Children
Adults
1. Fat pads
1. Fat pads
2. Anterior humeral line
2. Cortex of radial head and neck
3. Radiocapitellar line
3. Radiocapitellar line
4. Ossification centers
4.1.2 Magnetic Resonance Imaging and Magnetic Resonance Arthrography
Magnetic resonance imaging (MRI) is considered the next step in the imaging workup. Appropriate patient positioning, coil selection, and sequence technique are of vital importance in proper imaging of the elbow. The anatomical position with the patient lying supine, the elbow in full extension and the forearm in supination, is the most comfortable and a widely used position. Note that with this position, the elbow is located off-center of the scanner’s magnetic field. This will reduce the signal-to-noise ratio and may introduce inhomogeneous fat suppression. For this reason, fat suppression by means of inversion recovery sequences is preferred over frequency-selective fat suppression techniques when the anatomical position is applied [13]. An alternative is the “superman position,” where the patient lies prone with the elbow over the head and the forearm in pronation. This will bring the elbow closer to the center of the magnet which will increase overall image quality at the cost of markedly reduced patient comfort. In any case, a dedicated surface coil should be used for optimal imaging of the elbow [14]. Obtaining cross-sectional images in all three orthogonal planes will allow for adequate assessment of all relevant structures around the elbow.
T1-weighted (T1W) images are useful for illustrating anatomical detail, whereas fat-saturated T2-weighted (T2W) images or short-tau inversion recovery (STIR) images are suitable for detecting pathological changes manifesting as fluid or edema. Furthermore, proton density-weighted (PDW) images can provide additional anatomical detail. Gradient-echo sequences are not routinely indicated but may enhance the visibility of intra-articular loose bodies [14, 15]. However, detecting loose bodies without intra-articular contrast remains difficult. Gadolinium is a contrast agent used in MR imaging that can be injected intravenously or directly into a joint, known as MR arthrography (MRA) (see below). Indirect MRA by means of intravenous administration of gadolinium may aid in the detection of post-traumatic disorders affecting the synovium. Direct MRA by means of intra–articular injection of gadolinium may provide superior visualization of disorders commonly encountered in throwing athletes, including partial capsular and ligamentous (ulnar collateral ligament) tears, intra- articular loose bodies, instability, and osteochondritis dissecans [16, 17].
For MRA, approximately 5–10 mL of gadolinium diluted in sterile saline (1:250) is injected with a 20- or 23-gauge needle into the elbow joint. The elbow joint space can be accessed via the standard lateral or posteromedial approach under fluoroscopy. For the lateral approach, the needle is inserted vertically at the superior third of the radiocapitellar joint line while the patient is lying prone with the elbow in 90° flexion and the forearm in supination. A disadvantage of this lateral approach is the possible extravasation of contrast agent around the radial collateral ligaments. For this reason, the alternative posteromedial approach can be employed with the patient lying supine on the fluoroscopic table, with the elbow over the head in 30° flexion and the forearm in pronation. The needle is then inserted between the olecranon and the medial epicondyle, approximately 1 cm lateral to the medial epicondyle to avoid damaging the ulnar nerve. Subsequently, the needle is advanced in anterolateral fashion into the olecranon fossa. Fat- saturated T1W and T2W sequences should be obtained immediately after contrast injection [18] (Table 4.2).
Table 4.2
Characteristics of the elbow on MRI
Appearance on: | |||
---|---|---|---|
Tissue | T1-weighted images | T2-weighted images | T2-fat saturated images |
Cortical bone | Hypointense | Hypointense | Hypointense |
Medullary bone | Hyperintense | Hyperintense | Hypointense |
Fibrous cartilage | Hypointense | Hypointense | Hypointense |
Hyaline cartilage | Isointense | Isointense | Isointense |
Bands and ligaments | Hypointense | Hypointense | Hypointense |
Fluid | Hypointense | Hyperintense | Hyperintense |
Fat | Hyperintense | Hyperintense | Hypointense |
Muscle | Hypointense | Hyperintense | Hyperintense |
4.1.3 Computed Tomography and Computed Tomographic Arthrography
CT scans of the elbow are mainly used in the acute setting for assessing osseous abnormalities such as occult fractures and loose bodies, for further characterisation, and for support in preoperative planning [19, 20]. Current multi-detector CT scans allow for high-resolution images, multi-planar reconstruction, and fast scanning times. Typically, a section thickness of 1 mm is used with a matrix size of 512 × 512, and scanning is performed in the axial plane [21, 22]. The patient is scanned in the prone position with the elbow resting above the head at about 90° flexion [23–25].
In order to perform CTA, iodinated contrast agent is injected into the elbow joint. As in magnetic resonance arthrography (MRA), 5–10 mL of contrast agent is injected under fluoroscopic guidance through the lateral and, in some cases, the posteromedial approach. In addition to iodinated contrast agent, air can be injected into the elbow. This is defined as double-contrast arthrography. CT scans should be obtained within 30 min of contrast administration [26].
CTA is particularly useful in the evaluation of osteochondritis dissecans, osteochondral lesions, and loose bodies [27]. However, in the diagnostic workup of the athlete’s injured elbow, MRA has essentially replaced the role of CTA. The main reasons for this are the absence of ionizing radiation in MRA and the fact that MRA is superior in the detection of concomitant soft tissue injury [18]. Nonetheless, CTA can be used as an alternative in patients with contraindications for MRA such as pacemakers, implanted devices, or gadolinium-based contrast allergies [28].
