(1)
Department of Orthopedic Surgery ASAN Medical Center, University of Ulsan College of Medicine, Seoul, Korea, Republic of South Korea
Abstract
Most of the surgeons would agree that the surgical technique itself is the most important prognostic factor for TKA. However, there may be some flaws in the designs or the materials used in TKA prosthesis which can lead to a poor prognosis and early TKA failure. Even if these prostheses do not have any problem, the operation can be difficult and outcomes can be poor if an inappropriate implant is selected for the patient.
Most of the surgeons would agree that the surgical technique itself is the most important prognostic factor for TKA. However, there may be some flaws in the designs or the materials used in TKA prosthesis which can lead to a poor prognosis and early TKA failure. Even if these prostheses do not have any problem, the operation can be difficult and outcomes can be poor if an inappropriate implant is selected for the patient.
It was a general and traditional concept to adopt a graduated system for implant selection, and the selection of prosthesis was made gradually based on the severity of the lesion. For example, a unicondylar prosthesis was used in single-compartment arthritis, whereas a hinged prosthesis was used in the most severely degenerated and deformed knees. But with the development of implants and surgical techniques, implant selection is now done according to the surgeon’s choice if arthritic change, deformity, or instability is not extreme.
No matter which type of prostheses is used, the material should have certain strength and should offer biocompatibility and durability. Also, the implants should be designed such that they are compatible with the mechanics of artificial joint and should allow the performance of the operation with ease. If possible, the implants should provide various options for their use in special situations and they should cause little problems during revision surgery.
It is difficult to know at a glance which implant satisfies all these requirements since the implants show quite different results in the in vitro and in vivo studies. Generally, it is recommended to select those implants that have sound theoretical background, provide good long-term clinical results, and are familiar to the surgeon.
Here, I would like to provide information on the materials and designs of implants, bone cement, and surgical instruments used in TKA.
2.1 Material
Materials include metals for the femoral and tibial components, recently developed ceramics, polyethylene (PE) for the patella and spacers, and bone cement for fixation. These materials are produced through sufficient testing in each manufacturer’s laboratory. However, even if the materials have been proved to be excellent in an in vitro study, quite different outcomes can be resulted clinically after application of the implant to the patients. Barrack stated that it is important to understand the properties of these materials because the laboratory results do not always correspond to the clinical results.
2.1.1 Metal
The metals which are used in prostheses should be biocompatible, should have enough strength to endure the load, and should not corrode under physiological conditions. Most of the metals used in the human body are alloys. These are iron-based stainless steel alloy, cobalt chrome-based alloy, titanium-based alloy, zirconium-based alloy, and tantalum.
The properties of metals such as strength, durability, and biocompatibility are determined according to their composition and structure. These properties can be changed by various manufacturing processes applied to the metal.
Metal is a kind of crystal and is formed into various alloys to improve its natural properties. Alloys can be made by adding very small interstitial atoms (C, O, N, etc.) or little larger substitutional atoms (Mn, Al, V, etc.) to the basic metal. Application of heat to disperse the substitutional atoms uniformly is called precipitation hardening. Metal can be processed through hot working and cold working. Hot working alters its shape and solidifies the internal bonding by reducing grain sizes. The outcomes vary according to the temperature and time. Cold working reduces dislocation of metal crystals by strengthening the bonding between crystals, thereby improving yield strength and reducing ductility (Table 2.1).
Table 2.1
Changes in properties according to the metal grain or crystal size
Increase in metal grain or crystal size | |
---|---|
Ductility | ↑ |
Toughness | ↓ |
Strength | ↓ |
Young’s modulus | ↓ |
Tensile strength | ↓ |
Strength is also affected by the method in which implants are fabricated. Most implants used in orthopedic surgery are fabricated by casting, machining, or forging. Since casting may not ensure an even grain size, it can decrease the strength of the implant. Even in the best implant material, the Young’s modulus can be changed if the metal has defects during the manufacturing or fabrication, and even a 0.2 % of defect in the metal can affect its strength.
Fatigue and corrosion is closely related with durability. Fatigue strength is the break point of the material at which cracks develop under repeated constant impact of 10 7 cycles. Fatigue strength is closely related to how the metal is used (strength and direction of force) and defects in the metal, along with property of the metal. Corrosion is oxidative degradation of the metal and is caused by the electrochemical dissolution phenomenon and wear, or the synergy of both. It is rapidly accelerated when the metal breaks. The corrosion of the metal inserted into the human body has two effects: first, it reduces the implant strength, and second, the materials or ions generated by corrosion induce bone loss and inflammatory reactions around the implants. Corrosion may occur focally or on the whole metal surface. When exposed to air or saline, a very thin 2–10 nm passive film is formed on the outermost surface of the metal by oxidation of metal elements. This passive film serves as the barrier that prevents corrosion and release of metal ions. Stainless steel forms FeO, Fe2O3, and Cr2O3 (if there is Cr), while cobalt chromium forms Cr2O3 and titanium forms TiO2. The strength of this passive film and the bonding of this film with the main metal greatly affect the extent of corrosion. In order to reduce corrosion, metal is dipped in aqueous nitrogen or the surface is treated during manufacturing in order to increase the strength of this passive film.
Biocompatibility is affected by either the wear particles or materials generated by corrosion or both. These materials either spread to the entire body through the blood circulation to cause tissue damage or immune reaction or are deposited focally to evoke inflammatory reactions, tissue destruction, and tumor formation. These reactions are different according to the type of the metal, duration of exposure, and amount and size of wear particles.
2.1.1.1 Stainless Steel
Stainless steel is an iron-based alloy and is of many types according to the strengthening method used by addition of nitrogen or manganese, or according to the manufacturing process. It is mainly used in the plate and screws for fracture treatment. In the past, stainless steel has also been used in artificial joints, but it is no longer used for this purpose since it is not as reliable as cobalt chromium or titanium.
2.1.1.2 Cobalt Chromium
This alloy is very strong and resistant to corrosion but tends to lose its strength as the grain size becomes irregular when the implants are made by casting. It has low ductility and strong resistance to wear, but this means that it is hard to machine during the fabrication process. Molybdenum (Mo), carbon, nickel, silicon, or iron is added to this metal to strengthen its properties. Vitallium contains Mo; addition of 2–3 % Mo reduces pitting and corrosion.
2.1.1.3 Titanium
Pure titanium is only used to obtain porous coating since it has low strength. Currently aluminum–vanadium–titanium (Ti–Al6–V4) alloy is generally applied to the human body in the orthopedic field because it has excellent biocompatibility as it does not evoke inflammatory and toxic reactions, and it is strong and has high fatigue strength. Its elastic modulus is about half of that of cobalt chromium and this helps in distributing the load imposed on the implants to the bones so as to reduce bony resorption caused by stress shielding. However, titanium alloy has a poor mechanism of passive film formation that prevents corrosion in the body compared to that with cobalt chromium. It is no more used in the femoral component of knee joints, as it causes wear problems when articulated with PE.
2.1.1.4 Zirconium
Zirconium is a metal similar to titanium. Zirconium alloy containing 2.5 % niobium (Zr-2.5Nb) has fatigue strength similar to that of cobalt chromium. When the oxygen diffusion method is applied to zirconium, its surface gets transformed into approximately 5 μm thickness of zirconia (OxZr), which is ceramic in nature. In other words, the main part remains metallic in nature, while the surface changes into a ceramic component which reduces surface wear. Hence, surface fragmentation develops when it is sterilized by heating.
This ceramic has superior abrasive/adhesive wear performance than other metals. It is reported that zirconia can reduce the abrasive/adhesive PE wear by 40–90 % compared to cobalt chromium. Ezzet et al. reported that an oxidized zirconium femoral component could significantly reduce PE wear under simulated conditions of athletically active patients with modestly malaligned TKA prostheses. But wear of the knee joint is mainly the delamination wear, which is different from the abrasive/adhesive wear. Thus it is uncertain whether zirconia reduces the wear rate in the knee joint. Hui et al. reported no significant differences in the clinical, subjective, and radiographic outcomes with the use of zirconia femoral components. In a 5-year follow-up study, Kim also reported that there were no differences in size, weight, and shape of wear particles between cobalt chrome and zirconia. However, there were no adverse effects associated with the use of oxidized zirconium femoral implants.
2.1.1.5 Tantalum
It is a kind of biomaterial that offers several potential advantages over commonly used metals in the human body, including low stiffness, high porosity, and high coefficient of friction. Tantalum surface also has the effect to increase host white blood cell activation and lower bacterial adherence.
The elastic modulus of this metal is similar to that of the bone. Bony ingrowth occurs when 80 % porosity is maintained. It is mainly used as trabecular system in a contained defect and in prefixed tibial plate with PE.
2.1.1.6 Interaction Between Metals
When two different implants are used in the same site, if possible, the same metals should be used so as to reduce galvanic corrosion. Galvanic corrosion occurs due to electrochemical shift between metals. Galvanic corrosion is more prominent between stainless steel and cobalt chromium, and between stainless steel and titanium. It is minimal between cobalt chromium and titanium.
2.1.2 Ceramic
Ceramic has a stable surface and its wear is less as it has a low coefficient of friction. Ceramics used in the human body are the oxidative form of aluminum (OxAl) or zirconium (OxZr) derivatives. Ceramic is chemically stable as it does not undergo oxidative degeneration. Ceramic has good biocompatibility and excellent strength and is particularly resistant to corrosion and fatigue. Also, its elastic modulus is close to that of bones, so load transmission to the bones is more gradual than that of metals. The zirconia-based ceramic is less brittle and less stiff than the alumina-based ceramic. Another benefit is that the bone can grow into the surface pores of the ceramic. Due to these benefits, it has been used in the hip joint prosthesis since the 1960s.
However, it is difficult to use ceramic in the knee joint prosthesis because the knee joint has more complex biomechanics and structural congruency than the hip joint. And ceramic has to be fixed without bone cement. As a result, it causes problems of migration into the acetabulum in the hip joint, and same problems occur in the tibial side. Onishi reported that when ceramic was used in the knee joint, the major cause of failure was loosening of the tibial component, not due to the chemical reaction but due to the mechanical factors. Therefore, in order to ensure stable fixation, fabrication of a metal-backed ceramic is currently under investigation.
Ceramic is strong against primary compressive forces but is weak against tensile and shear forces. Also, it is brittle due to low ductility. When a cyclic force is applied, it can undergo a microfracture. Moreover, it may be hard to achieve homogeneity during fabrication, since it is difficult to control the grain size. The ceramic may pose problems during sterilization as it is weak against heat. Although it does not cause much wear if it articulates with PE, the zirconia–zirconia articulation dramatically increases the wear (Table 2.2).
Table 2.2
Comparison of properties of the materials used in the tibial and femoral components
Stainless steel | Cobalt chromium | Ti–Al6–V4 | Ceramics | |
---|---|---|---|---|
Corrosion resistance | 4th | 3rd | 2nd | 1st |
Young’s modulus | 3rd | 2nd | 4th | 1st |
Yield strength | 2nd | 1st | 2nd | 4th |
Ductility | 1st | 2nd | 3rd | 4th |
Hardness | 3rd | 2nd | 4th | 1st |
2.1.3 Polyethylene (Ultrahigh Molecular Weight Polyethylene: UHMWPE, PE)
2.1.3.1 History
Various materials have been used since artificial joints were introduced in the late nineteenth century. Hey-Groves used ivory in the femoral head prosthesis in 1922. Judet used synthetic polymer for the first time in 1936, but it caused problems of wear and loosening. In 1958, Charnley used polytetrafluoroethylene (PTFE; known as Teflon, but not strictly Teflon), but it was replaced with HMWPE (high molecular weight polyethylene) due to its weakness against creep deformity and abrasive wear. Currently, UHMWPE (ultra HMWPE) is used. Other similar products include polyacetal and polyester. Polyacetal was used in the early products as it has high yield strength and is easy to manufacture, but it is only used in trials currently due to its high failure rate. Polyester is not used now because it causes bone resorption.
