The treatment of articular cartilage lesions is complicated, but novel tissue engineering approaches seem to improve the outcome. A tissue engineering approach is less invasive and reduces surgical time, periosteal hypertrophy, and morbidity. Cell-based therapies using scaffolds have advantages compared with microfracture techniques, but the efficacy and cost-effectiveness need to be investigated. Second-generation cell-based therapies have lower morbidity and the ease of the technique is not significantly different from that of first-generation autologous chondrocyte implantation techniques. Third-generation cell-based therapies such as the use of tissue engineered scaffolds need to be studied in more detail.
Osteochondral repair has been a therapeutic challenge owing to the complex biomechanical properties and poor healing capacity of articular cartilage. Cartilage lesions have limited repair potential because of their avascular nature and lack of access to reparative and humoral factors that promote healing. Full-thickness osteochondral defects that have access to the subchondral bone marrow cells, mesenchymal stem cells, growth factors, and cytokines are able to undergo repair, but fibrocartilage is deposited in place of hyaline articular cartilage. A conservative approach to the treatment of the osteochondral defect significantly increases the risk of developing early osteoarthritis and functional disability. Repair in weight-bearing joints such as the knee has been particularly challenging in pediatric patients who wish to resume levels of activity regardless of the pain, swelling, stiffness, or instability.
Current treatment methodologies include (1) microfracture, (2) arthroscopic lavage and debridement, (3) mosaicplasty, (4) periosteal and perichondrial transplantation to full-thickness cartilage defects, (5) autologous chondrocyte implantation, and (6) cell-based and scaffold treatment.
Microfracture is a technique of bone marrow stimulation by creating perforations in the subchondral bone. This technique results in the migration of mesenchymal stem cells from the bone marrow to the site of the lesion, creating a clot. The mesenchymal stem cells are then able to differentiate into fibrochondrocytes that participate in the repair of the chondral defect. This technique, which is straightforward, is commonly used for the management of small chondral defects. However, studies have shown that the results are unsatisfactory in athletic or elderly patients.
Arthroscopic lavage and debridement can provide short-term relief. The goal of arthroscopic debridement and lavage is to reduce the inflammation and mechanical irritation within the joint. Debridement may include smoothening of the fibrillated articular surface, meniscal trimming, shaving of osteophytes, and removal of inflamed synovium. Joint lavage is thought to reduce synovitis and pain by washing fragments of cartilage and calcium phosphate crystals from the knee. Although these procedures have been reported to produce some temporary palliative effects, especially in older, low-demand patients, they are not a long-term solution.
Resurfacing of the osteochondral defect is achieved via mosaicplasty. This technique involves the transfer of osteochondral plugs from less-weight-bearing surfaces of the medial and lateral condyles of the femur. Also known as autologous chondral mosaicplasty or osteoarticular transfer system (OTS), it provides several advantages such as the direct transplantation of hyaline cartilage, shorter rehabilitation duration, and being able to complete the technique in 1 surgery. Disadvantages include donor site morbidity, limited availability of grafts, and mismatch between donor and recipient graft sites, which could result in poor chondral integration. Osteochondral allograft transplantation using either fresh, cryopreserved, or fresh-frozen allografts may overcome the disadvantage of donor site morbidity; however, this has a significant risk of immunologic rejection and disease transmission.
Autologous chondrocyte implantation (ACI) has shown great promise for the treatment of full-thickness patellofemoral chondral defects. However, it presents some limitations: there is the need for general, or at least regional, anesthesia (to harvest the chondrocytes and transplant expanded cells) for 2-knee procedures. There is also the difficulty in obtaining an adequate number of chondrocytes, a slow rate of chondrocyte proliferation, and donor site morbidity.
Cell-based therapies that involve either use of ACI or bone marrow–derived mesenchymal stem cells have evolved considerably. The use of biocompatible, biodegradable scaffolds onto which the cells are seeded has significant advantages such as an even distribution of cells and low donor site morbidity (because there is no need for periosteal harvesting or implantation, which also simplifies the technique).
The purpose of a scaffold is to act as a template to promote cellular interactions and formation of the extracellular matrix that provides the structural framework for the newly formed tissue. It is important that the scaffold, a temporary structure, mimics the physiologic conditions of the extracellular matrix because this affects the differentiation potential of the cells being seeded. There are certain requirements for a scaffold to be suitable for tissue engineering applications. It should be biocompatible, biodegradable, nonimmunogenic, and nontoxic. In addition, the structure of the scaffold (ie, both its macrostructural and microstructural properties) affects the potential for adequate cell survival, proliferation, growth, and organization within the scaffold construct. It also plays a significant role in gene expression and the modeling of cell shape.