4.1.4 Ultrasound
The major advantage of ultrasound (US) is that it provides a low-cost, noninvasive, and dynamic evaluation of elbow structures, without ionizing radiation [29–31]. However, this imaging modality is highly operator-dependent and thus requires sufficient experience of the assessor. US can assist clinicians in the assessment of a wide variety of elbow injuries, including overuse syndromes, traumatic changes, inflammatory diseases, and neuropathies [31]. Transverse and longitudinal images of all four aspects (posterior, anterior, medial, and lateral) of the elbow in both flexion and extension are necessary for a complete examination [31].
Echogenicity is the characteristic ability of an elbow structure to return a signal in US examination; each tissue has its own characteristic appearance. A practical order of echogenicity in musculoskeletal ultrasound can be depicted as bone, ligament, tendon, nerve, and muscle [29]. In general, bone and gas-like substances are hyperechoic and fully reflect the sound waves, which is represented by a more intense appearance on US images. Muscles and fluids are less echogenic (hypoechoic) and are represented darker.
Ultrasound plays a major role in the examination of traumatic changes to ligaments and tendons of the elbow [29, 32]. Although these structures have a similar appearance, they can be distinguished because ligaments are slightly more echogenic than tendons. Moreover, the echogenicity of the fibrillar tendinous pattern increases when the tendon is being held under tension. Pathologic degeneration and partial tearing of a tendon are visualized as a structural hypoechoic gap. In case of a complete tear, the fibrillar pattern is completely absent. In addition, US may demonstrate intra-articular effusion due to a fracture even when the undisplaced fracture line is not detected on plain radiographs. Fractures can also be detected directly by US through depiction of irregularities or interruption of the hyperechoic bone cortex [31].
4.2 Osseous and Osteochondral Injury of the Elbow
4.2.1 Fractures of the Elbow
Elbow fractures in overhead athletes are most often caused by low energy trauma, such a fall onto an outstretched hand (FOOSH) and hyperextension or hyperflexion injuries [33]. Nontraumatic upper extremity fractures related to throwing are rare [34, 35]. However, stress fractures arising from repetitive microtrauma are not uncommon. In the following section, a description of fractures of the distal humerus, proximal ulna, and proximal radius, with associated characteristics on imaging, will be given.
4.2.1.1 Outline Pediatric Osseous Injury
In General
The immature skeleton contains growth plates, which appear as a radiolucency similar to cartilage on radiographs. Understanding of the developmental anatomy of the pediatric elbow is essential to distinguish normal ossification centers from a fracture fragment in radiography, since misinterpretation is not uncommon [36]. The mnemonic CRITOE is a helpful tool in analyzing pediatric elbow injury. It represents the sequential order of appearance of the ossification centers of the elbow: capitellum, radial head, internal (medial) epicondyle, trochlea, olecranon, and external (lateral) epicondyle [1, 5]. This sequential order extends over the period from 1 year to 12 years of age [37].
Pediatric osseous injury differs in many aspects from adult osseous injury due to the differences in bone composition between children and adults [8, 38]. The thick periosteum of the immature skeleton, for example, inhibits displacement of a fracture. However, supracondylar fractures with posterior displacement occur frequently and are thus an exception to this rule. Finally, children’s bones tend to be more flexible which can result in plastic bowing, torus, or greenstick fractures, mostly affecting the radius or ulna in FOOSH or hyperextension injury [5].
Physeal Injury
Since the cartilaginous physis is a more vulnerable structure than the surrounding ligaments and muscle tendons, injuries affecting the physis are common in childhood [2]. Fractures of the epiphysis and/or metaphysis are classified according to the Salter-Harris classification, which relates the radiographic appearance to the clinical importance of the fracture (see Table 4.3) [39]. Nevertheless, MRI is considered superior for evaluating fractures of the cartilaginous epiphysis in children [40].
Type | Mnemonic | Description of fracture |
---|---|---|
I | “Slipped” | Through the physis without involvement of bone, epiphysis, or metaphysis |
II | “Above” | Involving part of the metaphysis and extending to the physis |
III | “Lower” | Involving the epiphysis and extending to the physis |
IV | “Through” | Involving epiphysis and metaphysis and extending to the physis |
V | “Rammed” | Involving compression of the physis |
4.2.1.2 Fractures of the Distal Humerus
Fractures of the distal humerus can broadly be categorized into supracondylar, transcondylar, or intercondylar fractures (above the olecranon fossa, through the olecranon fossa, or between the condyles, respectively) [41, 42]. More specific and commonly used is the AO classification system, in which type A describes an extra-articular fracture, type B an intra-articular fracture of a single column, and type C an intra-articular fracture of both columns with no portion of the joint contiguous with the shaft (see Table 4.4) [41]. Each type is subdivided into three subtypes to classify the degree of comminution, with subtype 3 being the highest degree of comminution. Anteroposterior, lateral, and oblique views in plain radiography can be used to confirm the presence and location of distal humeral fractures [42].
Table 4.4
AO/OTA classification of distal humerus fractures
![]() | ||
Type A | Extra-articular | Supracondylar |
Type B | Intra-articular, single column | Partial articular-isolated condylar, coronal shear, epicondyle |
Type C | Intra-articular, both columns | Complete articular |
Supracondylar (type A) fractures are common and account for more than half of all elbow fractures in children, but are relatively uncommon in adults [6]. Pediatric supracondylar fractures are classified according to the classification of Gartland [43]. Type I fractures are non-displaced, type II fractures are partially displaced (with intact posterior cortex) and type III fractures are completely displaced. The anterior humeral line in particular can be used to assess the direction of the displacement, which is commonly posterior [5]. A rare, but important complication of pediatric supracondylar fractures is the fishtail deformity (see Sect. 4.2.2) [44, 45].