2.1.3.2 Polyethylene (PE) in TKA
In TKA, PE is used as a spacer between the tibia and femur and as a patellar component. The mechanical strength of PE is weaker than that of metal or ceramic, but PE has the advantage of high corrosion resistance, high elasticity, and flexibility, which make it possible to make various forms of PE. Good PE should be easy to manufacture, inexpensive, not easily damaged, durable, and biocompatible.
The most common complication of recent artificial implants is the wear, and a lot of research have been undergoing to reduce the wear of PE. The factors that affect long-term implant survival and good performance of PE include molecular weight, manufacturing process, sterilizing method, cross-linking, and the properties of materials articulating with PE.
Properties
The properties of PE are closely related to its molecular weight which is correlated with the number of branches of ethylene radicals (C2H4). The molecular weight of PE is calculated by multiplication of the number of ethylene radicals.
UHMWPE is produced by polymerization of ethylene and its molecular weight is 2–6 million, which is much higher than the molecular weight of high-density polyethylene (50,000–200,000). Due to its high molecular weight, UHMWPE has an excellent impact strength, toughness, and resistance to abrasion wear. Hence, it is used in the site where a high abrasion resistance is required such as the bottoms of the skis or snow mobiles.
Properties of PE are also affected by its structure. PE is a form of semicrystalline polymer, meaning that it has crystals and amorphous component. PE originally has longer chains and can hardly form crystals. Its crystal content is determined by its molecular weight and the manufacturing temperature. Synthetic PE generally contains 58–75 % of crystals and its crystallization is altered when it is heated beyond its melting point. This crystal is the smallest structural unit of PE and gathers to form a lamella of about 10 nm in size, which determines the properties of PE (Fig. 2.1).
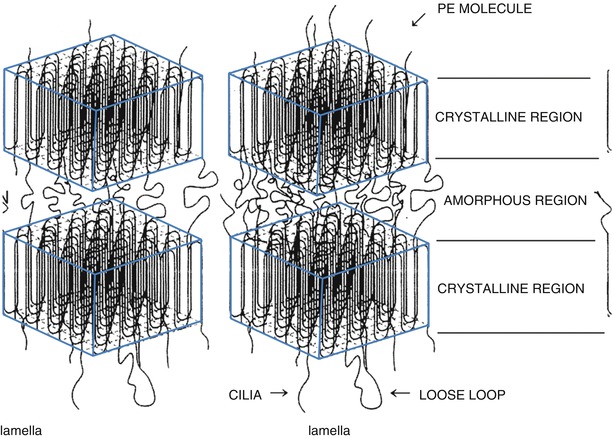
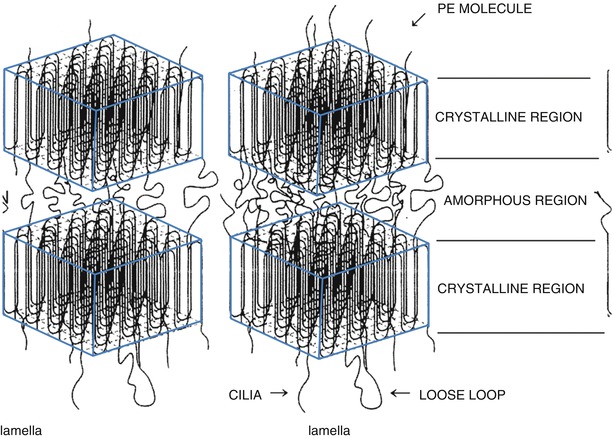
Fig. 2.1
Schematic figure of polyethylene
With high crystallization, its elastic modulus and yield strength increase along with an improvement in resistance to creep deformity and fatigue strength. Hence, many attempts have been made to improve its crystallization. One of them has been the addition of carbon. This type of PE has improved resistance against compressive and tensile forces and has higher creep resistance. However it is no longer used in artificial joints because it is difficult to mold, has low fatigue resistance, and has a high probability of surface damage. A product called Hylamer was introduced that improved crystallization using a completely different method, and improved the elastic modulus by 375 % and tensile yield strength by 30 %. However, this product had a limited commercial use because it easily underwent oxidative degradation under gamma radiation sterilization, and caused eccentric wear and rim fracture due to the design problem. Recently, vitamin E-containing PE has been introduced to prevent oxidative degradation. Haider et al. reported that the vitamin E-stabilized material had minimal changes and exhibited 73 % to 86 % reduction in wear for both CR and PS TKA prostheses.
Manufacturing
When ethylene is polymerized, it turns into a fine granular white powder. Small amounts of titanium chloride (TiCl4) and aluminum are added during the polymerization process. Four to six bar pressure is imposed at 66–80 °C to maximize the molecular weight and any particles larger than 500 μm are filtered out.
There are three methods to produce polyethylene. First is the direct molding method, which comprises of adding powder into a mold and applying pressure to it. The advantage of this method is that it is possible to make complex shapes of PE in one step and create a very smooth surface. The drawback of this method is that it is expensive due to the time-consuming process and the properties of PE can be affected by the molding conditions. Second is the ram-extruding method. Powder is poured into a cylinder and heat is applied. After that, PE is pushed out to produce a cylindrical PE of 1–6 in. in diameter, which is carved into the desired shape. Third is making compression-molded PE sheets of about 8 × 8 × 8 cm in size and then modifying them into the desired shape by machining (Fig. 2.2). But the products obtained by machining may have surface irregularities.
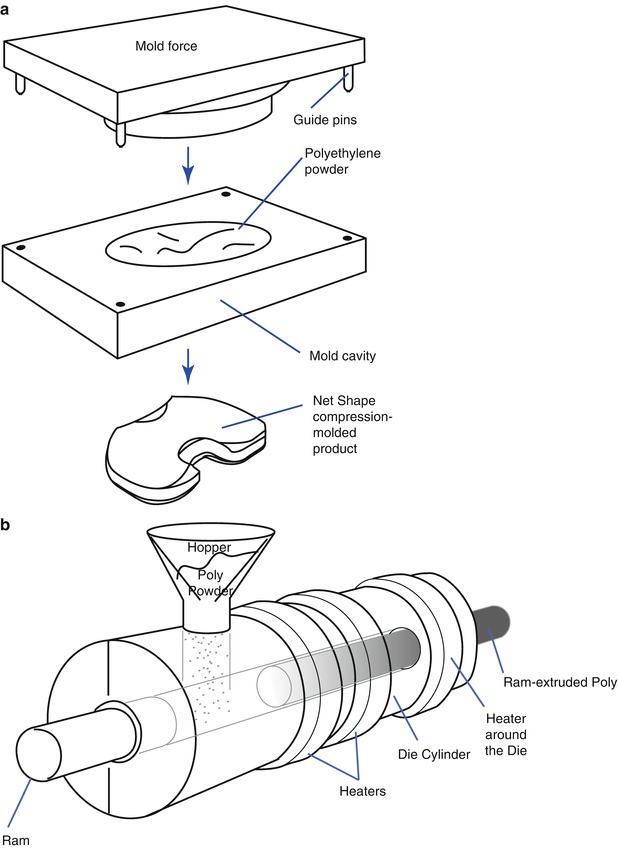
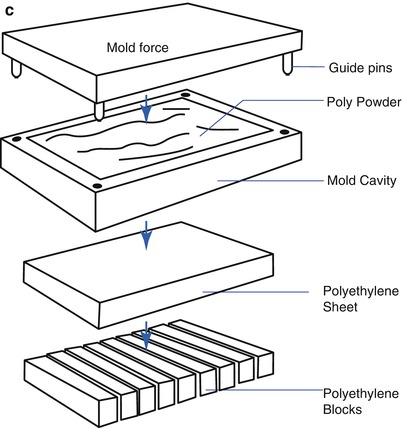
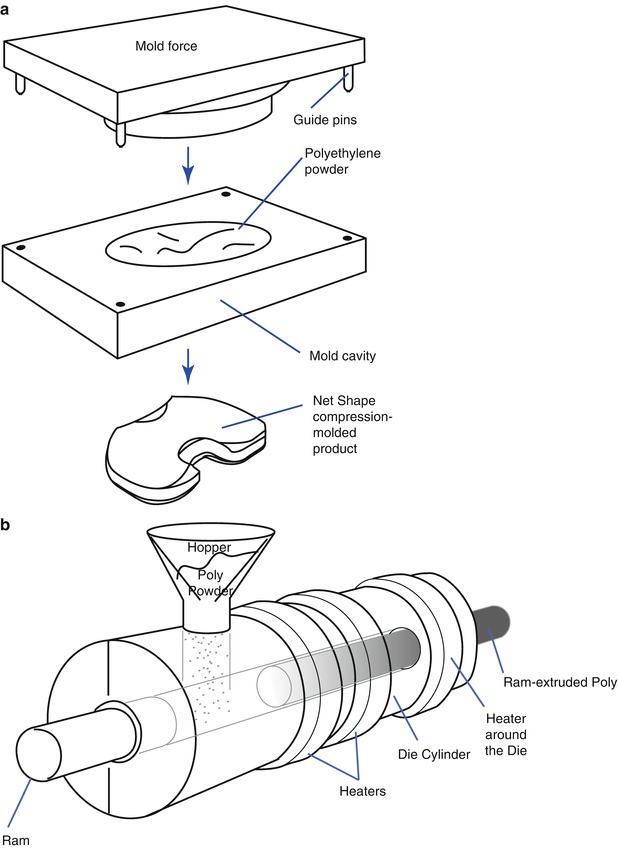
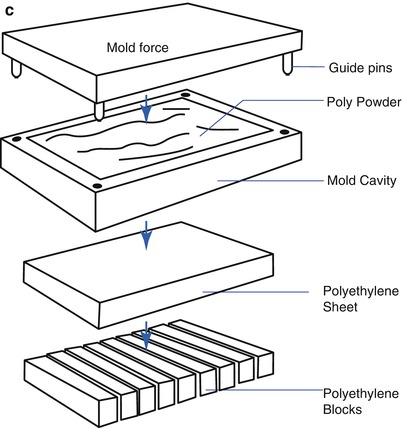
Fig. 2.2
Manufacturing of polyethylene (Courtesy of Zimmer, Warsaw, IN). (a) Direct molding (net shape), (b) Ram extrusion, (c) Compression-molded sheet machined into final parts
Recently, the annealing or remelting process has been added to the process of manufacturing PE in order to reduce the surface wear, improve the resistance to fatigue, and decrease oxidation. Annealing process involves heating the PE at a temperature below its melting point. With this process, the crystallinity and properties of PE are maintained, while the defect of PE is decreased. Remelting process involves heating beyond the melting point (135–138 °C) of PE. The properties of PE can be changed by the remelting process. This process reduces the free radicals which are related to oxidative degradation.
Cross-linking of the PE unit can occur by changes in the amount and method of irradiation and by remelting process. When cross-linking occurs, the properties of PE change and it becomes more stiff and more resistant to the surface wear. Recently, the 3rd generation of highly cross-linked PE (HXPE or X3) has been introduced. Its superiority has been proven in the laboratory tests and in the hip joint prosthesis. However, more experimental studies and more observations are needed for its clinical application in the knee joint prosthesis.
