Impaction Grafting of the Acetabulum
B. Willem Schreurs
Wim H.C. Rijnen
Pieter Buma
Nico Verdonschot
Jean W.M. Gardeniers
Many total hip arthroplasties, cemented and cementless, fail because of aseptic loosening. This often results in loss of bone stock. One of the major key challenges in revision surgery is how to manage the periprosthetic bone loss. Although controversy still exists about the best treatment for bone stock deficiencies, this chapter presents an attractive biologic method that uses impaction allografting with cement.
Case Example
A 45-year-old woman presented with pain following a cemented revision total hip arthroplasty performed 11 years ago. Her primary total hip was done at the age of 19 years following a native septic arthritis. She reports increasing pain and shortening of the left leg. Physical examination shows an antalgic gait, restricted range of motion of her left hip joint, and a left leg that is 4 cm short. There is substantial atrophy of her left gluteal muscles. An anteroposterior radiograph of the total hip arthroplasty shows aseptic loosening and bone loss on both the acetabular and femoral sides (Fig. 112.1A). Laboratory values and a nuclear scan showed no evidence of infection.
History
Reconstructive bone surgery is an ancient art. Animal bone tissues were already used as transplants in Hippocrates time. According to Mankin (1), the legend called “The Miracle of the Black Leg” is considered to be based on the first known allograft procedure in humans. In 1668, Job van Meekeren (2), a Dutch surgeon, reported the first bone grafting procedure, and Anthony van Leeuwenhoek (3), a Dutch scientist, first described the microscopic structure of bone. The popularity of using autogenous grafts and allografts in clinical practice has waxed and waned. During the latter half of the nineteenth century and the early part of the twentieth century, well-known surgeons such as Ollier (4) from France, Macewen (5) from Scotland, Curtis (6) from the United States, and Barth (7), Axhausen (8), and Lexer (9) from Germany popularized these grafting techniques. During this period, bone transplantation was still considered experimental, with an unpredictable outcome. Clinical need spurred its development as a surgical procedure. Between 1947 and 1950, Bush (10) and Wilson (11) developed preservation techniques that enabled storage of allograft bone at –20°C. This breakthrough made it possible to use allografts clinically on a larger scale, particularly to replace traumatic or tumor-related bone loss. In the 50s, Herndon and Chase (12), Burwell and Gowland (13), Campbell (14), and Urist (15) performed extensive laboratory and animal experiments to provide scientific support for the clinical application of bone grafting. Most of these investigations were aimed at the processes of union, incorporation, osteoinduction, and immunogenetic reactions of the grafts. In the 70s, clinicians began to use bone grafting to repair osseous defects in association with primary and revision hip arthroplasty. The size of the bone grafts used differed among the various surgeons. Some surgeons adhere to the use of small-fragment grafts (16,17), while others advocate the use of large-fragment or structural grafts with or without cement for revision hip arthroplasty (18,19,20).
We started to use impacted morselized grafts (small fragments of 1 × 1 × 1 cm) with cement for acetabular revisions in 1979 based on reports on the clinical use of small fragments in primary total hips. In 1975, Hastings and Parker (21) described the combination of cemented total hip replacement and autogenous morselized cancellous grafting in intrapelvic protrusio. However, the graft was not impacted and was subsequently totally covered with a narrow-rimmed, coarse-mesh cup. In 1978, McCollum et al. (22) reported their first experience with autogenous wafers of corticocancellous bone to augment acetabular bone stock in 25 patients with protrusio acetabuli after failed total hip arthroplasty. They utilized gel foam to avoid direct contact between cement and graft material, thereby preventing cement penetration into the graft. They subsequently tucked a fine vitallium mesh into acetabular anchoring holes to distribute the forces across the acetabulum. They combined a cemented cup with an Eichler ring if the medial wall was absent. In 1983, Roffman et al. (23) investigated the fate of autogenous chips under a layer of polymethylmethacrylate bone cement in an animal model with intrapelvic protrusio. Their model had a medial segmental defect. Histologic evaluation revealed bone formation from the acetabular wall toward the graft. The graft appeared viable, and new bone formation was induced along the surface adjoining the bone cement. Based on these experimental results in dogs, Mendes et al. (24) published the results of a clinical study on
primary cemented arthroplasties combined with autogenous bone chips supported with a metal mesh for intrapelvic protrusio. Their follow-up studies of up to 6 years showed clinical success in all patients. In 1984, Slooff et al. (25) published their initial experience with a new technique using morselized allografts which was started in 1979. Acetabular segmental defects were closed with corticocancellous slices or with flexible metal wire meshes. The contained acetabulum was tightly packed with allograft chips (sized ∼1.0 cm3). The cup was inserted after pressurizing the cement directly onto the graft.