Transcondylar (type B) fractures include fractures of the lateral and medial humeral condyle. Fractures of the lateral condyle are the most common fractures in children under the age of 7 years [5]. When only the cartilaginous part of the distal humeral epiphysis is involved, this fracture equals a Salter-Harris type IV epiphyseal fracture. A specific type of transcondylar fractures of the capitellum and trochlea are coronal shear fractures. These fractures occur when the radial head impacts into the anterior articular cortex of the distal humerus and both the capitellum and the lateral ridge of the trochlea are sheared off. Indicative for this injury is the double–arc sign on lateral view radiographs [46, 47]. This sign represents an increased radiographic density due to overprojection of the subchondral bone of the displaced capitellum and the lateral trochlear ridge. Coronal shear fractures can also be visualized with a radial head-capitellum view [48].
Regarding other imaging modalities, two- and three-dimensional CT images have been shown to be of particular benefit in preoperative decision making and planning of the operative treatment [49]. Nonoperative treatment (i.e., immobilization and bracing) is only recommended in case of non-displaced fractures. Patients with displaced, comminuted, or highly unstable distal humeral fractures should be referred to an orthopedic surgeon, since surgical intervention is the standard treatment [41, 42].
4.2.1.3 Fractures of the Proximal Ulna
Olecranon process fractures can be the result of a direct trauma to the elbow, for example a fall on the elbow with the arm flexed. As a consequence, the olecranon collides with the distal humerus and is often comminuted [50]. These fractures occur more frequently in adults than in children, as the immature olecranon is relatively stronger than the distal humerus (which also explains the higher occurrence of supracondylar fractures in children). Indirect forces are mostly due to a FOOSH injury together with forceful contraction of the triceps which may show transverse or short oblique fractures on plain radiographs [50, 51]. Undisplaced, simple fractures are easily assessed on plain radiographs. Displaced or comminuted fractures require two- and three-dimensional CT imaging in support of surgery [52].
In addition to traumatic injury, the olecranon process is the most common location for stress fractures in throwers [2]. During throwing, repetitive forces in valgus load are applied through excessive pulling of the triceps on the olecranon, which may result in posteromedial osseous stress syndrome. This comprises trabecular collapse and transverse or short oblique stress fractures. Since plain radiographs may not show significant alterations in the appearance of the proximal ulna, accurate assessment is justified [53, 54]; progression of small stress fractures to a complete and displaced fracture is possible. Either a hairline fracture or a lucent region surrounded by a sclerotic margin (indicating nonunion and periosteal new bone formation) can be seen. These features can also be detected with CT [2]. However, MR imaging is the most sensitive method for identifying early changes consistent with osseous stress injury, like bone marrow edema and hyperemia [53]. These changes on T1-weighted images consist of poorly defined, patchy areas of low signal intensity in the affected bone.
Fractures of the coronoid process rarely occur isolated. Since the coronoid is responsible for resisting posterior displacement of the ulna, these fractures are often associated with other elbow injuries that increase joint instability. In the O’Driscoll classification, three major traumatic injury patterns are linked to coronoid fractures [55]. This classification can aid in predicting associated injuries of coronoid fractures [56]. Type I includes a small transverse fracture of the coronoid tip. This fracture accounts for one of the three distinct injuries in the terrible triad, the others being a fracture of the radial head and a posterior elbow dislocation [57]. If external rotation forces and valgus stress are loaded axially in a FOOSH injury, the lateral collateral ligament (LCL) is typically torn as well. Type II fractures of the anteromedial facet are often seen with varus posteromedial rotatory instability pattern injuries, occurring after an elbow subluxation. Associated injury includes an LCL avulsion from the lateral epicondyle. Varus stress radiographs often reveal radiocapitellar widening and ulnohumeral narrowing. Type III includes relatively large fractures of the coronoid process, associated with transolecranon fracture-dislocations (anterior or posterior).
4.2.1.4 Fractures of the Proximal Radius
Radial head fractures are the most common type of elbow fracture in athletes and represent 50 % of all elbow fractures in adults [33]. In children, the radial neck is more commonly involved (leading to Salter-Harris II fracture). Based on results of 100 cases of radial head fractures, Mason established a classification system to guide treatment based on the injury pattern [58]. Type I fractures include non-displaced or peripheral fractures of the rim, type II includes displaced fractures of the rim, and type III fractures are comminuted and displaced fractures of the entire radial head. Johnston added a fourth type to this classification, which denotes a fracture of the radial head with associated dislocation [59]. Initially, type I fractures are treated nonoperatively, type II may be treated either nonoperatively or operatively, while types III and IV require surgical management. However, although these guidelines of the Mason-Johnston classification are widely used, there is a paucity of data confirming the outcomes of surgical management [60].
Isolated radial head fractures resulting from a fall with the elbow extended and the forearm pronated occur rarely. Investigation of radial head fractures with MR imaging showed that radial head fractures in three-quarters of cases are associated with soft tissue injuries [61, 62]. Common injuries occurring in association with these fractures are posterior dislocation of the elbow, medial collateral ligament rupture, capitellar fracture, terrible triad injuries, and Monteggia injuries [63]. If a radial head fracture is suspected, anteroposterior and lateral radiographs of the elbow should be obtained. A radiocapitellar view may help delineate the fracture. In addition, computed tomography can identify fractures not visualized in plain radiographs. CT may help in identifying the fracture pattern, the degree of comminution (if present), possible associated injuries and in planning surgical treatment [63, 64].