Sterilization and Storage
The properties of PE change according to the method of sterilization and storage. Oxidation occurs during these procedures and long-term survival of PE is affected by oxidative degradation.
If PE is irradiated by gamma rays, the stable polymers become oxidized and carbon is transformed into the reactive form.
This reactive form undergoes one of the following three processes:
1.
Recombination to return to its original state
2.
Chain scission is that the chain breaks to degrade into high-density polyethylene with reduced molecular weight and elastic modulus and increase the wear rate
3.
Cross-linking, in which two polymer chains undergo chemical bonding (Fig. 2.3)
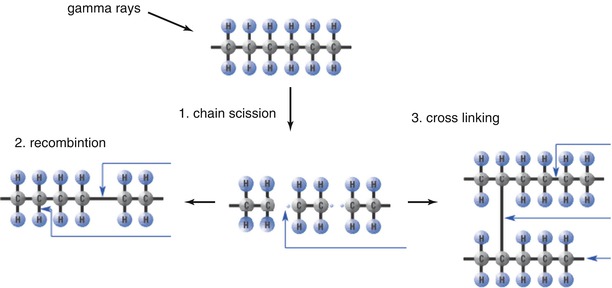
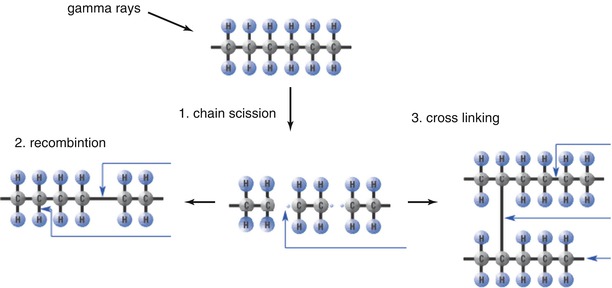
Fig. 2.3
Changes after irradiation of PE
These three processes are affected by the amount of irradiation and the presence of oxygen during sterilization. When PE is irradiated with 3.0 Mrad in the presence of oxygen, cross-linking does not occur and the quality of PE is degraded. When PE is irradiated with 4.0 Mrad in vacuum, cross-linking occurs. Also, when PE is irradiated with 5.0–10.0 Mrad, cross-linking does not occur, but the strength improves. Therefore, most of the manufacturers have used a different method of sterilization to reduce the degradation of PE. They sterilize PE either in vacuum or in argon gas during gamma radiation. Either EO gas (ethylene oxide) or gas plasma sterilization method is also used. MacDonald examined retrieved 20 EO gas sterilized PE and reported that the PE remained stable with respect to both oxidative and mechanical properties for up to 10 years in vivo, and there was no evidence of delamination. However, Medel et al. reported that gamma sterilization in inert gas did not provide PE with a significant improvement in terms of wear resistance as compared with gamma sterilization in air, except for a lower incidence of delamination. However, EO gas sterilization is not effective for metal-backed implants since the penetration of EO gas to the metal is weak. In the gas plasma sterilization method, microenergy and gas are used. According to McNulty et al., this method does not break the polymer chains or generate free radicals that cause PE oxidation. However, gamma irradiation under vacuum is still the most popular method of sterilization.
After storage for a long duration, a white line is formed at about 1–2 mm from the surface of PE and this is a type of aggregation of the fracture line. This white line is closely related to the exposure time to the air and it is a common finding in case of PE that has been stored on a shelf for more than 4 years. Generally, the white line is known to indicate degradation of the quality of products, but some surgeons state that it does not affect the survival of PE implants or incidence of osteolysis in the hip joint. However, Barrack emphasized that this white line can be critical for the knee joints, since PE in the knee joint prosthesis is mostly under shear force as opposed to that in the acetabular cups of the hip joints. Also, oxidative degradation can occur in vivo as time elapses.
Cross-Linking
Cross-linking occurs when the amount of gamma radiation is increased to a little higher level than what is needed for sterilization in oxygen-free condition and thermal stabilization is applied afterwards. More cross-linking makes the PE stiffer and more resistant to abrasive/adhesive wear, but resistance to fatigue failure decreases due to the reduction in elastic modularity.
In the laboratory, the wear decreases dramatically, resistance to fatigue crack propagation and ultimate tensile strength increases, and fracture toughness improves as well. But, a strong resistance to wear means that the size of the wear particles becomes smaller, and these smaller particles are more likely to cause inflammatory reaction and osteolysis.
In the hip joint prosthesis, cross-linked PE has been used for the acetabular cups with promising results, but it has not been proven that this PE has a longer survival rate than the conventional PE in the knee joint. TKA is different from THA in several aspects; the hip joint is a ball-and-socket joint in which the forces are distributed evenly to cause abrasive/adhesive wear, whereas wear of the knee joints is mainly pitting and delamination wear due to eccentric forces and repeated trauma. A stiff cross-linked PE can be more problematic in the knee joint prosthesis. Furthermore, the incidence of loosening of the knee joint prosthesis is increased and a fracture may occur at the post of the PS type or lugs of the patella. Therefore, its use in the knee joint prosthesis is limited to the mobile-bearing joint, and further observation is needed in the fixed-bearing joint.
2.1.4 Bone Cement (Polymethylmethacrylate, PMMA)
Bone cement is the most frequently used material to fix the prosthesis to the bone. It was applied in cranioplasty and for replacement of the head of the femur by Judet. Charnley used polymethylmethacrylate, which has been used in temporary dentures, to fix the femoral stems into the bone marrow cavity.
2.1.4.1 Role of Bone Cement and Its Relevant Theories
The primary purpose of using bone cement is to enhance stability of the implants by increasing the bone–implant contact area and to absorb shock by evenly distributing the load. When the implants are not securely fixed to the bones, they can become loose. Loosening can occur either between the cement and the bone or between the cement and the implant, but it can sometimes occur at both the interfaces.
The factors that are involved in loosening are penetration of cement into the bones, the strength of bone cement, the bonding strength between cement and implants, and the forces transmitted to the implants. The bonding strength is affected by the properties of bone cement, the timing of cementing, the pressure applied to fix the cement, the presence of a foreign body, and the quality of the bone.
The bonding strength between cement and bone is dependent on the micro-interlock obtained by cement penetration into the bone. Miller et al. stated that bonding strength of low-viscosity cement is inferior. If the implant is not securely fixed to the bone, it will cause loosening or accelerate loosening by causing a fracture at the bone–cement interface.
With respect to stability between bone cement and implants, many authors believe that bone cement enhances stability of the implants by positioning the implants in the right location rather than a glue action. Jasty et al. reported the results of a retrieval study of THA which indicates that the first step in loosening is the separation between the implants and bone cement at an early postoperative period and followed by slowly progresses to a fracture at the bone–cement interface and loosening between the bone cement and the bone in that order. Fornasier and Cameron reported five cases of hip retrieval study which demonstrated that the loosening occurred between the bone cement and implants in all cases, and the gap was filled with fibrous membranes. They assumed that the gaps are created by hammering during the curing stage of bone cement, the stem returns to its original size from its expanded size due to the heat generated during the setting of cement, or there is a decrease in bone cement volume by about 2 % after curing.
On the other hand, Davis and Harris emphasized the glue action of bone cement and Ahmed et al. stated that the implants begin to move when they lose their bonding with the bone cement. Bonding force is related to the properties of cement, surface treatment of implants, timing of cementing, and curing temperature. Cho et al. reported the results of bonding strength of CMW (DePuy Ltd. Blackpool, UK) and Simplex P (Stryker-Howmedica-Osteonics, Allendale, NJ) cement to the implants in an in vitro study, which suggested that bonding strength between implant and cement was markedly decreased at 5–6 min after mixing of the polymer and monomer. Pittman et al. reported that bonding is weaker when the surface is smooth and it is stronger when the surface is precoated or roughly finished. In comparison between titanium and cobalt chrome, titanium provided stronger bonds, but the difference was not statistically significant.
Bone cement volume decreases after curing, and bonding strength between the bone cement and implants decreases. So, the greater the change in bone cement volume, the greater the decrease in bonding strength.
A composite unit of bone cement and implants is formed as a result of this bond which enhances the strength of implants. However, it has to be reconsidered whether stronger bonds between the bone cement and implant are clinically desirable, because a stronger bond increases the stress between the cement and the bone causing the cement to loosen from the bones.
Stability is also affected by the forces applied to the implants. It is known that stability of cemented implants is the strongest against compressive force followed by that against shear force and tensile force in that order.
2.1.4.2 Composition
PMMA is a type of polymer similar to PE, polyvinyl chloride (PVC), and polystyrene. It comprises of a series of carbon units whose molecular weight is 100 and has chains with variable lengths (Fig. 2.4).
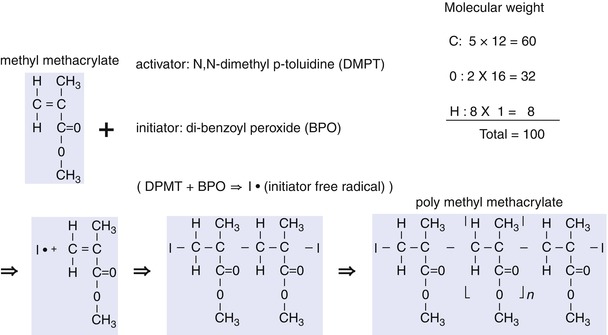
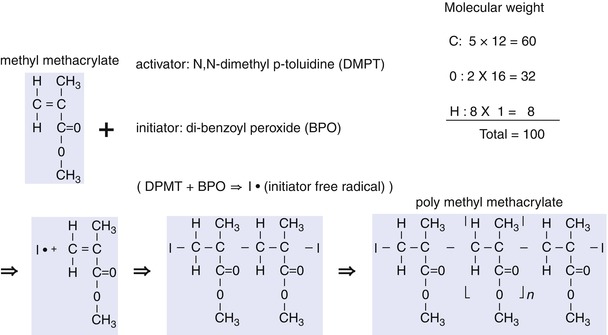
Fig. 2.4
Basic structure of methylmethacrylate and illustration of its activation
The length of the chain varies according to the manufacturing process and the amount of initiator and activator included in bone cement. It is known that with a longer length of the chain, the PMMA molecular weight increases, the properties of bone cement improve, and the viscosity of PMMA increases.
The bone cement packet contains prepolymerized solid particles (so-called polymer) in powder form and liquid monomer. This powder contains copolymer and dibenzoyl peroxide (BPO, BP), which is the initiator. Liquid monomer contains hydroquinone that prevents the monomer from being converted into polymers, along with activator N, N-dimethyl-p-toluidine (DMPT). Each product of prepolymerized powder varies in the grain size, molecular weight, and chain length. When polymerization occurs, PMMA size becomes between 30 and 150 nm and molecular weight becomes between 20,000 and 2,000,000 with an average molecular weight of 170,000.
Barium sulfate (BaSO4) or zirconium oxide (ZrO2) may be added to allow better visualization on X-ray.
2.1.4.3 Heat Generation
When the polymer in powder form is mixed with the monomer in liquid form, the activator (DMPT) in the liquid and the initiator (BPO) in the powder are mixed together to generate free radical initiators (I°), which convert the double bonds of carbon in methylmethacrylate into single bonds for polymerization with the monomers. This process of polymerization generates heat. About 130 cal of heat is generated per 1 g of monomer; and about 2,600 cal of heat is generated per 20 g of liquid monomers.
