Presented by Branch et al. [17]
This device is motorised with the patient lying supine with knees flexed at 25°. Femur and patella are stabilised with clamps. The ankle is stabilised in pronation and dorsiflexion to limit its rotation during the test. This device is adjustable to the natural frontal plane leg alignment. Rotation is measured at the foot with an inclinometer. The authors showed that only 49 % of the rotation measured at the foot corresponded to the tibiofemoral rotation [17]. ICC for total range of rotation reached 0.97, but was not evaluated for internal and external rotation separately [17].
38.1.2.5 Rotational Measurement Device [2]
This device consists of three parts: a femoral clamp and a tibial splint to which are fixed inclinometers to measure rotation and a boot with a torque wrench. The rotational measurement device allows for a better evaluation of femorotibial rotation compared to a system which measures the angle of rotation at the foot. The latter option multiplies by three the observed values. The device only showed slightly increased values compared to direct measurements at the tibia with electromagnetic sensors [2].
38.1.2.6 Vermont Knee Laxity Device® [98]
The Vermont Knee Laxity Device® measures anterior, rotational and varus-valgus laxity. The subject is lying supine with knees flexed at 20° and hips at 10°. The thighs are fixed with clamps at the femoral epicondyles. The angle of rotation is measured on the tibia through electromagnetic sensors. The ICC is above 0.86 for internal, external and total range of rotation [89]. Ninety-five per cent CI of the absolute measurement errors were evaluated to reach 5–7° for internal and external rotation, but was not reported for anterior displacement [89].
38.1.3 Physiological Knee Laxity and Its Role for ACL Injuries
Physiological knee laxity represents the amount of laxity which is considered to lie within the “normal” range. It does not represent knee range of motion, and hence does not include the recurvatum knee in hyperextension, which is often cited in the context of ACL injuries and hyperlaxity [99]. Although limited data are available comparing recurvatum and laxity, both seem to be weakly correlated [53].
Physiological laxity has been more extensively studied due to the recent development of specific arthrometers with improved measurement characteristics. Nevertheless, validity, precision and reference values from a control population are seldom reported, and the results from one device should not be generalised and applied to another one. Still, these aspects are critical, since they allow for an evaluation of the clinical significance of a particular laxity measurement. Thus, ideally every arthrometer should have its own reference values.
Knee laxity is specific to every individual. Based on anterior laxity measurements with the KT-1000®, values between 1.5 and 14 mm have been reported in the literature in healthy subjects, while up to 21 mm has been measured in healthy contralateral knees of ACL-injured patients and up to 29 mm in patients after ACL injury [81]. Thus, anterior laxity in the contralateral knees of ACL-injured patients on average is greater than the laxity of healthy control individuals [99, 105]. These results suggest that increased physiological laxity could be a risk factor for ACL injuries. The same holds true for rotational knee laxity, which seem to play a similar role in this regard [17, 62].
Physiological knee laxity is influenced by several parameters, which makes it more difficult to establish reference values. The characteristic which is the most frequently discussed in the literature is sex, since women have higher laxity in general compared to men. However, some studies do not confirm this observation, reporting differences of less than 0.3 mm [78, 82], while others do, but based on differences of less than 1.5 mm [74, 99, 108]. One study described a difference of 2.5 mm between men and women [90]. Since the measurement precision of the arthrometers used is rarely reported, the question remains open. On the other hand, sex differences regarding rotational laxity are less controversial [5, 17, 40, 61, 71]. It has been shown that women have up to 40 % higher knee rotation compared to men [61, 71], which could represent a risk factor for the higher ACL injury incidence in women.
Other parameters have been shown to influence physiological knee laxity. Body mass seems to have a considerable impact on rotational laxity [61, 85]. Age can also influence the results, with the paediatric population showing generally greater knee laxity [12, 27, 38]. Similar observations have been made regarding rotational laxity [12]. Knee laxity develops during knee maturation and stabilises around 14 years for girls and around 16 years for boys [12, 27, 38]. No difference has been observed in the paediatric population between girls and boys [12, 27]. As regards the changes in knee laxity at adult age, only limited and inconsistent data exist in the literature [5, 85].
Another factor, which could have an influence on the physiological knee laxity, is the menstrual cycle, but its role on the ACL injury risk has not been clearly established [86, 87]. Lower leg alignment has also been considered to impact on laxity [85, 88] as well as sport activity that increases ACL laxity, while rest decreases it [23]. The team of Shultz et al. [85] has studied combined anterior, rotational and varus-valgus laxity, as well as recurvatum knee. They have shown that healthy individuals with increased frontal and transverse plane laxity tend to have lower values for body mass index, femoral length and muscle force and are generally younger. In this population, increased sagittal laxity was correlated with greater hip anteversion and was inversely proportional to navicular height. Thus, it seems possible to establish knee laxity profiles. Since the different laxity types (sagittal and rotational) are weakly correlated [90], they provide complementary information and should allow to establish more detailed individual knee laxity profiles.