4.2.2 OCD and Avascular Necrosis Around the Elbow
4.2.2.1 Osteochondritis Dissecans of the Capitellum
Osteochondritis dissecans (OCD) is an idiopathic disorder of the subchondral bone with dissection of the articular surface and underlying bone of the immature skeleton. OCD is commonly localized in the capitellum of the dominant elbow but can also occur in the trochlea, radial head, and olecranon [65]. It typically affects young, competitive athletes in overhead sports such as baseball or weight-bearing sports like gymnastics, in which repetitive valgus stress is placed on the elbow joint [66, 67]. Patients, most commonly adolescent boys, present with lateral elbow pain, swelling, tenderness, stiffness, and locking of the joint. Although the etiology remains unclear, it is believed that the underlying pathogenesis involves repetitive microtrauma due to compression and shear forces, leading to overuse injury of the vulnerable and relatively hypovascular epiphyseal cartilage [65].
Although prevention is the best treatment for OCD, early detection and classification of the lesion are necessary to protect athletes from developing irreversible damage [68, 69]. The International Cartilage Repair Society (ICRS) divides OCD lesions into four categories. To determine the best treatment option for capitellar OCD in young athletes, it is important to differentiate between stable and unstable lesions: ICRS I and II are classified as stable and ICRS III and IV as unstable [69–72]. However, the major drawback of this classification is that it is based on intraoperative findings. To assess the stability of the OCD lesion in a noninvasive way, the use of ultrasound, radiographs, MRI, or CT is recommended [72]. The characteristics of each imaging modality will be discussed in the following section (Table 4.5).
Type | Description |
---|---|
I | Stable lesions with a continuous but softened area covered by intact cartilage |
II | Lesions with partial discontinuity that are stable when probed |
III | Lesions with a complete discontinuity that are not yet dislocated but are unstable when probed (dead in situ) |
IV | Empty defects as well as defects with a dislocated fragment or a loose fragment within the bed |
Ultrasound is useful in the initial examination of cartilaginous changes in capitellar OCD [74]. US can visualize the subchondral bone and overlying articular cartilage simultaneously in one dynamic image [75]. The image should be obtained in both an anterior and a posterior longitudinal view to display the whole capitellum. The normal capitellum is shown as a highly echogenic band with the overlying cartilage as an overlying hypoechoic band. Subchondral bone flattening causes the highly echogenic band to narrow. Moreover, non-displaced or (slightly) displaced bony fragments, marrow gap formation, or complete osteochondral defects can be seen on ultrasound imaging [74]. It is advised to compare findings on ultrasound with MRI and/or radiographic assessment to identify both cartilaginous and bone changes, so the lesion can be more accurately classified [74, 76].
Routine AP radiographic examination of the elbow for detecting capitellar OCD and intra- articular loose bodies has limited sensitivity [77]. However, radiographic images of the capitellum on radiocapitellar view or AP view with the elbow 45° flexed can show the following:
Grade I. Localized flattening or subchondral radiolucency
Grade II. Non-displaced bone fragment(s)
Grade III. Displaced or detached fragment(s)
Takahara et al. [71] proposed a guideline for treatment, based on findings at initial presentation of the patient, supplemented with radiographic findings (Table 4.6) [71]. In stable OCD, an immature capitellum with open growth plate is present with flattening or radiolucency of the subchondral bone (Grade I), but with normal elbow motion. The preferred treatment is conservative; elbow rest and analgesics are recommended. In unstable OCD, the capitellum is mature (the growth plates have closed) and fragments (Grade II or III) may occur. The fragments or loose bodies can lead to restricted elbow motion due to narrowing of the articular space. In this case, surgical treatment is indispensable to prevent further damage. The advantage of this classification system is that it directly links radiographic findings with the ICRS classification and thus is useful in the choice for treatment [78].
Classification of lesion | Capitellar growth plate | Radiographic grade | Range of motion | ICRS classification | Preferred treatment |
---|---|---|---|---|---|
Stable | Open | I | Normal | I | Elbow rest |
Unstable | Closed | II or III | Restricted | II | Fixation and bone-peg graft |
III | Fixation and bone-peg or iliac bone graft | ||||
IV | Fragment removal and reconstruction for large defect |
Magnetic resonance imaging has been approved as the most sensitive and reliable means for the assessment of osteochondritis dissecans [74, 79]. MRI provides information about size, location, presence of joint effusion, bone marrow change, and loss of continuity or cartilage over the OCD lesion [79]. Cartilage changes in early disease may not be obvious radiographically, but can be visualized with MRI [68]. These early changes of osteochondral defects are detectable on T1-weighted images and appear normal on T2 images [67]. Advanced changes are detectable in both T1 and T2 images. T2-weighted images may show high-signal intensity interfaces between fragments and their beds or reflect the interposition of synovial fluid interposed through the articular cartilage. Focal articular defects may be seen as well [69, 72]. The MRI staging system developed by Itsubo et al. [79] provides evidence regarding the instability of the OCD and the corresponding stages of the ICRS classification, but has not yet been validated in other studies [79].
It should be noted that the literature on imaging of capitellar OCD by computed tomography (CT) is limited. The general consensus on the advantages of CT over radiography or MRI is that CT can aid in defining the subchondral bone condition and that it is often used to determine the extent of the osseous lesion and the presence of ossified loose bodies [80]. However, CT should not be used to detect cartilaginous change at the lesion; for this purpose, computed tomographic arthrography (CTA) is more suitable. CTA favors examination of the overlying cartilage and can confirm the intra-articular position of calcified loose bodies, yet this can also be achieved with MRI [73, 81].