Heat generation during the operation varies according to the type of bone cement, dispersion of heat to the surrounding tissues, the amount and the thickness of bone cement, and the initial temperature (before mixing). In the laboratory, an average 66–82.5 °C of heat was generated. The temperature reaches 60 °C with the use of 3 mm thick cement and 107 °C with the use of 10 mm thick cement. However, in the operation field, a temperature of 40–43 °C is generated since the metal implants diffuse the heat, the bones are wet, there is blood, and saline is used to cool down the temperature. This temperature is lower than 56 °C at which protein denaturation occurs and is lower than 47 °C at which thermal necrosis of the bones occurs.
2.1.4.4 Stages of Cementing
The stages of cementing can be divided according to the time sequence after mixing. When the powder and the liquid are mixed, the powder and the liquid first form lumps (wetting stage). Wetting of the prepolymeric powder with the liquid monomers generates free radical initiators by the action of BPO and DMPT.
After the wetting stage, the mixture enters the liquid stage. The cement is in a homogeneous liquid form with a relatively low viscosity. In this stage, the mixture can be poured and easily transferred to a cement gun.
Chemical reaction for polymerization begins at this stage and the mixture reaches the dough stage. In this stage, polymers with chains of various lengths are formed in the mixture. In the dough stage, the mixture does not form strings or adhere to surgical gloves, and the polymerized cement volume increases slightly and it generates heat. As polymerization progresses, the mixture undergoes curing (curing stage), and finally, complete setting (setting stage) takes place.
The amount of polymers formed is affected by several factors including the ratio of liquid and powder, the concentrations of BPO and DMPT, grain size, and temperature during mixing. However, it is known that about 1.4 % of monomers remain in the cement even at 4 months after setting, and bone cement becomes even harder as the amount of monomers decreases.
Temperature plays an important role in the speed of polymerization. High temperature accelerates the speed of polymerization. Therefore, it is recommended to cool down the temperature so as to extend the setting time. The setting time is also delayed by about 1 min when humidity is increased by 20 %. On the other hand, the setting time is shortened by vacuum mixing.
The 3–7 min of the dough stage is actually very important in cementing technique and the method of cementing differs according to the viscosity of cement. CMW®-1 (DePuy Ltd., Blackpool, UK) has the highest viscosity, followed by Palacos® (Heraeus Medical, Wehrheim, Germany), Simplex P® (Stryker-Howmedica-Osteonics, Allendale, NJ), and LVC® (Zimmer, Inc., Warsaw, Indiana). Therefore, it is easier to use CMW® and Palacos® with the hands, but not with the cement gun. On the other hand, LVC® has a very low viscosity and is suitable for use with the cement gun. Simplex P® has medium viscosity and may be used with the cement gun (Fig. 2.5).
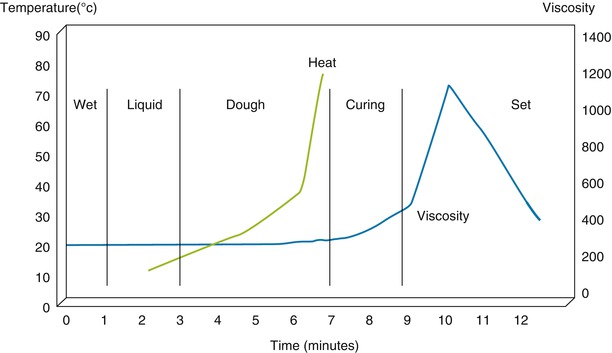
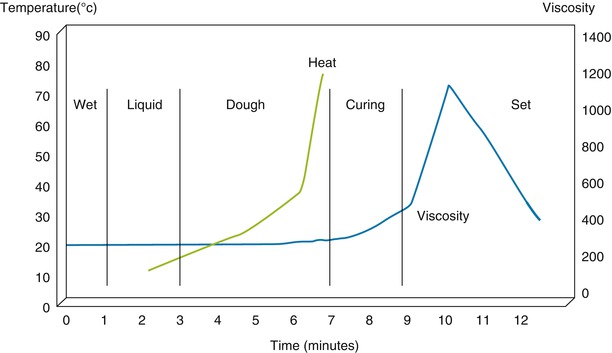
Fig. 2.5
Relationship between heat and viscosity according to the time sequence during polymerization of bone cement (CMW)
2.1.4.5 Strength
The strength of bone cement varies according to the molecular weight, grain size, the ratio of polymers and monomers, method of sterilization, defects and void in bone cement, and foreign materials (barium, antibiotics, etc.) in bone cement.
The strength of bone cement increases with an increase in the molecular weight. In terms of the method of sterilization, gamma radiation lowers the molecular weight and the strength of bone cement is decreased, whereas EO gas does not alter the strength of bone cement.
Vacuum mixing is recommended to increase the strength of bone cement, while the strength of bone cement is decreased by hand mixing since about 10 % of air is mixed during hand mixing. However, Rimnac et al. reported that there was no clinically significant difference in the strength of bone cement when compared between the hand-mixing and vacuum-mixing methods.
Air content decreases in lower viscosity bone cement. Since LVC has a lower viscosity, it has the potential to increase its strength by decreasing the air content; however, it naturally has low strength due to low molecular weight (Table 2.3).
Table 2.3
Factors affecting the strength of bone cement
Condition | Cement strength |
---|---|
Moisture content | 3–10 % ↓ |
Inclusion of blood or tissue(depending on the amount) | Up to 70 % ↓ |
Antibiotic inclusion | 10–15 % ↓ (1:1), 40–60 % ↓ (1:2) |
Radiopaque fillers | 5 % ↓ |
Vacuum mixing | 10–25 % ↑ |
Hand mixing | Up to 21 % ↓ |
Pressurization delay | Up to 40 % ↓ |
Environmental temperature(body temperature) | 10 % ↓ at body temperature thanat room temperature |
The strength of bone cement can be decreased by mixing it with the other materials. Antibiotic-loaded cement is frequently used to prevent or to control the infection. If antibiotics are added in the ratio of 1:1, the cement strength decreases by about 10–15 % with little adverse effects. When antibiotics are mixed in the ratio of 1:2 (one pack cement: two packs or vials of antibiotics), the strength decreases by about 40–60 %.
Creep is another problem associated with the use of bone cement. When bone cement is under a force that is greater than its elastic strain, cement creep occurs and leads to implant subsidence.
2.2 Design
2.2.1 Introduction
There are some guidelines that need to be respected for designing TKA prostheses. In 1913, Freeman and colleagues outlined the following objectives of designing the prosthesis. First, a salvage procedure should be readily available. To achieve this, the bone should be preserved as much as possible so as to leave broad, flat cancellous bones. Second, the chances of loosening should be minimized. In order to do so, the tibial and femoral components should be incompletely constrained in relation to each other. Any hyperextension-limiting mechanism should be progressive and not sudden. The prosthetic component should be fitted to the bone by a means which allows dissipation of the loads over the largest possible area of the bone–prosthesis interface. Third, the rate of production of wear debris should be minimized. In order to do so, the friction between the components should be minimized and the debris produced should be as innocuous as possible. Fourth, possibility of infection should be decreased by reducing the dead space. Fifth, the consequence of infection should be minimized by avoiding the use of long intramedullary stems and intramedullary cement. Sixth, a standard surgical technique should be available. Seventh, the prosthesis should allow knee joint motion from 5° of hyperextension to at least 90° of flexion. Eighth, some freedom of rotation should be resisted. And finally, excessive movements in any direction should be resisted by the soft tissues, particularly by the collateral ligaments. By following these guidelines, knee joint function can be preserved as much as possible and the survival of TKA can be extended by reducing the complications.
The design of the knee prosthesis is largely based on two concepts: the first is the design focused on anatomy and the second is the design focused on function. In the anatomical design, the normal anatomy is preserved as much as possible and the prosthesis is designed similar to the anatomy of the knee joint. In the functional design, an attempt is made to improve function by preserving kinematics of the artificial knee joint. This can be achieved by simplification of biomechanics of the knee joint and by sacrificing some of the anatomy. However, the current prostheses are designed considering the concept of both the anatomy and function.
In order to improve the knee joint function, the key factor to be considered in TKA prosthesis is “to what extent the anatomy should be reflected on the design.” The anatomy cannot be ignored, but every knee joint is different and the anatomy of the knee joint is altered during the operation. Since the anatomy of the knee joint is already altered due to the operation, the changes in biomechanics of the knee joint should also be considered. As the anterior cruciate ligament (ACL) or both the anterior and posterior cruciate ligaments (ACL and PCL) and both menisci are already removed during the operation, the kinematics of the artificial knee joint is quite different from those of normal joint, for example, the four-bar linkage concept does no longer exist.
Therefore, the most desirable prosthesis design for knee joints is the design in which the changes in the geometry and biomechanics of the knee joint are taken into consideration, and at the same time, it should also be compatible with the altered knee joint mechanics and should be more forgiving.
However, there has been a recent resurgence in the trend to preserve normal knee kinematics in TKA prosthesis design in an attempt to improve function and lessen anterior knee pain. One of the most important anatomical structures of physiological kinematics is both the cruciate ligaments. Based on this concept, there are some prostheses which retain both the cruciate ligaments.
2.2.2 Anatomy and Biomechanics
In the past, the anatomical and biomechanical studies concerning the design of artificial joints were accomplished with primitive biomechanical studies under static conditions, cadaveric structural study, and gait analysis. But these methods were not accurate as they did not provide dynamic information regarding biomechanics and only provided indirect and two-plane information. Currently, with the advancements in electronic technology and computer system, more accurate and detailed information can be obtained through fluoroscopy, CT, MRI, stereoscopic study, etc.
The biomechanical study of TKA is mostly concerning A–P translation, rotation, flexion–extension motion, and condylar lift-off. Since these motions are interrelated with each other, it is very difficult to interpret the results when all of them are considered together, as there can be countless combinations. Furthermore, the results of this mechanical study can be changed according to the status of weight bearing. When the knee joint is not loaded with the weight, it follows the path of least resistance of motion. When the knee joint is loaded with the weight, its motion is affected by muscle interactions, soft tissue tension, soft tissue impingement, and articular congruity.
The A–P translation is often expressed as rollback and it greatly affects the ROM. It is known that normal translation is about 19.2 mm on the lateral side and 3.4 mm on the medial side. This is different from the traditional concept which implies that the degree of translation is uniform on both the sides. There are other reports suggesting that the rollback is less on the medial side before 120° of flexion as described above, but the rollback is equal on both the sides between 9 and 10 mm after 120° of flexion. The amount of rollback varies according to the existence of cruciate ligaments. The rollback clearly decreases even when only the ACL is removed. Therefore, not only the PCL but also the ACL is involved in rollback and this is one of the reasons why the range of motion after TKA becomes less than that in the normal joints. The theory that only the PCL is involved in rollback could have been derived from the studies which are focused on the role of the PCL, because the ACL is removed in all the cases during arthroplasty and there is a need for comparing the CR and PS type of implants. However, rollback does not occur in all cases and the so-called paradoxical rollback, in which the femoral part is pushed forward, may occur due to the design defect or surgical failure. When paradoxical rollback occurs, soft tissue impingement develops, and the patellofemoral mechanism becomes overstuffed causing a reduction in flexion motion and provokes rapid increase in the PE wear.
In flexion–extension motion, the axis of motion is known to have a J-shaped inconsistent center, but the current theory that it is fixed around the transepicondylar axis is more convincing. Rolling and sliding occur during flexion. Rolling occurs during the first 20–30° of flexion and then sliding occurs thereafter in a 1:2 ratio. This has a critical impact on the range of motion and PE wear.