The anatomy of the hip was reconstructed by packing as much chip graft material as necessary to build up the socket to the level of the transverse ligament.
primary cemented arthroplasties combined with autogenous bone chips supported with a metal mesh for intrapelvic protrusio. Their follow-up studies of up to 6 years showed clinical success in all patients. In 1984, Slooff et al. (25) published their initial experience with a new technique using morselized allografts which was started in 1979. Acetabular segmental defects were closed with corticocancellous slices or with flexible metal wire meshes. The contained acetabulum was tightly packed with allograft chips (sized ∼1.0 cm3). The cup was inserted after pressurizing the cement directly onto the graft.
The anatomy of the hip was reconstructed by packing as much chip graft material as necessary to build up the socket to the level of the transverse ligament.
Biology of Acetabular Bone Impaction Grafting
Animal Studies
By the mid-1980s, animal experiments were being performed on both the acetabular side and the femoral side at the Radboud University Medical Center of Nijmegen (26,27,28,29) to provide additional scientific support for this reconstruction method. A study in goats was designed to histologically evaluate the processes involved in graft incorporation. The surgical technique was comparable to that used in the human procedures. This experiment demonstrated rapid union of the graft with host bone using this method. From 24 weeks onward, very little of the original graft bone remained, and a new immature trabecular bony structure was formed (Fig. 112.2). In the course of time, no signs of resorption or collapse of the reconstruction were seen. The results of this study encouraged more extensive clinical use of this biologic reconstruction method.
Human Biopsies
Only human data can prove true clinical incorporation. Van der Donk (30) reported the results of our histologic analysis of graft incorporation after primary and revision operations of acetabular reconstruction with impacted morselized grafts and cement. In the period of 1983 to 1998, 21 reoperations were performed for different reasons in 20 patients, all previously done with acetabular bone impaction grafting. Core biopsies and large bone fragments were harvested from 3 months to 15 years after the reconstruction during re-revision of the acetabular components or during other invasive procedures not directly related to loosening of the acetabular components. Analysis of the human core biopsies suggested that a sequence of events took place strongly resembling the incorporation process observed in animal experiments. During revascularization of the graft, osteoclasts removed large parts of the chip graft. Woven bone was formed on the graft and fibrin remnants and in fibrous stroma tissue that was invading the graft during neovascularization. Thereafter the mixture of graft, new bone, and fibrin was rapidly remodeled into a new bony trabecular structure. In some specimens, localized areas were found where nonincorporated bone graft was surrounded by vital fibrous tissue and (fibro)cartilage. Occasionally incorporated bone grafts showed signs of secondary necrosis. From these observations, we conclude that reconstruction of bony defects with impacted allograft chips indeed results in the generation of a new bony structure.
Indications
The main indication for bone impaction grafting on the acetabular side in hip revision arthroplasty is the progressive loss of periprosthetic bone, which results in pain and physical disability. Radiographic signs of loosening often precede the clinical symptoms of failure. Since the 70s, regular follow-up had been included in the standard postoperative protocol after primary total hip arthroplasty to prevent extensive loss of bone (Fig. 112.3).