4.2.2.2 Panner’s Disease
It is important to distinguish Panner’s disease from OCD of the capitellum. Although the presentation and clinical features may be similar, Panner’s disease is a self-limiting condition of the epiphysis and will resolve with rest and conservative treatment [65]. In general, it affects a younger age group (mainly boys under the age of 10 years) and it is not necessarily related to sports. The characteristic appearance of Panner’s disease on radiographs is the initially subchondral rarefaction, which is in a later stage followed by translucency and fragmentation of the entire capitellum. Magnetic resonance imaging shows low T1 signal and high T2 signal of the entire capitellum. Loose bodies are seldom seen [65].
4.2.2.3 Hegemann’s Disease
In the continuum of disorders of endochondral ossification like OCD and Panner’s disease, in 1951 Hegemann described a total of 15 cases of avascular osteonecrosis of the humeral trochlea [82]. Since then, reports on this disease have been limited. This condition seems to affect predominantly preadolescent boys and is seldom accompanied by pain. Swelling and decreased range of motion are more often described [83]. In contrast to OCD, there is no locking of the joint and radiography shows rarefaction of the entire epiphyseal center of the trochlea (instead of the subchondral bone only) [84]. Another condition that strongly resembles Hegemann’s disease is the fishtail deformity of the trochlea, a late complication of pediatric supracondylar fractures [45]. Claessen et al. [85] provided an overview of the most recent knowledge on the etiology, radiographic findings, and treatment options of both these rare conditions [85].
4.2.3 Apophysitis and Apophysiolysis: Little Leaguer’s Elbow
The apophysis is a secondary ossification center located outside the joint surface. Injury of the medial epicondylar apophysis occurs almost exclusively in young athletes performing overhead sports and is referred to as the clinical diagnosis Little Leaguer’s elbow [86–88]. The medial epicondyle is relatively weak compared to the increasing muscle strength in adolescents. Therefore, apophysiolysis or apophyseal avulsion fractures are often the consequence of sustained valgus stress forces with traction of the common origins of the flexor muscles at the apophysis, due to repetitive overhead throwing [5, 89, 90]. Moreover, avulsion fractures can also be the consequence of an acute traumatic event such as a dislocation due to FOOSH injury [91].
AP and lateral radiographic images with comparative views of the unaffected side should be used in the initial evaluation [1]. Although these images appear normal in 85 % of cases, they may reveal a hypertrophic medial epicondyle with bony fragmentations and apophyseal widening or complete avulsion from the underlying humerus, with possible entrapment of the fragment in the joint [87, 89].
MRI is not warranted in the initial imaging workup, but can be justified to outline the surrounding structures [92]. MR images in such cases may show bone marrow edema in the apophysis (or distal in the humerus) and tendinopathy of the common flexor tendon. Contrary to previous literature, there is a growing consensus that the ulnar collateral ligament (UCL) is not involved in the pathology of the Little Leaguer’s elbow, but solely associated with valgus extension overload in adult patients (see Sect. 4.3.1) [92].
4.2.4 Degeneration, Osteophytosis, and Loose Bodies
While traumatic injury may precipitate secondary degenerative arthritis in the elbow, primary degeneration is not associated with acute elbow trauma or rheumatologic disease. Primary degenerative arthritis of the elbow is a relatively rare condition, but occurs to a greater extent in overhead athletes at whom excessive stress on the elbow joint is placed [93, 94]. The pathologic changes that occur in both the radiohumeral and ulnohumeral compartments of the elbow can be divided in three stages [95]. The first stage involves loss and fragmentation of the cartilage due to repetitive impaction of the coronoid process and the tip of the olecranon against the olecranon fossa membrane. As a response to this erosion, hypertrophic bone and cartilage formation results in so-called osteophytes and loose bodies. Osteophytes or bone spurs reduce the amount of joint space needed for a full, pain-free range of motion, giving rise to symptoms as pain, locking, or reduced elbow motion. In the final stage, the impingement caused by these small protuberances (particularly in the olecranon fossa) leads to distortion and in most severe cases to contracture of the elbow joint. Arthroscopic intervention with removal of the eroded bone and its fragments is the best treatment option to prevent further degeneration of the elbow [96]. Plain radiography and computed tomography are the modalities of choice when assessing the condition of the elbow.
Two views in plain radiography are usually sufficient for the initial evaluation of primary osteoarthritis. Standard lateral radiographs allow identification of the most frequent features of the osteoarthritic elbow (i.e., osteophytes of all involved bony structures, thickening of the olecranon fossa membrane, and joint space narrowing). The anteroposterior view in addition enables the assessment of the olecranon fossa membrane [97].
In preoperative planning, computed tomography (CT) is favorable when heterotopic ossification or intra-articular loose bodies are suspected [93]. More advanced three-dimensional CT scans can specifically determine the size, location, and bony architecture of the hypertrophic bone spurs and loose bodies [97, 98].
4.2.5 Goalkeeper’s Elbow
Shot blocking of a ball with the forearm fully extended induces repeated hyperextension trauma of the elbow, mostly seen in goalkeepers of handball and soccer [99]. The injury pattern resembles elbow lesions in overhead athletes: repeated impaction of the posteromedial olecranon leads to arthritic changes with cartilage damage, osteophyte formation, and intra-articular loose bodies [100]. The presence of these pathological alterations can be confirmed by radiological evaluation. Soft tissue lesions can be visualized by US or MRI and may comprise bilateral thickening of the medial collateral ligament, flexor-pronator tendon, triceps tendon, and ulnar nerve [100].