In the rotational motion, the tibia rotates internally by about 5.7° when the knee is bent during normal walking and displays up to 15° of internal rotation in maximum flexion when the weight is not loaded. The pattern of rotation on the medial side is similar to that in the ball-and-socket type of joint and that on the lateral side is just like two round boards articulating along the medial axis. However, as has already been mentioned regarding the rollback, the medial condyle moves about 3 mm in physiological state and the medial plateau translates a little back and forth (Fig. 2.6). The degree of rotation after TKA is less than normal, no matter whether or not the PCL is present. In a mobile-bearing joint, it is known that the mechanism of rotation is similar to that in a normal joint, but the mechanism of rotation in a fixed-bearing joint is different from that in a normal joint. However, these biomechanics are more complex when rollback and rotation are considered in combination, and the biomechanics clearly vary greatly from product to product when the PCL has been removed.
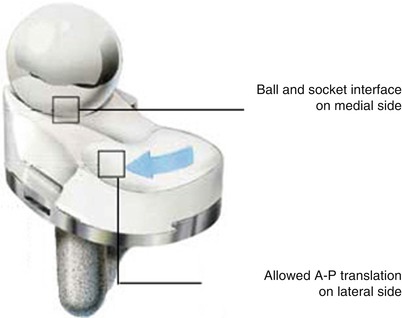
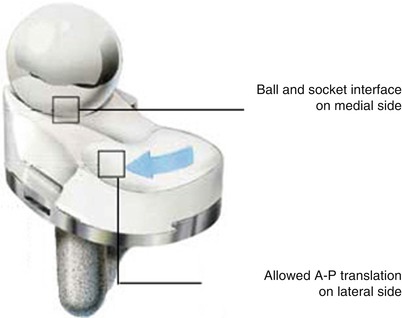
Fig. 2.6
Movement of contact surface of the tibial implant and the femoral implant (ADVANCE, Wright Medical Technology, Arlington, TN)
Condylar lift-off also affects the survival of the prosthesis (Fig. 2.7). When it occurs, stress concentration develops at the compressed site inducing early wear. Condylar lift-off generally occurs on the lateral side at maximum flexion, but it occasionally occurs on the medial side. Condylar lift-off is significantly influenced by the existence of cruciate ligaments and is related to the attachment site of the cruciate ligaments to the femur. The ACL attaches to the inner side of the lateral condyle, so it acts as the checkrein for lateral lift, whereas the PCL attaches to the inner side of the medial condyle and prevents lifting of the medial condyle. Therefore, lift-off generally occurs at the lateral condyle when the PCL is retained.
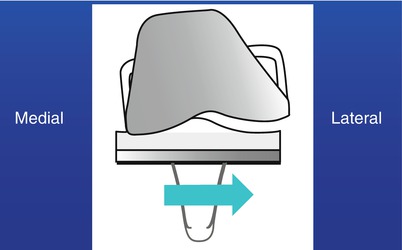
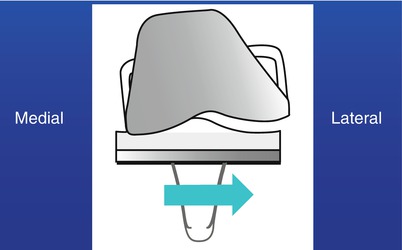
Fig. 2.7
Condylar lift-off
In addition to these biomechanical studies, a simulator is used mainly for the study of survival of the implant. This simulator is designed to produce mechanics similar to that of the knee joint. The data obtained from the simulator are design specific and may be different according to design of the simulator.
In spite of these experimental studies, the clinical results may be somewhat different from the in vitro data. Hence, the implant design should follow the clinical results based on the laboratory experiment.
Banks et al. used the image-based measurement technique and reported the results of a few mechanical studies of TKA. The results of their study were different from the preexisting theories in several aspects. First, because the femur is bent forward and the tibia is sloped posteriorly, a usual osteotomy would cause an average 9.5° of knee hyperextension compared to the anatomical axis of the tibia and the femur in the sagittal plane. This is in stark contrast with the basic concept of conventional TKA. Hyperextension causes many problems such as anterior impingement when the knee is fully extended and excessive stress on the post in the PS type of implant. Moreover the results of mechanical studies of TKA may be different from the real situation because nearly all the current prostheses have been designed, assuming that the alignment of implants is identical to the alignment of bones in the sagittal plane. Second, it is true that femoral rollback increases the amount of flexion. However, in the dynamic study of walking, the femur would translate forward in flexion and backward in extension when the ACL was sacrificed and meniscectomy was done. In case of the CR type of implant, if the flexion gap is too wide, a paradoxical rollback would occur that could cause reduction in flexion motion. Third, posterior condylar geometry is closely related to the range of flexion. The range of flexion increases with an increased posterior condylar offset (the maximal distance between the posterior femoral cortex and the posterior condyle of implant: Fig. 2.8); when posterior condylar offset increased, the flexion angle increased. Unlike the assumption that using a smaller implant in the anterior referencing system would widen the flexion gap and increase the range of flexion, it would decrease the posterior condylar offset to decrease the range of flexion. Fourth, in the fixed-bearing type of implant, the tibia rotates internally with the rotational axis along the medial condyle during flexion as has been shown on biomechanical study. Whereas in the mobile-bearing type of implant, the medial femoral condyle would be pushed forward and may not act as the axis of rotation.
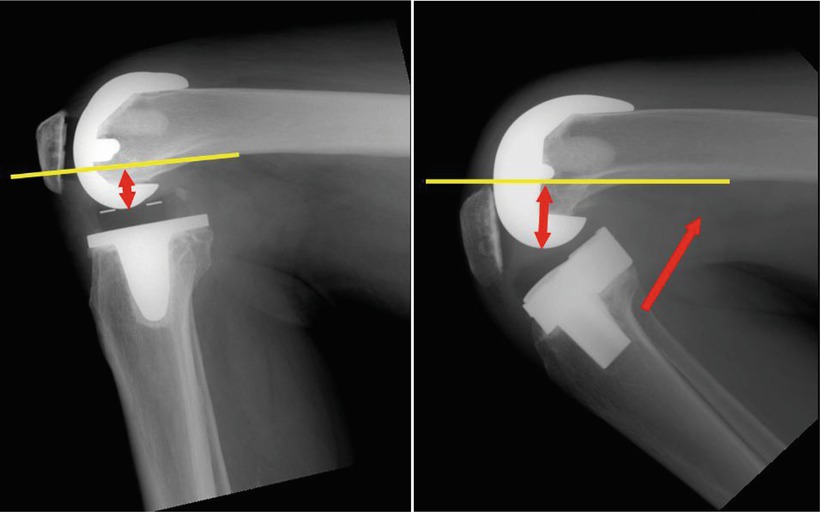
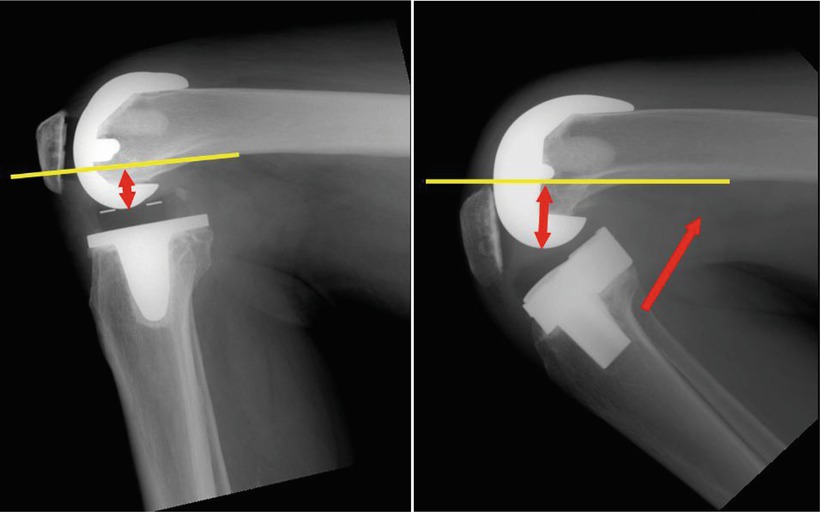
Fig. 2.8
Posterior condylar offset (Left: short offset, Right: long offset)
2.2.3 Factors to Be Considered in Implant Design
Most implant designs focus on the structural congruency, motion constraint between the components and the method of fixation. All these factors are mutually related in various aspects.
2.2.3.1 Congruency Between Components
Congruency is closely related to wear and loosening, which are the most critical complications of TKA. With good congruency between components, for example, a round PE would increase the contact surface area reducing stress on the PE and decreasing the incidence of wear, while stress between the bone and the prosthesis interface would increase the incidence of loosening as a result. With lower congruency between components, for example, a flat PE would decrease the incidence of loosening at first, but wear would be accelerated and wear particles may cause osteolysis and further loosening (Fig. 2.9 ).


Fig. 2.9
Effect of congruency of TKA design. The left image shows lower congruency and the right image shows higher congruency. The load is evenly distributed in the right side. Stress is broadly dispersed in higher congruency (Right)
Historically, the first modern prosthesis designs of TKA were focused on the stability of implants through increasing congruency, but many problems associated with loosening occurred. In order to solve this problem, congruency was reduced thereafter by sacrificing some amount of stability. However, a reduced congruency increased the incidence of wear and wear particles further accelerated loosening. Currently, most of the designs focus on maintaining the appropriate structural congruency.
2.2.3.2 Motion Constraint Between the Components
Motion between components needs to be balanced with stability. Generally, increased motion of implants reduces the stability whereas an increase in stability decreases the range of motion.
The implants of artificial knee joint are classified into unconstrained, semiconstrained, and constrained designs according to the amount of motion restriction between each component. There is neither unconstrained nor constrained type of implant in a strict sense, but the unconstrained type of implant allows for more motion between the femoral and tibial components, and the mobile-bearing joint is included in this category. On the other hand, any implant that strictly restricts valgus–varus motion could be classified as a constrained type of implant. The constrained type of implant generally provides varus–valgus constraint and a few allow some rotational motion. Most of the prostheses which are not included in the unconstrained and constrained categories are classified as the semiconstrained type of implants.
The selection of implants according to motion constraint must be based on the condition of the knee joints. Generally, it is desirable to choose a design that allows less motion constraint and provides an appropriate level of stability. If an implant constrains motion to a great extent, it can increase the incidence of loosening. Whereas when instability is overlooked and an implant that allows less motion constraint is used, giving way or dislocation may develop.
Unconstrained Type
In TKA, the unconstrained type allows for motion between the PE and tibial components. Mobile-bearing joint is included in this category of implants, which allows A–P translation and rotation of PE placed on the tibial plate.
History
The first mobile-bearing joint was the Oxford Knee which was introduced by O’Connor as a concept of compartment replacement in the 1970s. In the Oxford Knee, the femoral condyle was designed in a spherical shape so as to retain both the cruciate ligaments.
Following this, the most popular mobile-bearing LCS (low-contact stress) New Jersey Knee was developed (Fig. 2.10).