When planning revision arthroplasty, attention should be paid to assessing the diagnosis of loosening and establishing
the cause of failure. After a thorough physical examination laboratory tests and good-quality plain radiographs in three views should be obtained: anteroposterior, axial, and abduction-external. These radiographs could be used to evaluate the severity of anatomic distortion, the location and extent of bone lyses, the distribution of cement, and any acetabular or femoral deficiencies. Serial radiographs could be compared to monitor changes in the position of the component, cement, and bone stock over the course of time. CT scanning can be helpful to evaluate the periprosthestic bone loss in demanding cases. If septic loosening is suspected, prerevision management should include gamma-immunoglobulin scintigraphy (IGG) or a leucocyte scan to exclude infection. Also, a preoperative joint puncture can be planned to obtain material for bacterial cultures. For patients with an infected loose hip implant, we used a two-stage revision.
the cause of failure. After a thorough physical examination laboratory tests and good-quality plain radiographs in three views should be obtained: anteroposterior, axial, and abduction-external. These radiographs could be used to evaluate the severity of anatomic distortion, the location and extent of bone lyses, the distribution of cement, and any acetabular or femoral deficiencies. Serial radiographs could be compared to monitor changes in the position of the component, cement, and bone stock over the course of time. CT scanning can be helpful to evaluate the periprosthestic bone loss in demanding cases. If septic loosening is suspected, prerevision management should include gamma-immunoglobulin scintigraphy (IGG) or a leucocyte scan to exclude infection. Also, a preoperative joint puncture can be planned to obtain material for bacterial cultures. For patients with an infected loose hip implant, we used a two-stage revision.
Contraindications
Inability to contain a large segmental defect, either peripheral or central (31)
Unhealed acetabular fracture
The presence of untreated infection
Previous radiotherapy to the area of the affected hip
Surgical Technique
The treatment strategy (Fig. 112.4) for correcting structural acetabular integrity loss and impairment of implant mechanical support with acetabular bone impaction grafting is based on the following principles:
Repair hip mechanics by positioning the cup at the level of the anatomic acetabulum (teardrop).
Close segmental defects with metal wire mesh to achieve containment; a cavitary defect remains.
Use allograft bone chips to augment the cavitary defect.
Restore stability by impacting the chips and using bone cement.
The X-Change Acetabular Revision Instrumentation System (Stryker Howmedica, Newbury, England) was developed to achieve these goals. The posterolateral approach is our standard approach. This enables extensive exposure of all aspects of the acetabulum and proximal femur. A trochanteric osteotomy is rarely necessary. Identifying major landmarks is helpful for orientation purposes if the anatomy is disturbed by scarring and distortion. These landmarks include the tip of the greater trochanter, the tendinous part of the gluteus maximus, the lower border of the gluteus medius and minimus, and the sciatic nerve. Aspiration of the hip is done at this stage to obtain fluid culture. The proximal part of the femur is exposed and mobilized extensively before the hip is dislocated. Free exposure of the entire socket can be achieved by removing all scar tissues, performing circumferential capsulotomy,
and if needed, dividing the iliopsoas tendon or releasing the femoral attachment of the gluteus maximus tendon.
and if needed, dividing the iliopsoas tendon or releasing the femoral attachment of the gluteus maximus tendon.
After removal of the acetabular component and, if appropriate, the cement, the fibrous interface is removed completely from the irregular acetabular wall using sharp spoons and curettes. Special care is taken to locate the transverse ligament on the inferior side of the acetabulum. The socket is reconstructed up to this level (Fig. 112.5A). At least three specimens are taken from the interfacial fibrous membrane for bacterial culture, if available one additional specimen can be sent for frozen section. After these samples are taken, systemic antibiotic therapy is started.