4.3 Ligamentous Injury of the Elbow
Various osseous and soft tissue constraints provide static and dynamic stability to the elbow joint, respectively. Primary stabilization is provided by the ulnohumeral articulation as well as by the medial (ulnar) and lateral (radial) collateral ligament complexes. The medial ulnar collateral ligament (MCL/UCL) complex comprises anterior, posterior, and transverse bundles, of which the anterior bundle is the primary restraint against valgus stress. The lateral ligament complex includes the radial collateral ligament, the lateral collateral ligament (LCL), and the annular ligament, of which the LCL provides both varus- and posterolateral stability [101]. The radiocapitellar articulation, the common extensor tendon, the flexor-pronator tendon, and the joint capsule all contribute to secondary stabilization [102].
Ligamentous injury of the elbow in athletes can be caused by repetitive overhead activities or by an acute traumatic event like an elbow dislocation. Timely recognition of injuries to these structures is very important; disruption of the ligaments may threaten elbow stability and can possibly be career ending for an athlete [102, 103]. MR imaging is indispensable in the assessment of the ligaments, since it provides superior soft tissue contrast and allows for simultaneous evaluation of bony structures in a single examination [104]. In the following section, an overview of elbow ligament injuries and their appearance on various imaging methods are provided.
4.3.1 Ulnar Collateral Ligament Injury and Valgus Extension Overload
Valgus extension overload is a spectrum of symptoms that are commonly seen in competitive overhead athletes [105]. Large valgus and extension forces in the acceleration phase of throwing lead to major tensile stress on medial structures, compressive forces on the lateral structures (see Sect. 4.2.2), and shear forces posteriorly (see Sect. 4.2.4). These chronic tensile forces lead to inflammation, microtearing, and laxity of the ligament, which may progress into disruption of the UCL. Less commonly, the UCL may be injured after traumatic elbow dislocation [105].
Plain radiographs may not provide any direct information on ligamentous injuries, but can be indirectly supportive if focal calcifications of the UCL are present [106, 107]. When compared to the normal appearance of the UCL on US, UCL sprains show thickening, decreased echogenicity, and hyperechoic areas demonstrating local calcifications [31, 107]. A completely ruptured UCL appears as a hypoechoic band surrounded by fluid.
On normal axial MR images, the anterior band of the UCL has uniform low signal intensity on T1W and T2W images. However, a completely normal UCL on MRI in a competitive throwing athlete is rarely seen [108]. Adaptations in response to forces in throwing include thickening of the anterior band of the UCL and posteromedial subchondral sclerosis of the trochlea. Therefore, MRI ought to be used to differentiate between acute versus chronic injury and to observe the degree of remodeling of the chronic ligament deformity [109]. Ruptures, sprains, laxity, or other irregularities manifest as a discontinuity with hyperintense fluid filling the hiatus on both T1W and T2W images [14, 104]. Avulsion fracture of the medial epicondyle may be present.
MR arthrography may be of particular benefit when partial-thickness tearing is suspected, since it improves the sensitivity of detecting such tears [18]. In case of a partial-thickness tear, the so-called T-sign may demonstrate increased signal intensity at the distal insertion near the sublime tubercle [14, 110].
4.3.2 Dislocation of the Elbow Joint
Dislocation of the elbow is the most common dislocation in children and the second most common dislocation in adults (after dislocation of the shoulder) [111]. The elbow owes its stability to the osseous architecture of the ulnohumeral joint, which provides the most stability in the anteroposterior direction. The surrounding capsuloligamentous and musculotendinous aspects (including the collateral ligaments, joint capsule, and adjacent muscles) provide further stability. If these components are disrupted by trauma, elbow dislocation may result.
Dislocations of the elbow can either be simple or complex depending on the absence or presence of associated bony injury, respectively. Simple dislocations are described by the direction of the dislocated ulna relative to the humerus. Posterior displacement occurs in over 90 % of cases, with posterolateral dislocation as its most common subtype [112]. The injury mechanism is considered to be a combination of axial compression, supination, and valgus stress, often seen in FOOSH-type injuries [103]. Lateral and anterior displacements are rare and may result from a direct posterior blow to a flexed elbow [113]. Bony injuries of the olecranon and avulsion of the medial and lateral condyles and epicondyles can be present. Complex dislocations with combined fractures of the radial head or neck and the coronoid process are referred to as the terrible triad (see Sect. 4.2.1) [57, 102].
Accompanying ligamentous and capsular disruption can be described according to the Horii circle [103]. Stage 1 involves disruption of the LUCL with posterolateral rotatory subluxation of the ulna. In stage 2, the coronoid places on the trochlea (i.e., incomplete dislocation) and the other adjacent lateral ligaments are torn, including anterior and posterior aspects of the joint capsule. Finally in stage 3, the elbow is completely dislocated with the coronoid located posteriorly to the humerus. The MCL may be disrupted only posteriorly (stage 3A) or completely (stage 3B). Thus, elbow dislocation is the result of a posterolateral rotatory subluxation followed by a total disruption of the surrounding soft tissue from the lateral to the medial side [102].
Posterolateral dislocation can lead to permanent valgus instability that correlates with a worse overall clinical and radiographic result. All treatment options are therefore primarily aimed at restoring functional elbow stability [102]. Simple dislocations may be treated nonoperatively after reduction under adequate muscular relaxation and appropriate analgesia. To prevent joint contractures, definitive management involves limited mobilization and early active range of motion [114]. Complex fracture-dislocations require operative management with fixation of fractures and repair of damaged surrounding soft tissues. Damage to the brachial artery or median and ulnar nerve must be ruled out, although neurovascular injury is uncommon in the setting of a FOOSH injury [103].