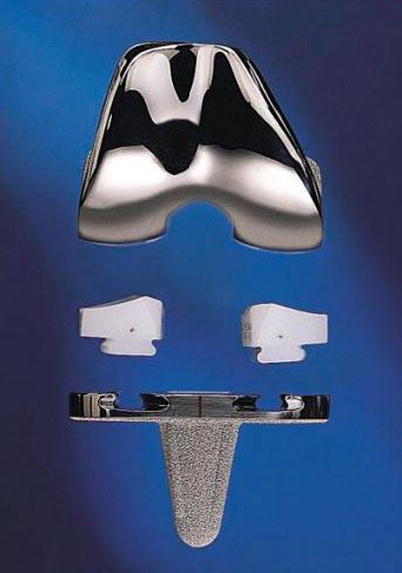
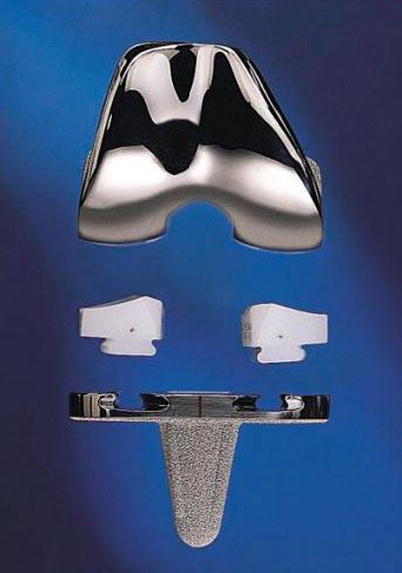
Fig. 2.10
Early form of the LCS meniscal-bearing joint (DePuy, Warsaw, IN)
Theoretical Background
The theoretical background of mobile-bearing joint is to reduce the wear and loosening, which are the most common complications affecting the survival of TKA. Wear can be reduced by increasing conformity to broaden the contact surface between the femur and PE. But the resultant decrease in motion between the femoral component and PE imposes an excessive stress at the implant–bone interface that accelerates loosening of the components. If the PE is designed to move, stress at the implant–bone interface would be lowered, thereby decreasing the incidence of loosening (Fig. 2.11).
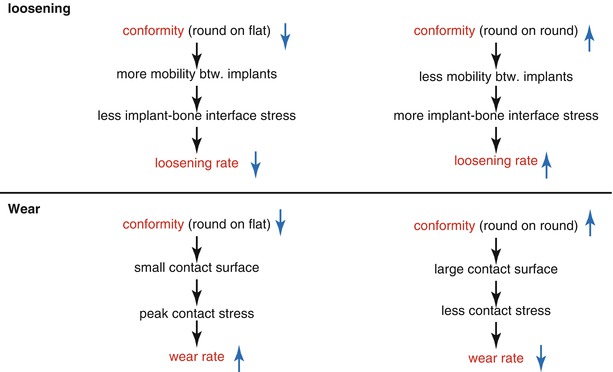
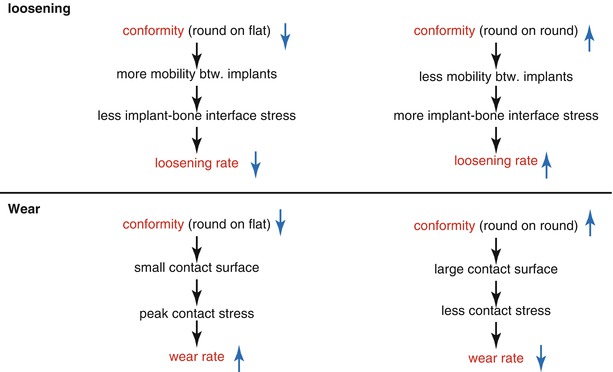
Fig. 2.11
Relationship between conformity and wear/loosening
However, in a mobile-bearing joint, there is a possibility of dislocation due to excessive motion of PE and breakage of PE due to increased conformity. Stability of the implants is a key factor in preventing dislocation of the mobile-bearing joint, and it relies on articulating geometry and ligament balance.
To increase stability, there are some differences and modifications of design in mobile-bearing joint. Considering the pattern of articulation, single-radius design of femoral component is often used to increase the stability. A large single radius can push the bearing component too far back to cause dislocation in flexion/extension. So, the radius of the posterior condyle is decreased to align the anatomical axis with the axis of implants in the sagittal plane so that the anatomical axis can be at the center of the bearing component in flexion/extension (Fig. 2.12). The tibial component is also designed to control A–P translation to prevent the PE from translating too far back so as to reduce the incidence of dislocation. The ligament balance greatly affects the stability of mobile-bearing joint, so the earliest form of mobile-bearing joint was designed to retain both the cruciate ligaments. After a while, the ACL-sacrificing and both ACL- and PCL-sacrificing implants were introduced. In case both the anterior and PCLs are sacrificed, only axial rotation is permitted so that the bearing component is stabilized at the center. The patella component is designed to ensure stability and to improve tracking by its anatomical shape.
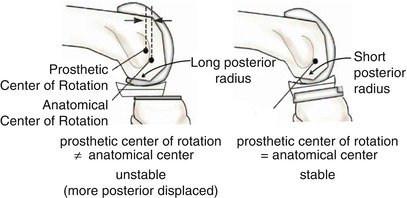
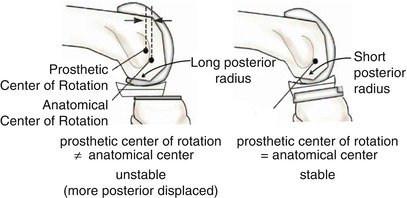
Fig. 2.12
Comparison of the femoral implants according to the radius of the lateral condyle in single-radius femoral implants. The left image shows a larger radius and the right image shows a smaller radius (reducing the radius of the posterior condyle can align the axis of flexion and extension of artificial joint to the real knee joint as much as possible so as to decrease lateral dislocation)
Breakage of PE occurred frequently when a sharp edge for increased conformity was combined with malrotation, when condylar lift-off developed or when a PE defect was noted. Therefore, the overall implant design has gradually improved. The more improved designs have edges that are not too sharp and excessive motion is constrained so as to reduce the condylar lift-off.
Types
Mobile-bearing joint is classified according to the method of PCL treatment into posterior cruciate-retaining (PCR), posterior cruciate-sacrificing (PCS), and posterior cruciate-stabilized (PS) type.
The first design of PCR implant had two separate bearing components (Fig. 2.13), but the current design has one meniscal bearing. It is designed to move anteroposteriorly and rotation is controlled to a small extent (Fig. 2.14). This movement is similar to the normal meniscal movement so as to facilitate load distribution and more flexion. The PCS type allows for rotation only (Fig. 2.15). The PCS type was modified into the PS type by the addition of cam and post mechanism.
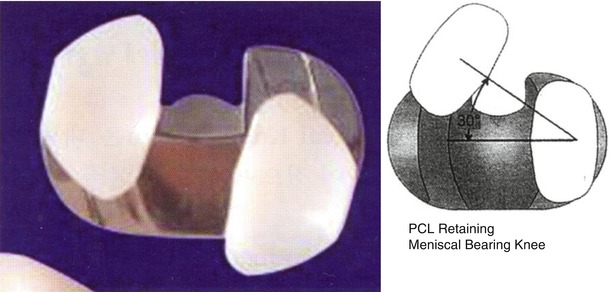
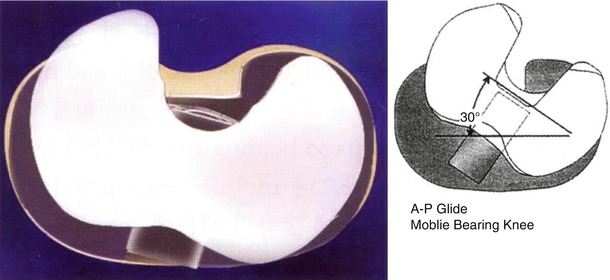
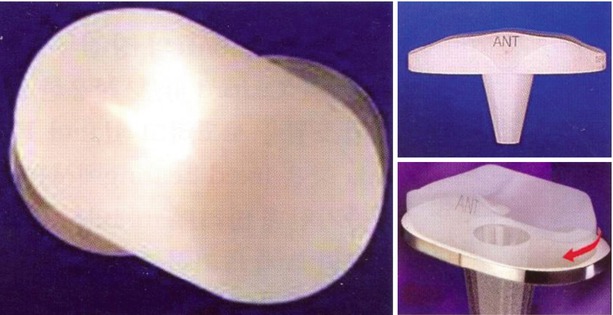
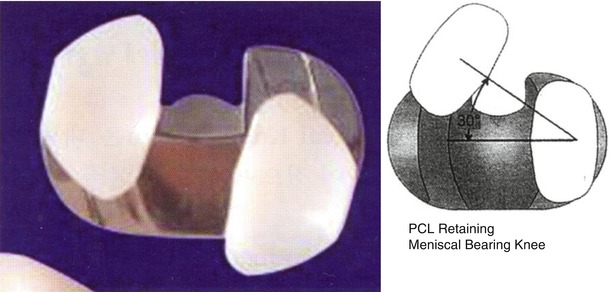
Fig. 2.13
Earlier form of meniscal-bearing knee that retains the posterior cruciate ligament in the LCS prosthesis (DePuy, Warsaw, IN)
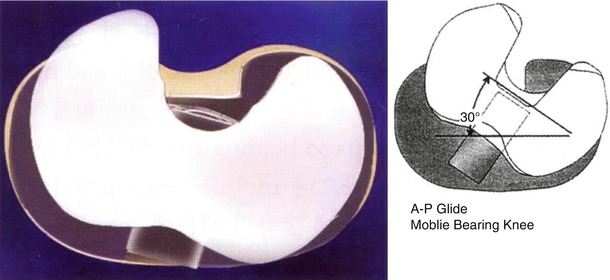
Fig. 2.14
The current A–P glide design of knee prosthesis (DePuy, Warsaw, IN)
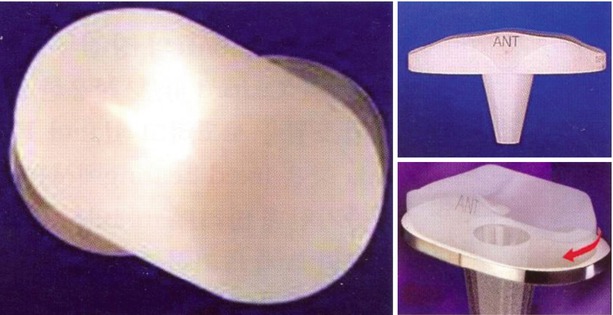
Fig. 2.15
Posterior cruciate-sacrificing LCS prosthesis (DePuy, Warsaw, IN)
Advantages of a Mobile-Bearing Joint
Menchetti and Walker found in a study of mobile-bearing joint using the Oxford jig that it reduces the wear by uniformly reducing stress on the PE, and Sharma et al. also reported almost similar results in an in vivo study. Many authors have reported an average wear rate of 0.09–0.36 mm/yr in mobile-bearing TKA, which was comparable with the wear rate of 0.20 mm/year in THA. Based on a retrieval study, Ho et al. reported that the wear was less in the mobile-bearing joint.
The greatest advantage of mobile-bearing joint is that the malrotation can be corrected automatically and normal knee joint biomechanics can be maintained by the free motion of the bearing component. In normal knees, the tibia rotates externally during the last phase of extension of the knee joint, and a mobile-bearing joint enables smooth rotation similar to that in the normal knees (Fig. 2.16). However, a fixed-bearing joint does not allow tibial rotation biomechanically.