Porosity and interconnectivity of the scaffold play important roles in both in vitro and in vivo processes such as cell adhesion, growth, reorganization, and neovascularization. Surface properties of the scaffold such as morphology, hydrophilicity, surface energy, and charge should also be considered because they influence the adhesion, migration, intracellular signaling, cell recruitment, and healing of the tissue-scaffold interface. The mechanical strength of the scaffold should be similar to that of native tissue because this aids in withstanding the natural stresses and loads being applied, and prevents mechanical failure caused by premature loading of the native tissues. This property is particularly critical in bone and cartilage tissue engineering scaffolds because these are weight-bearing tissues.
The integration of growth factors such as bone morphogenic proteins (BMPs) into the scaffold structure by means of structural entrapment or surface complexes (ionic or covalent bonding) has been widely used. Growth factors play an important role in guiding and controlling cellular behavior. Novel methods are being explored, such as the use of growth factor–based microspheres and bioactive microstructures (bioactive glass and ceramics) to create scaffolds.
Natural and synthetic polymers have been used for cartilage regeneration. Synthetic polymers such as biodegradable and bioabsorbable polymers, polylactic-co-glycolic acid and polycaprolactone have been used for cartilage regeneration. Polyurethanes, polyhydroxyalkanoates, and polyphosphazene are some of the other synthetic polymers being investigated. Natural polymers such as collagen, fibrin, and hyaluronic acid have also been used for the same purpose. Hydroxyapatite, calcium phosphates, and bioactive glasses are some of the inorganic materials that have been composited with polymers to significantly increase the bioactivity and mechanical properties of the scaffolds.
Currently available treatment methodologies using scaffolds for osteochondral repair are (1) matrix-associated chondrocyte implantation (MACI), (2) hyaluronan-based scaffolds, and (3) tissue-engineered collagen scaffolds.
MACI
MACI is a technique that uses cultured autologous chondrocytes that are implanted onto a type I/III porcine collagen matrix. The cell seeding is done a few days before implantation. Results have shown expression of aggregan, type II collagen, and S-100 with a 75% hyalinelike tissue at 6 months. In a prospective randomized study comparing this technique with ACI, Bartlett and colleagues found similar results, and 66% rated good to excellent scores on the International Cartilage Repair Scale (ICRS). Type I collagen has also been used as a matrix to support autologous chondrocytes.
By overcoming the need to harvest and implant a periosteal flap, MACI has a significant advantage compared with ACI in terms of graft site morbidity, and prevents dedifferentiation of the chondrocytes while being cultured in vitro. Arthroscopic techniques may be adopted for lesions that are accessible, thus reducing the hospital stay and postoperative complications such as periosteal hypertrophy and arthrofibrosis. In a multicenter study, Wood and colleagues showed that open arthrotomy resulted in 26% of the procedure-related complications. Suture-free fixation has been explored using fibrin glue, which has shown a success rate of 88% in weight-bearing chondral defects of the femoral condyle.
The efficacy of MACI was studied by Jones and colleagues using animal models. They created 6-mm defects on the trochlea and medial femoral condyle that were treated with either MACI or unseeded, porcine-derived type I/III collagen membrane. At 10 weeks after surgery, arthroscopic assessment showed better fill, integration, and appearance in the study group. Magnetic resonance imaging (MRI) results were also consistent with MACI being superior to the control group. Immature cartilage and poor architectural constructs were seen in the control group.
Behrens and colleagues reported on a 5-year follow-up of 34 patients treated with MACI. They found that these patients had a localized cartilage defect with a mean size of 4.1 cm 2 . Lysholm-Gillquist, Meyer, and ICRS scores showed significant improvement in 11 patients (32.35%).
Trattnig and colleagues used noninvasive cartilage-specific MRI to monitor 20 patients at sequential intervals following repair of cartilage defects using MACI. They found that an incomplete filling defect improved to a complete or less incomplete filling of the defect in 10 patients (50%). Within a year, 3 cases of cartilage hypertrophy returned to normal and integration with the native cartilage was complete in 10 patients. The signal intensity of the implanted cartilage was also found to be the same as that of the native cartilage in 13 patients (65%).