Even though the influence of physiological knee laxity on knee function has not been clearly established, several indicators suggest that it could be related to ACL injury risk and that it could even determine the outcome of ACL reconstructive surgery [18, 43, 44]. However, more data are required to confirm these preliminary conclusions. Recent progress in the area of knee laxity measurements suggests that future multidirectional evaluations will provide complementary information which will allow to establish individual profiles of the static knee laxity envelope.
38.1.4 Laximetry for the Diagnosis of ACL Ruptures
Laxity evaluation in the acute state can be negatively influenced by haemarthrosis, pain and insufficient relaxation of the patient. A proper diagnostic evaluation of knee laxity is thus preferably performed days or weeks following the accident in a non-swollen and non-painful knee. The diagnosis is based on the side-to-side difference between the injured and the healthy knee. Laxity differences as scored with the objective IKDC (International Knee Documentation Committee) score are illustrated in Table 38.1. It represents the global reference to describe objective knee function after injury or surgery. Since its last update, this classification has never been questioned, although the surgical procedures for ACL reconstruction have changed over the years. For example, the laxities described under C or D have become rare in everyday clinical practice, and the reference laxity measurements – initially defined by the KT 1000® – are not necessarily valid for other arthrometers. However, it is generally accepted that a laxity difference of 3 mm at an applied anterior force of 134 N (KT-1000®, GNRB®) or a difference in the laxity slope greater than 10 μm/N reflects a complete ACL lesion (GNRB®) [49]. Based on the Telos system, the threshold of laxity difference at 15 kg is however 5 mm, yielding a sensitivity of 81 % and a specificity of 82 %. As well, with the Rolimeter, a similar threshold leads to a sensitivity of 67.5 % and a specificity of 84 % [70].
Table 38.1
Laxity evaluation as presented in the IKDC knee examination form
A (normal) | B (nearly normal) | C (abnormal) | D (severely abnormal) | |
---|---|---|---|---|
Lachman; 134 N | <3 mm | 3–5 mm (+) | 6–10 mm (++) | >10 mm (+++) |
Lachman; manual maximal anterior endpoint | <3 mm | 3–5 mm | 6–10 mm | >10 mm |
Currently, the instrumented diagnosis of ACL injury is essentially based on anterior knee laxity. However, the combination of anterior and rotational laxity measurements could increase sensitivity. Regarding rotational knee laxity, there is not yet a consensus concerning the laxity difference threshold to define an ACL injury. A cadaveric study based on 24 healthy knees in which the ACL ligament was sectioned revealed an increase of 3.6 ± 1.2° in internal rotation when applying a torque of 5 Nm (Robert H, personal communication, 2015). An older clinical study on patients with chronic ACL lesions showed that medial tibial rotation of the injured knee was 3.0 ± 6.6° using a torque of 10 Nm, the knee being in 20° flexion, compared to the healthy side [58]. These preliminary results suggest that side-to-side laxity difference may be weak, which highlights even more the need for accurate and reproducible arthrometers to detect ACL injuries.
Ideally, the best diagnostic capacity is achieved by choosing a detection threshold, which provides the highest possible sensitivity (capacity of detecting an ACL rupture) while at the same time yielding the highest specificity (capacity of detecting a healthy knee). By privileging the sensitivity, it is possible to detect a greater proportion of ACL injuries, but this will decrease the specificity, which will yield more false-positive cases. Another possibility is to choose a detection threshold, which optimises the number of correctly classified patients and healthy control subjects.
The studies, which have investigated the diagnostic capacity of arthrometers, have often included patients with complete ACL lesions. However, this does not reflect the clinical reality, because many ACL lesions are partial ruptures. To evaluate the true diagnostic capacity of an arthrometer, it is therefore preferable to consider all kinds of ACL lesions and to determine if different types can be identified prior to surgery.
Several subtypes of ACL lesions have been described in the literature. Based on arthroscopic classification, it is possible to distinguish lesions of a single bundle (the anteromedial bundle being more often concerned than the posterolateral one) with or without a functional remnant, complete lesions with total resorption of the ligament or with a healed remnant on the notch or the PCL [70]. These different scenarios can influence the translational or rotational side-to-side laxity difference [13, 21, 49, 66, 69]. Those lesions where the ligament has totally disappeared are the easiest to diagnose: they are frequently observed in patients with a long-standing lesion, are more often associated with meniscal lesions and present greater side-to-side laxity differences. A second group that can be identified concerns patients with a ligament remnant healed on the PCL, with a laxity difference close to the one of complete ruptures. Those lesions with conservation of the posterolateral bundle are generally more stable anteriorly than the previously described. This is in accordance with the fact that the anteromedial bundle restraints chiefly anterior translation. Those lesions with a healing on the notch pattern are the most stable ones [69].