Anteroposterior, lateral, and oblique radiographs should be obtained to determine the direction of the dislocation and the potential presence of associated fractures. An intact radiocapitellar line should be evident on all views, since this is no longer aligned in posterior elbow dislocations [8]. Post-reduction radiographs are required to ensure correct positioning of the elbow.
4.3.3 Chronic Insufficiency of the LCL: Posterolateral Rotatory Instability
Elbow dislocation from a FOOSH trauma poses a substantial risk for recurrent elbow instability, since the stabilizing architecture of the surrounding ligaments, the radial head, and the coronoid process can be significantly disrupted. This condition has also been reported following coronoid insufficiency, radial head excision, or steroid injections for lateral epicondylitis [18].
Several criteria are used to classify the degree of the instability: the articulation(s) involved, the direction of the displacement (valgus, varus, anterior or posterolateral), the degree of displacement (subluxation or dislocation), the timing of displacement (acute, chronic or recurrent), and the presence or absence of associated fractures [103]. The most common type of chronic elbow instability is posterolateral rotatory instability (PLRI) [117]. PLRI implies a dislocation by which external rotation of the radius and the ulna relative to the distal humerus results in posterior displacement of the radial head relative to the capitellum. Contrary to isolated dislocation of the radial head, the radioulnar joint does not dislocate because the annular ligament is not affected [118].
The lateral ligament complex limits external rotation of the radius and ulna relative to the humerus and is therefore considered the weakest link in the pathogenesis of PLRI [102]. However, the medial collateral ligament may contribute as well [119, 120].
The diagnosis is made clinically based on the patient’s history and physical examination. Patients with PLRI often have a history of ulnohumeral dislocation; recurrent symptoms of lateral pain, locking, clicking, snapping, or popping can be present. The feeling of instability mostly occurs when the elbow is actively brought from flexion into extension with the forearm in supination. Several specific apprehension tests are available to provoke these symptoms [118, 121]. During the lateral pivot-shift maneuver, the elbow is in supine position and mild valgus stress is applied while the elbow is flexed. The test is positive if apprehension or frank subluxation of the radius and the ulna (rotating away from the humerus) occurs [122]. The posterolateral rotatory drawer test involves overhead placement of the elbow in 40° of flexion. Subsequent application of an anteroposterior force on the ulna and the radius (with the forearm in external rotation) will subluxate the forearm away from the humerus on the lateral side, pivoting on the intact medial ligaments [122]. A more adequate evaluation of instability by these tests may be performed with the patient under anesthesia. The radial head then visibly subluxates posteriorly, whereas apprehension occurs when the patient is awake.
The primary treatment goal in patients with PLRI is to restore elbow stability. Nonoperative measures are applied in the first days after reduction. These measures include both splinting of the arm as well as rehabilitation to strengthen the surrounding musculature [123]. If unsatisfactory results are yielded by conservative management, surgical treatment may be considered. The majority of surgically treated patients encounter satisfactory outcomes regarding elbow stability [118]. Surgical management aimed at the reconstruction of ligaments can be performed either open or arthroscopically [123]. Deficiency of the radial head or coronoid may require bony reconstructions. In that case, computed tomography is of particular use to delineate complex fracture patterns and to assist in surgical planning [115].
Plain radiographs are used to demonstrate changes in the alignment of the elbow by reviewing the integrity of the radial head, coronoid process, and capitellum. The drop sign, indicative for PRLI, represents ulnohumeral separation on lateral radiographs [124]. Posterior displacement of the radial head in relation to the capitellum may be visible as well.
Although MRI has been well established as an effective method for the assessment of ligamentous injury to the LCL, the role of MRI in the diagnosis of PLRI remains questionable [121, 125]. However, examination through MR arthrography is advantageous if uncertainty about the diagnosis remains even though PLRI is suspected [123]. Arthrography reveals laxity of the LCL, widening of the lateral joint space, and osteochondral lesions at the radiocapitellar joint [18, 118].
4.3.4 Monteggia Injury of the Forearm
The ulna and the radius act as a single functional unit through binding via the interosseous membrane and ligaments in the forearm. As a consequence, hyper-pronation injury with fracture of the ulna is often accompanied by a dislocation of the proximal radioulnar joint. This combination of injuries was first described by Monteggia in 1814 and further classified by Bado [126]. Depending on the location of displacement of the radial head, four types can be distinguished (see Table 4.7).
Type | Description |
---|---|
I | Anterior dislocation of the radial head and fracture of the ulnar shaft with anterior angulation |
II | Posterior dislocation of the radial head and fracture of the ulnar shaft with posterior angulation |
III | Lateral dislocation of the radial head and fracture of the ulnar metaphysis |
IV | Anterior dislocation of the radial head, fracture of the proximal third of the radius and ulna |
Since the long-term range of motion of the elbow is seriously threatened in Monteggia injury, early recognition is important [127]. Pediatric patients may sustain injuries slightly different to Monteggia injury, including plastic deformation, incomplete or greenstick fractures, and ulnar metaphyseal fractures [127]. Although conservative management can be successful in the younger population, operative treatment is warranted for the majority of adults [127, 128]. The treatment goal is to restore the cooperative functioning of the radius, ulna, and their associated articulations.
Radiographic examination should comprise AP, lateral, and oblique views of both the forearm and the wrist. The distal forearm should be evaluated for displacement of the ulna relative to the radius. The radiocapitellar line must accurately be assessed in the proximal forearm, since it may seem normal due to concurrent displacement of the ulnar shaft [5].
