© ISAKOS 2017
C. Niek van Dijk, Philippe Neyret, Moises Cohen, Stefano Della Villa, Helder Pereira and J. Miguel Oliveira (eds.)Injuries and Health Problems in Football 10.1007/978-3-662-53924-8_99. Imaging in Football Lesions
Sérgio Rodrigues-Gomes1, 2, 3 , José Carlos Vasconcelos3 , Pedro Portugal3 , Rui Aguiar3 , Abdalla Skaf4, 5 and Nuno Sousa6, 7
(1)
Clinica do Dragão, Espregueira-Mendes Sports Centre – FIFA Medical Centre of Excellence, Porto, Portugal
(2)
Dom Henrique Research Centre, Porto, Portugal
(3)
Smic Group, Braga, Portugal
(4)
Hospital do Coração, São Paulo, Brazil
(5)
Musculoskeletal Department, Clínica Alta Excelência Diagnóstica, São Paulo, Brazil
(6)
Life and Health Sciences Research Institute (ICVS), School of Medicine, University of Minho, Braga, Portugal
(7)
ICVS/3B’s – PT Government Associate Laboratory, Braga/Guimarães, Portugal
Keywords
Sports medicineImagingFootballRadiography9.1 Radiologic Perspective
Sports medicine is one of the most rapidly growing subspecialties in orthopedics. It has been estimated that 25% of patients seen by primary care physicians complain of musculoskeletal problems, many of which are sports or activity related [1]. Sports injuries often generate nonspecific symptoms and clinical findings, demanding further imaging investigations for accurate diagnosis and optimal treatment planning.
Over the last 10 years, imaging techniques have become increasingly important as a diagnostic tool for sports injuries without replacing the traditional methods of management [2, 3].
The discipline of musculoskeletal radiology has evolved into a major imaging subspecialty in recent years since the first use of X-rays to diagnose fractures. Musculoskeletal radiology expertise has experienced enormous developments in diagnostic sensitivity and specificity and in image-guided treatment options, in addition to technologic advances far beyond X-rays. Advances in cross-sectional imaging such as CT and MR imaging and educational and research endeavors have contributed further to the growth of musculoskeletal radiology as a distinct subspecialty.
Diagnostic imaging plays an increasingly important role in the detection, management, and follow-up of sports disorders.
The use of a wide range of imaging modalities such as routine radiography and ultrasound, as well as advanced imaging modalities such magnetic resonance imaging (MRI) and computed tomography (CT), allows an accurate diagnosis of a broad range of osseous, articular, and soft tissue abnormalities.
However, over-imaging can cause problems in high-level athletes, who have easy access to imaging modalities.
The choice of the imaging modality depends on multiple factors inherent to the radiologist, athlete, and type of lesion, and the optimal imaging process may not exist and should be individually tailored.
While diagnostic imaging allows for accurate characterization of a lesion and local anatomy, it must be emphasized that clinical correlation is mandatory to avoid diagnostic discrepancies and promote optimal athlete management. Good communication between the radiologist and the sports physician is essential.
9.2 Role of Imaging
The athlete’s population differs from the normal population. In sports medicine, especially elite sports medicine, there is always the dichotomy between lesion severity and the pressure of time to resume to play.
Radiological imaging is often required to obtain accurate diagnosis in sports injuries. Even if symptoms and clinical findings in sports injuries are specific, sports medicine physicians often request additional and costly imaging more easily, as they often need a more rapid and accurate diagnosis for lesion severity grading and to optimize treatment planning [4]. Imaging techniques are also readily used to monitor ongoing pathology and to facilitate return-to-play decisions [5].
Imaging can play an important role allowing a fast and accurate diagnosis, helping in evidence-based decision for conservative versus surgical treatment, and also by demonstrating to the athlete the presence of the lesion and reinforcing his adherence to treatment. Imaging can also guide treatment interventions.
The sports medicine radiologist can provide confirmation of specific injury, monitoring of the healing process and the return to play, screening and evaluation before competition/signing, and technical assistance in invasive procedures.
The radiology department of sports medicine should provide different imaging techniques (X-ray, ultrasound, CT, MRI), radiologists with experience in sports medicine imaging, and availability 24 h/7 days with rapid response.