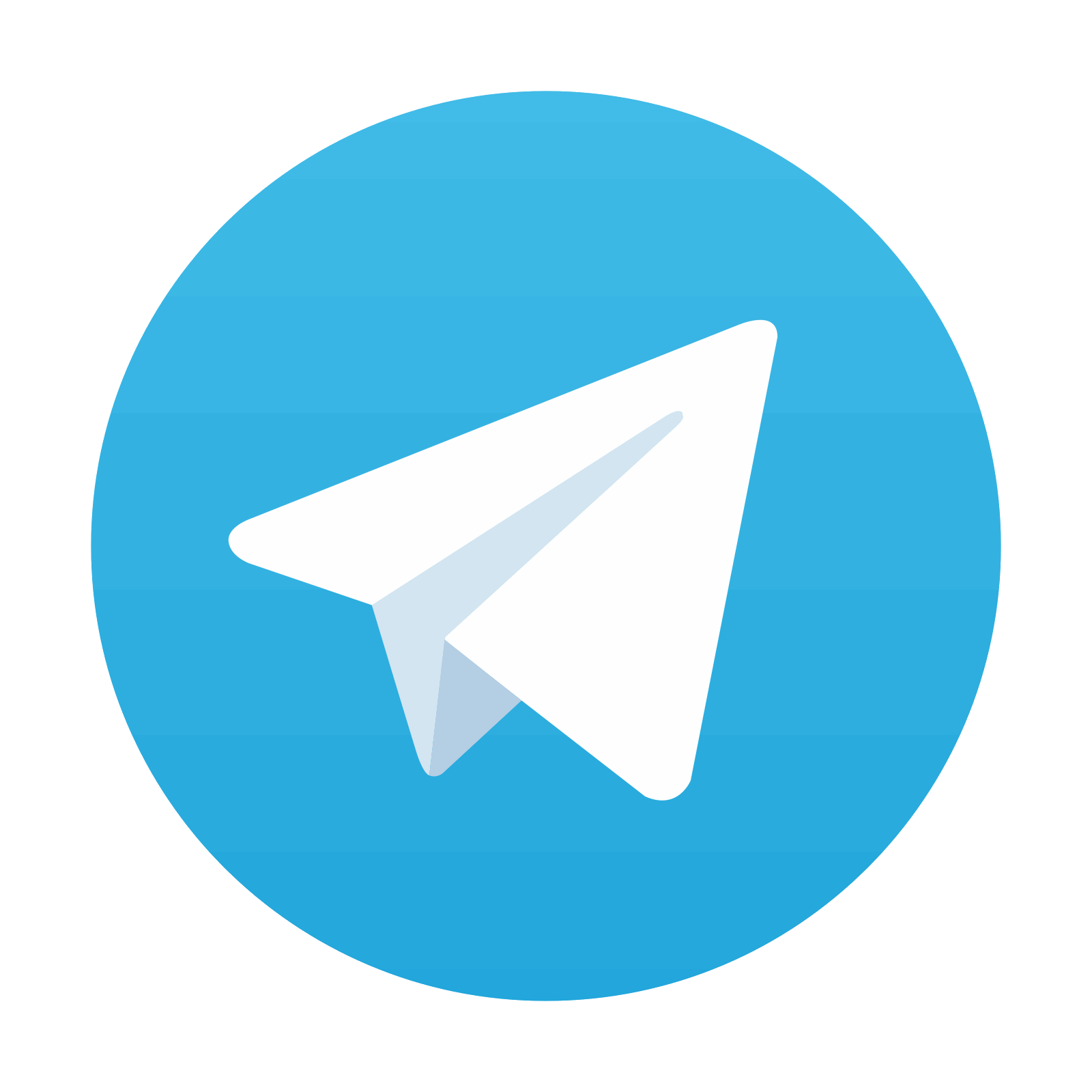
Stay updated, free articles. Join our Telegram channel

Full access? Get Clinical Tree
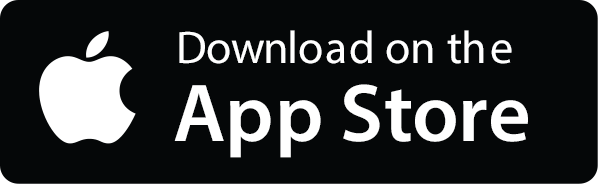
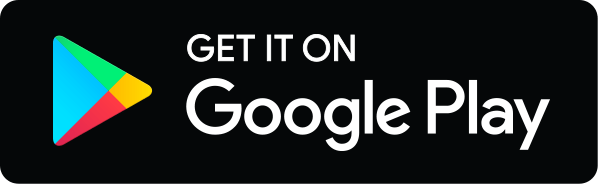