The acetabular floor and wall are examined meticulously for any hidden medial or peripheral segmental defects. Peripheral rim meshes are placed on the outer side of the pelvic bone (Figs. 112.4A,B, 112.5B). The overlying muscles (abductors) can be elevated off the pelvic bone with only limited risk of neurovascular damage. With a pair of scissors,
a flexible stainless steel mesh is trimmed and adapted to fit the defects. The mesh is then screwed to the iliac bone (Fig. 112.5C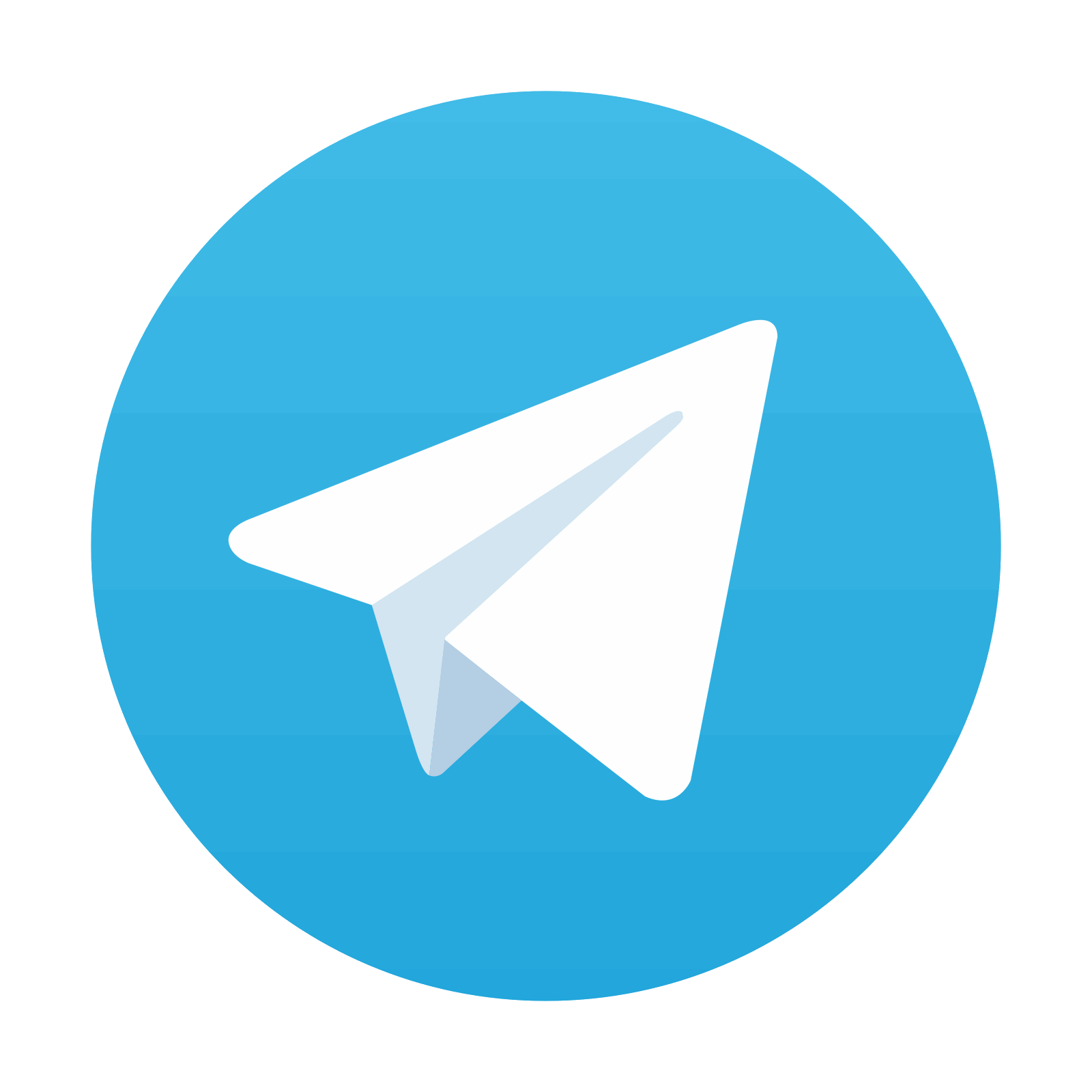
a flexible stainless steel mesh is trimmed and adapted to fit the defects. The mesh is then screwed to the iliac bone (Fig. 112.5C
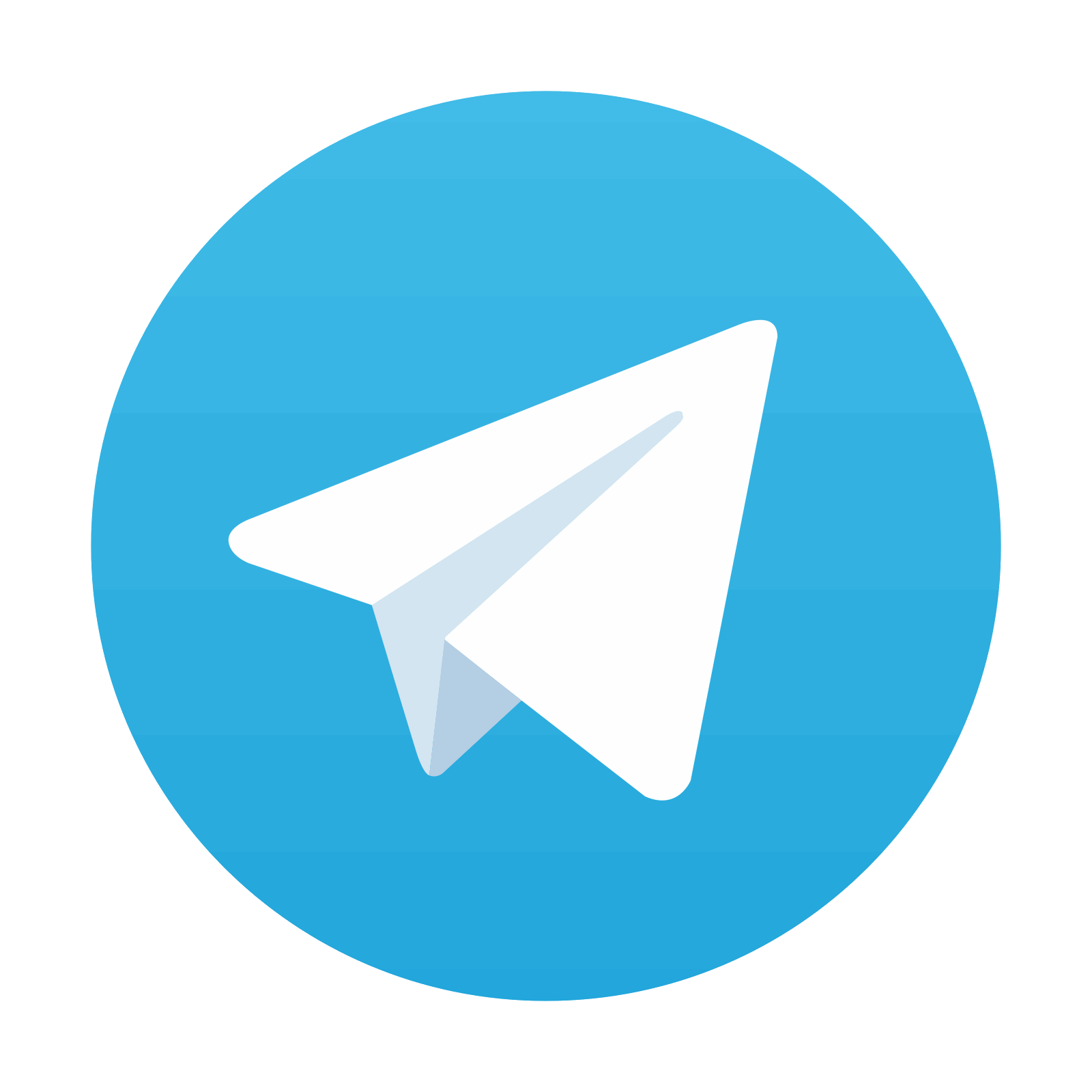
Stay updated, free articles. Join our Telegram channel

Full access? Get Clinical Tree
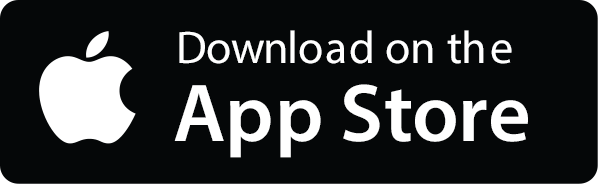
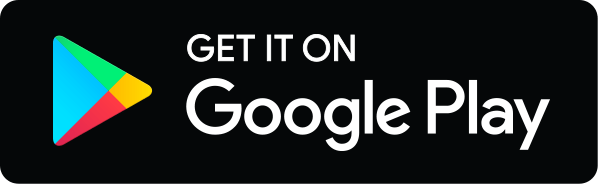