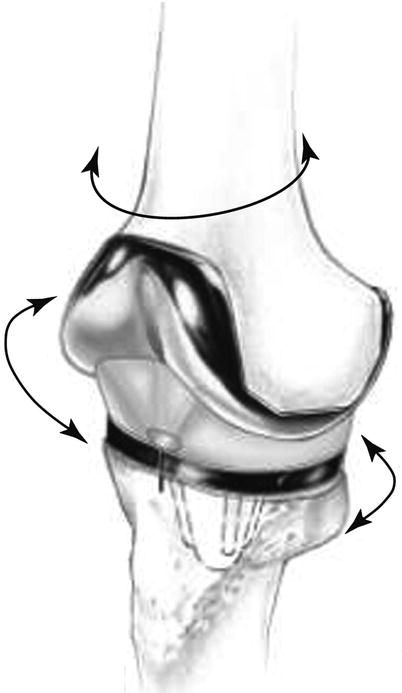
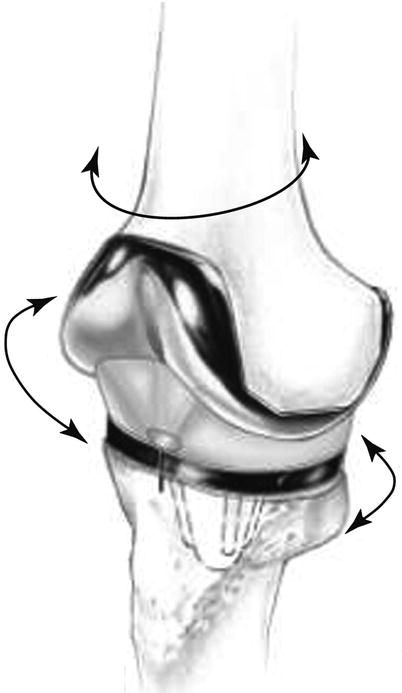
Fig. 2.16
Direction of motion of the mobile bearing knee prosthesis. It shows action similar to the native knee joint biomechanics in flexion–extension, axial rotation, and anterior–posterior translation
When there is malrotation, an excessive force is imposed on the PE and it causes rotational or mediolateral imbalance. A rotating platform design, through a rotation of the bearing, provides for greater self-correction of the component malalignment and allows better centralization of the extensor mechanism. When the tibial component was malrotated in the fixed-bearing joint, Matsuda et al. proved that stress on the PE was increased, whereas stress on the PE in the mobile-bearing joint was maintained constantly since malrotation could be corrected automatically (Fig. 2.17). These effects also decrease the stress between the implants and bone interface, thereby reducing the incidence of loosening. All rotating platform knee designs, however, are not the same kinematically. The kinematics of PE-bearing rotation is design specific and is quite different from products to products.
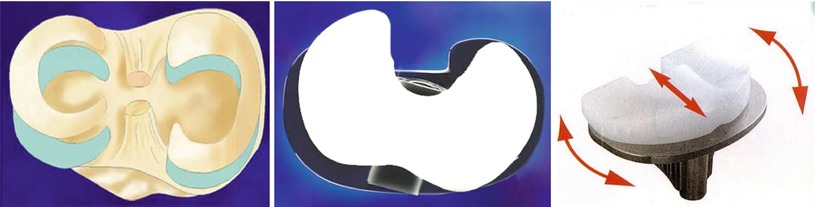
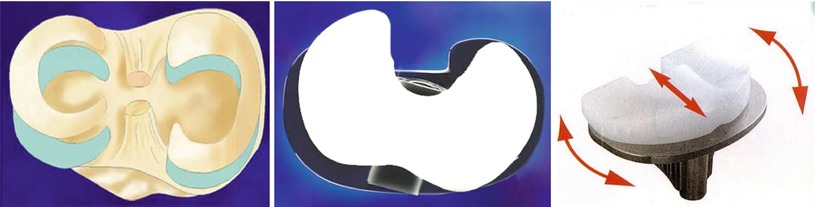
Fig. 2.17
How rotation is automatically corrected in mobile bearing knee prosthesis (DePuy, Warsaw, IN)
Sawaguchi et al. reported that patellar tracking is better in mobile-bearing joint, while Cho et al. reported that there was no difference in patellar tracking between mobile-bearing joint and fixed-bearing joint.
Disadvantages of a Mobile-Bearing Joint
The stability of mobile-bearing joint depends on the function of the surrounding ligaments. If these ligaments are not balanced, they cause instability. So, careful ligament balancing during the operation is required for a mobile-bearing joint. For this purpose, osteotomy is performed first in the tibia and the gap technique is used for femoral osteotomy.
Stiehl et al. reported the results of the mechanism of femoral rollback stating that 5.8 mm of rollback occurs in 0–30° flexion in the normal knee joints, whereas the PCR-type mobile-bearing joint shows excessive rollback of about 9.2 mm in the same range of flexion, and the tibial portion is translated anteriorly in 60–90° flexion. If tension on the PCL increases, posterior soft tissue impingement occurs causing paradoxical anterior translation instead of rollback even though the flexion range is increased. This may cause limited flexion and disturbance of the extensor mechanism of quadriceps muscles.
Condylar lift-off may also occur on both the sides, thereby increasing the wear rate.
There was a report stating that the peak stress on the mobile-bearing joint in 60° flexion was nearly the same as that on the fixed-bearing joint, which was quite different from the theoretical advantages of the mobile-bearing joint.
Wear can be divided into articular wear and undersurface wear according to the site, and both surface wear may increase in the mobile-bearing joint. Regarding the pattern of wear, Collier et al. reported that the LCS mobile-bearing prosthesis mostly showed burnishing which was a kind of abrasive/adhesive wear, whereas the fixed-bearing prosthesis mostly showed cracking and delamination wear. Kelly et al. reported that each bearing surface in mobile-bearing TKA was damaged to a similar extent as that in a fixed-bearing implant. Lu et al. reported that the articular surface wear was reduced, but the undersurface wear was increased. However, Barrack stated that the tibial component of mobile-bearing joint had good surface treatment causing less wear than that in the fixed-bearing joints.
Also, there are concerns regarding the wear particles that are generated in mobile-bearing joint. The wear particles are smaller and these smaller wear particles are more likely to cause osteolysis than the larger particles. In addition, polyethylene wear particles can get caught between the components and can cause problems such as third-body wear.
Another criticism is that little rotation of bearing actually occurs as shown in some kinematic studies. Wasielewski et al. reported that rotation was the best in the PS type of mobile-bearing prosthesis and it was only an average of 3.8° and reverse rotation to biomechanics also occurred in 17–32 % of cases. McEwan et al. reported that the screw home movement was variable, and it was not a physiological movement in the mobile-bearing joint.
Results
Good to excellent results have been reported by many authors. Kaper et al. reported a 94 % satisfaction rate during the midterm follow-up at 5 years or more, and Buechel et al. reported a 95 % or higher satisfaction rate during the long-term follow-up at 10 years or more and a 10-year survival rate of 97 %. Sorrels et al. reported excellent outcomes with a 13-year survivorship of 93 %. Carothers et al. reported the results of an average 8.6-year follow-up through meta-analysis of 3,506 cases of mobile-bearing TKA, which demonstrated that excellent results were obtained, and loosening and bearing instability were uncommon. However, comparing the fixed-bearing and mobile-bearing joint, Evans et al., Cho et al., Aglietti et al., and Mahoney et al. reported that no difference was found in the clinical results or survival rate between mobile- and fixed-bearing joints. But these results were quite different among the implants used, especially in the mobile-bearing joint.
By comparing between the PCR and PCS type of prosthesis, Stiehl et al. demonstrated that the PCR type showed better functional results, whereas the PCS type showed a better survival rate. Kim et al. reported that the PCS type is superior in terms of the ROM and patient satisfaction. In comparison between the cemented and cementless type of prosthesis, Hartford et al. reported a higher incidence of loosening in the cementless type.
In terms of complications, spinout is a specific complication to the mobile-bearing joint, and its incidence was reported to be between 0.5 % and 9.3 %.
Author’s Opinion

The concept of mobile-bearing knee joint is theoretically very sound and postoperative patient satisfaction is also very high. Some surgeons complain that it is difficult to perform the operation, but once accustomed to handle this system, it takes a shorter time than that for the fixed-bearing joint.
The key point of the operation is to balance the soft tissues. In this respect, I would recommend not to use a mobile-bearing joint in cases with severe deformity and an unstable joint.
In my opinion, selecting the right size of implant is also very important for ensuring stability. If the implant is too small, the joint space is widened causing dislocation of the implant.
I believe that proper selection of the size of the implant and a successful operation would help to ensure joint stability, increase the ROM, and reduce the incidence of wear and loosening, especially in the relatively younger patients.
Semiconstrained Type
Semiconstrained types are all fixed-bearing joints and are currently the most commonly used type of implants. Semiconstrained implants vary in shape and size based on how well they reflect the anatomy and biomechanics of the knee joint and how much stability and ROM of the knee joint can be achieved.
TKA implants have developed a lot so as to improve motion, stability, and survival, based on the anatomy and biomechanics of the knee joint. Constrained implants were developed early in the history of evolution of TKA and were followed by development of semiconstrained type of implants.
The most epoch-making event was the development of PCL-substituting type of implants. Most of the earlier semiconstrained implants were designed such that the ACL could be removed, but the PCL should be retained (so-called cruciate-retaining (CR) type). Soon thereafter, Total Condylar Knee prosthesis was developed for use in cases when the PCL was missing or weak. The term “total condylar” means that one component covers both the condyles of each bone. Total Condylar Knee prosthesis has two components covering the femur and the tibia respectively, and the basic concept is surface replacement. To achieve implant stability, the posterior lip of the PE was enhanced. But it caused limited motion and dislocation. To solve these problems, posterior-stabilized knee prosthesis was developed in 1978, and it showed good clinical results despite the fact that many were worried about the poor results initially. Since then, the PCL-substituting (PS) type of implants has been developed by modification of posterior-stabilized knee and is used by many surgeons even in cases which there were no problems with the PCL (Fig. 2.18).