4.3.5 Isolated Dislocation of the Radial Head
Isolated dislocation of the proximal radius, also termed nursemaid’s elbow or pulled elbow, is the result of a sudden pull on the arm. This longitudinal traction force with the forearm in pronation and extension pulls the radial head trough the annular ligament. Due to relative laxity of the annular ligament, this injury is common in children aged 0–5 years [129]. After the age of 5 years, the annular ligament is stronger and less likely to tear or be displaced. Generally, the diagnosis is based on the clinical presentation. The injured child is likely to not use the affected arm and holds it in pronation, mild flexion, and abduction against the body. Radiography (AP view) should be considered if the diagnosis is equivocal, if the mechanism of injury other than a pull is suspected, or if reduction attempts are unsuccessful [130].
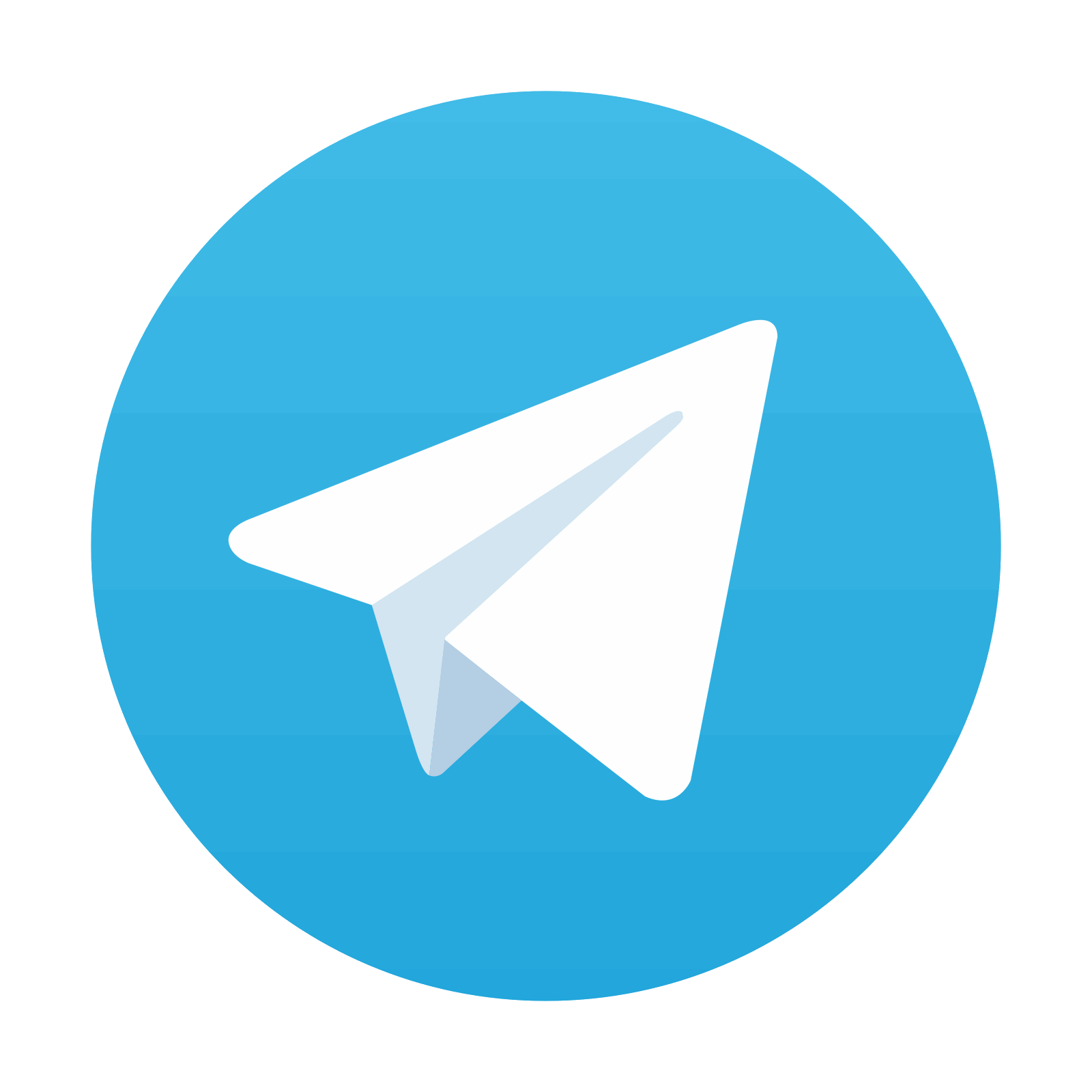
Stay updated, free articles. Join our Telegram channel

Full access? Get Clinical Tree
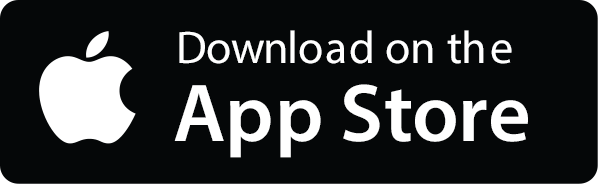
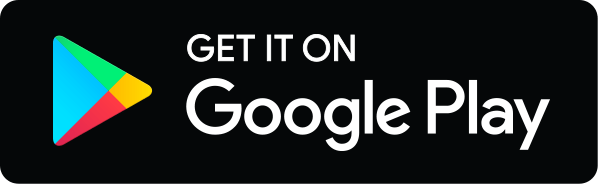