Bartlett and colleagues studied the use of a sandwich technique using 2 MACI membranes on 8 patients (age range 18–46 years). The patients were subsequently assessed at 6 months and 1 year after surgery using the modified Cincinnati knee, the Stanmore functional rating, and the visual analog pain scores. Results showed that, within 6 months, all patients improved, with further improvement at the end of the first year. The clinical outcomes were also found to be good or excellent in 4 patients (50%) after 6 months and 1 year.
Hyaluronan-based scaffolds
Hyaluronic acid is a linear molecular mass polysaccharide that is commonly referred to as hyaluronan. Hyaluronan is a major component of the extracellular matrix of various connective tissues. It functions in providing structure as well as binding of bioactive molecules and behaves as a lubricant protecting articular cartilage surfaces. It has been widely used in tissue engineering owing to its good biocompatibility, viscoelastic properties, nonimmunogenic properties, and ease of chain size manipulation. It also facilitates extracellular matrix remodeling via its interaction with cell surface receptors that promote the migration of cells. Chow and colleagues showed that, via cell surface receptor interactions with chondrocytes, hyaluronan allows the chondrocytes to maintain their native phenotype. Ehlers and colleagues studied the effects of hyaluronic acid on the morphology and proliferation of human chondrocytes in primary cell culture. They concluded that hyaluronan stimulates the production of type II collagen and aggregan, and promotes cell proliferation. Hyaluronan has also been conjugated with alginate, chitosan, and fibrin gel matrices and surface coated with type 1 collagen and fibronectin to facilitate cell attachment and tissue formation.
Burdick and colleagues used encapsulated porcine chondrocytes in photopolymerized hyaluronan hydrogels. They found that the cells maintained viability and were able to produce neocartilage within the porous network. Hyaluronan-derived polymers such as HYAFF7 and HYAFF11 have shown significant improvement in quality of healing of chondrocyte defects in vivo. In vitro studies have also shown that HYAFF11 3D scaffolds are able to reexpress their differentiated phenotype and also reduce the expression and production of molecules involved in cartilage degeneration. These scaffolds also have predictable degradation profiles that optimize the transition between newly formed and existing cellular matrices. MRI and arthroscopic evaluation of chondral defects treated with Hyalograft C (autologous cells implanted onto an esterified hyaluronic acid scaffold) have shown regeneration of normal cartilage in more than 75% of patients. Gobbi and colleagues studied 32 patellofemoral chondral lesions treated with Hyalograft C. They showed significant improvement in International Knee Documentation Committee (IKDC) scores in the course of a 2-year follow-up. MRI studies at 2 years’ interval showed 71% to have normal cartilage. In 6 cases, second-look arthroscopies revealed the repaired surface to be near normal with biopsy samples characterized by hyalinelike appearance. The 3-year results of a multicenter clinical study by Maracacci and colleagues showed similar results, with improved IKDC in 91.5% of patients. Preexisting cartilage defects had a mean size of 2.4 cm 2 and were Outerbridge grade III and IV. Normal or nearly normal postoperative arthroscopic evaluations were had by 96.4% of the patients in long-term follow-up. Histologic analysis showed hyalinelike cartilage in the lesion as early as 24 months after implantation. Subjective assessment also improved, with 76% and 88% of the patients having no pain or mobility problems, respectively.
Nehrer and colleagues studied 53 patients with full-thickness chondral defects treated with Hyalograft C. They found a statistically significant increase in all knee scores of patients who were treated for the primary indications (young patient with stable joint, normal knee alignment, and isolated chondral defects with otherwise healthy adjacent cartilage). Patients who were treated for secondary indications as a salvage procedure showed poor results, with 9 of the 11 patients (81.8%) requiring total knee arthroplasty between 2 and 5 years after implantation.
Kon and colleagues did a comparative study of autologous cartilage (hyaluronan-based scaffold) implantation and treatment with microfracture techniques for grade III and IV chondral lesions of the knee in 80 active patients (mean age, 29.8 years). In the course of a 5-year follow-up, they found significant improvement in IKDC in both groups. However, on return to sports, the scores remained stable after 5 years in the Hyalograft C group, whereas they worsened in the microfracture group. In a recent study, Kon and colleagues showed that both techniques have similar success in returning to competitive-level sporting activity. Microfracture resulted in a faster recovery and return to competitive-level sporting activity compared with ACI. However, there was progressive clinical deterioration over time in the microfracture group, whereas ACI offered a more durable clinical outcome.