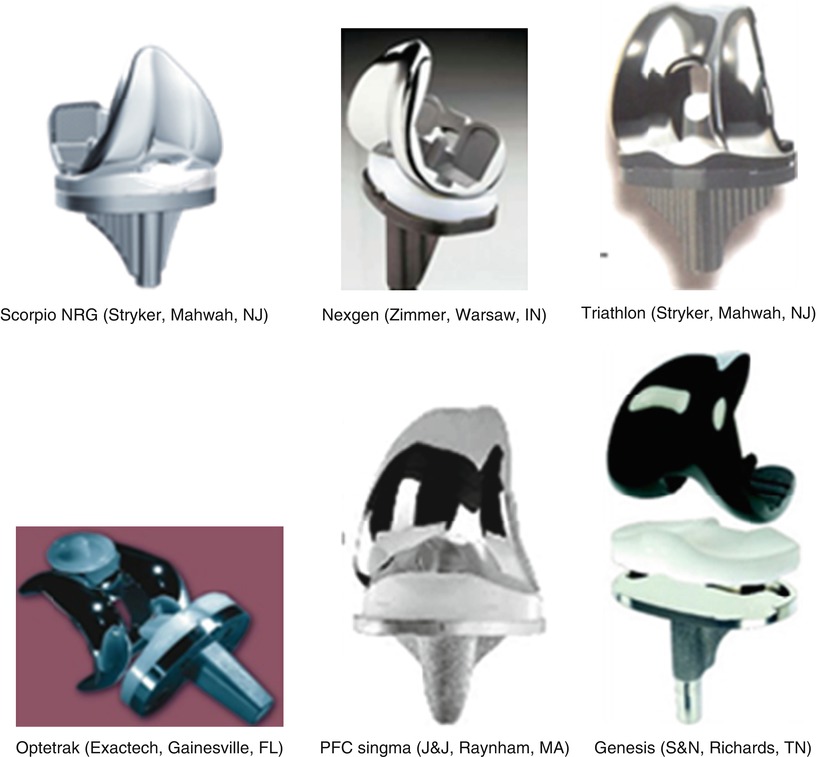
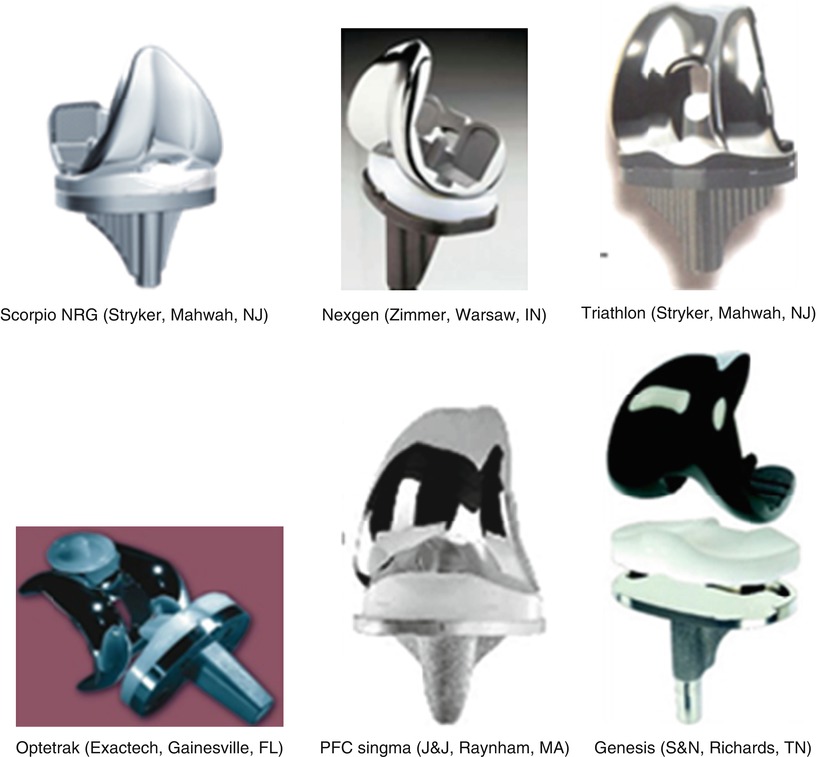
Fig. 2.18
Various kinds of semiconstrained type of implants
Posterior Cruciate-Retaining Type (CR Type)
Posterior cruciate-retaining type of implant was developed to maintain the normal kinetics of the knee joint as much as possible by preserving the kinematic function of the PCL. The function of the PCL is to allow femoral rollback, improve the mechanical efficiency of the extensor mechanism, act as the axis of rotation, increase anteroposterior and varus–valgus stability of the knee joint, and contribute to proprioception. Besides, the PCL can reduce stress between the components and between the component and the bone by transferring the load to a functional ligament, thereby decreasing the wear and loosening.
In terms of joint line, the CR type is better for maintaining the joint line than the PS type. Maintenance of the anatomical joint line is potentially valuable because patellofemoral dysfunction remains the main cause of many unsatisfactory TKAs. Mihalko et al. proved the presence of mechanoreceptors within the ligament by immunohistochemistry techniques using S100 protein and neurofilament protein (NFP) and stated that the proprioception was better by preserving the PCL. Zhang and Mihalko found positive stained elements with both S100 protein and NFP immunohistochemical staining form the specimen of PCL in 5 CR-type retrievals, which had been in place 5–12 years. Another advantage of the CR type in comparison to the PS type is that more bone is preserved in the CR type.
However, there are many surgeons who are not in favor of the use of the CR type. Fluoroscopic studies by Stiehl et al. and Dennis et al. have demonstrated that the CR prostheses do not replicate the kinematics of the normal knee joint. Instead, paradoxical roll-forward occurs due to improper tensioning of the PCL in many cases. Hence, maintaining the appropriate tension of the PCL is important. If the PCL is too tight, it causes excessive rollback to increase the wear of the posterior portion. This movement is demonstrated in the “slide-back” test, which is tested by pushing the tibia posteriorly. If the PCL is too loose, it causes roll-forward to increase the wear of the anterior portion and the result is the same as that with the use of early type of Total Condylar Knee prosthesis. Therefore, the CR type is recommended only when the PCL is functioning normally and in the absence of severe deformity, bone loss, or instability.
The PE had to be flat for the CR type to induce rollback and to prevent impingement between the PE and the PCL, but a flat PE accelerated the wear and caused poor patellofemoral alignment (Fig. 2.19). Currently, the designs have evolved and a flat PE is not used even in the CR type. In other words, PE is designed to be round in shape to maintain the appropriate tension so that the PCL can adjust to this change of the PE. Some other problems noted with the past implant designs and materials have been corrected and improved. These include improvement in the patellofemoral mechanism, modularity, locking mechanism between PE and tibial plate, the back side wear, and improvement in manufacturing and sterilization of PE.
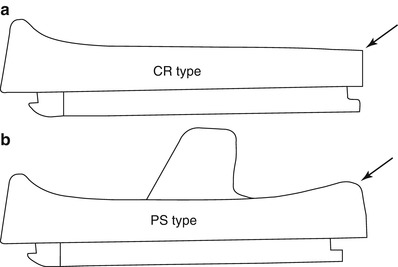
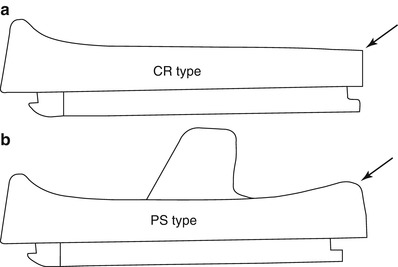
Fig. 2.19
Difference in the shape of the PE in CR and PS type. (a) Posterior end of PE (arrow) is flat to accommodate posterior cruciate ligament, (b) PS type has rounded posterior end (arrow) for better conformity
Posterior Cruciate-Sacrificing Type
History and Terminology of Posterior Cruciate-Sacrificing Type
The implants used when the PCL has been removed can be called Posterior Cruciate Sacrificing (PCS), posterior cruciate-stabilizing, or posterior cruciate-substituting prostheses. These terms are used interchangeably although they have a little different meaning. It is necessary to define these terminologies.
PCS type of prosthesis literally indicates that the PCL has been sacrificed. It can be divided into the non-substituting and substituting type according to the functional restoration of PCL after removal of the PCL.
Types of Posterior Cruciate-Sacrificing Design
Posterior Cruciate Sacrificing-Non-substituting (PCS) Type
This type of prosthesis has no cam and post mechanism after sacrificing the PCL. Total condylar type of implant was developed in 1973 and it was the first non-substituting type and first patellar resurfacing type of implant. So, it can be classified as PCS non-substituting type of implant. This type of implant was designed to improve the A–P stability by increasing articular conformity, and the collateral ligaments played an important role in the overall implant stability. However, it could not induce femoral rollback during flexion motion and the range of motion was not more than 90°. Also, subluxation or dislocation occurred easily with the use of this type of implant due to instability when the ligaments were not balanced.
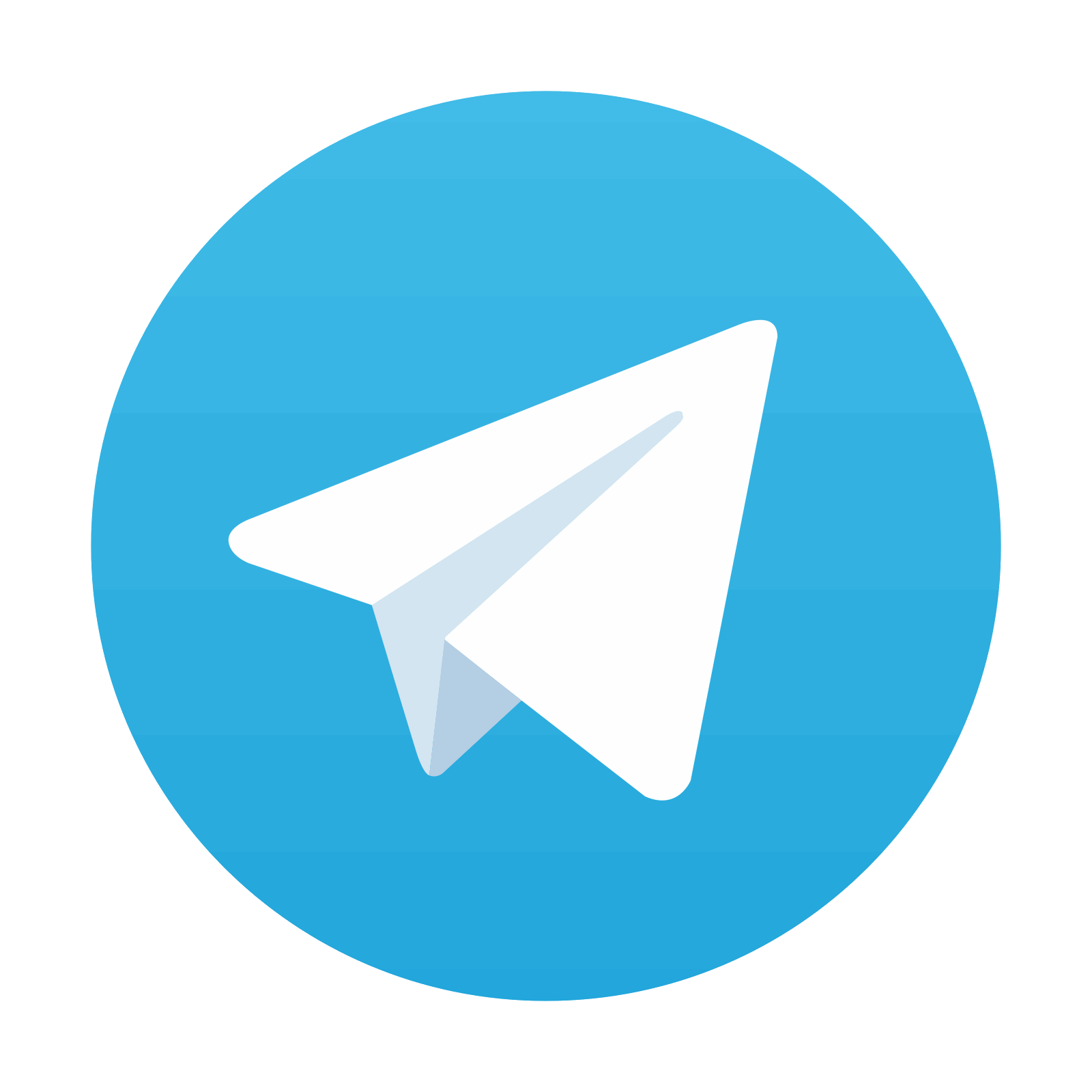
Stay updated, free articles. Join our Telegram channel

Full access? Get Clinical Tree
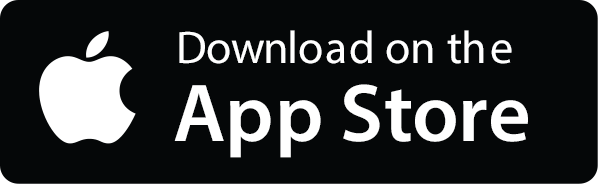
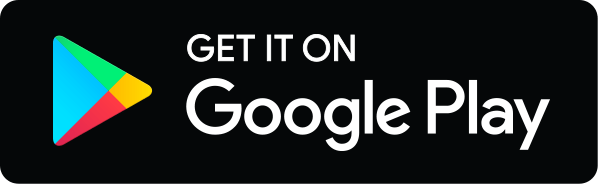