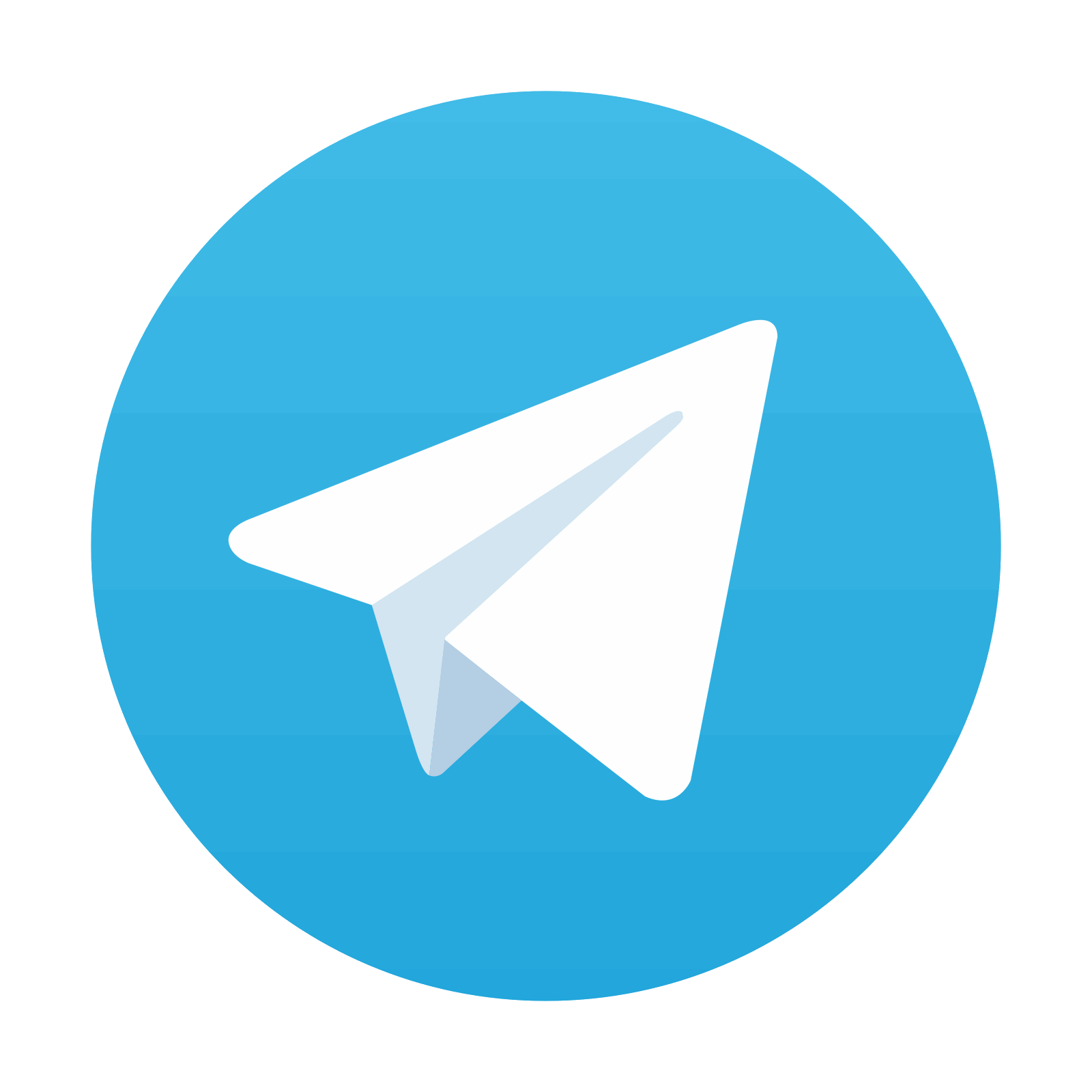
Stay updated, free articles. Join our Telegram channel

Full access? Get Clinical Tree
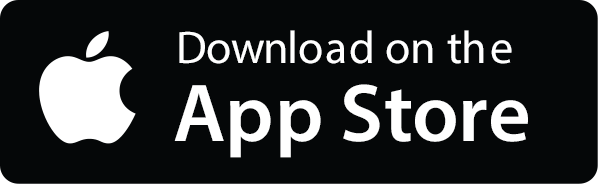
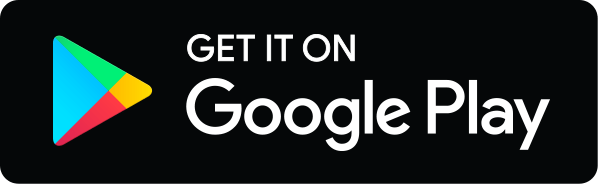