In an ideal setting, the radiologic exams should be available at the training center of the athlete. In recent years, there has been a crescent recognition of the importance of the imaging department within the medical departments of clubs, national and international federations, and Olympic committees. Top European clubs and Olympic Games have been establishing partnerships with imaging medical systems companies to provide radiologic equipment within their own medical and training centers and the Olympic village. It’s predictable the integration of radiologists in the medical department staff of top clubs, federations, and Olympic delegations.
9.3 Overview of Imaging Modalities
This chapter aims to review the role of imaging techniques available to the diagnosis and grading of injuries inherent to football practice, enlightening their specific advantages and limitations and delineating possible imaging strategies and pathways to evaluate most frequent problems and injuries in football, with additional practical guidelines that may be useful in daily clinical practice.
9.4 Conventional Radiology (CR)
Plain radiography is the initial screening examination for osseous disorders due to its ability to visualize osseous structures with high spatial resolution, low cost, and widespread availability [6].
The characterization of the cortical bone is excellent, but the trabecular bone is underdiagnosed. Bone lesions producing cortical disruption, namely, fractures, are relatively easily visualized. Bone loss limited to the trabecular bone from osteoporosis is more difficult to characterize.
The presence of metal is not a limiting factor for CR, allowing assessment of the postoperative bone lesions safely and without artifacts.
CR has important limitations in the evaluation of soft tissue lesions due to poor contrast resolution. It may play a role in the evaluation of the presence of calcifications within the lesion, for example, in myositis ossificans lesions.
Indications for radiography include trauma, pain, instability, impingement, infection, preoperative or postoperative evaluation and/or follow-up, and evaluation of soft tissues in an extremity (e.g., suspected foreign body) (ACR–SPR–SSR Practice Parameter for the Performance of Radiography of the Extremities [7]).
The minimum recommended views in routine circumstances most often include two orthogonal perpendicular projections. Views may be modified for any given clinical situation. Additional views (e.g., oblique views, stress views) may be warranted as part of the initial examination, or after review of the initial images, to clarify suspected pathology. In the evaluation of the shaft of a long bone, the X-ray should include both proximal and distal joints.
While standard radiographs are a static form of imaging, stress radiography is employed to dynamic evaluation, allowing assessment of alignment and stability. These stressed views may demonstrate misalignment or signs of instability not visualized in neutral position.
CR plays an important role in assessing bone position following the treatment of a fracture or dislocation, in monitoring the progress of fracture healing with callus formation and in the diagnosis of fracture complications or complications arising from treatment. Two or more views are usually required to accurately assess bone position after any treatment procedure.
There are numerous normal anatomical variants which may mimic fractures. Correlation of X-ray findings with clinical features is often useful to eliminate significant injury. Some fracture mimics have characteristic features.
Conventional arthrography (X-ray joint evaluation after injection of intra-articular contrast medium) has evolved during the last century from crude techniques with postprocedural radiographic imaging to modern CT and MR arthrographic techniques. Arthrography saw its widest use in the 1960s and 1970s, but indications for its use in many joints decreased significantly after the introduction of cross-sectional imaging modalities such as CT and MR imaging. Arthrography nowadays is only performed as part of CT or magnetic ressonance arthrography (MRA) [8].
CR uses ionizing radiation. Medical ionizing radiation has great benefits and should not be feared, especially in urgent situations. Radiological dose and risk depends on good methodology and quality control, taking into account the possible risk from radiation exposure and the diagnostic image quality necessary to achieve the clinical objective (“as low as reasonably achievable”).
The prescribing physician must justify the examination and determine relevant clinical information before referring the patient to a radiologist. Indications and decisions should reflect the possibility of using nonionizing radiation examinations, such as MRI or ultrasonography. Repetition of examinations should be avoided at other institutions.
9.5 Ultrasound (US)
US technology is rapidly advancing and being refined, and is aimed at both increasing image quality and opening new fields of applications.
Acute skeletal muscle injury is one of the major causative factors for loss of playing time in all athletes and is the most common injury in professional football. A recent study on male professional footballers showed that injuries to muscle represent more than 30% of all injuries and are responsible for approximately one quarter of total injury absence. Over 90% of muscle injuries affect the four major muscle groups of the lower extremity: hamstrings, adductors, quadriceps, and gastrocnemius. Injury to the hamstring muscle group is reported to be the most common injury subtype [9].
Over the last 15 years, musculoskeletal ultrasonography has become an important imaging modality used in sports medicine, being considered the sports medicine stethoscope. This technique has become an indispensable tool in the clinical management of sports injuries and degenerative and traumatic lesions of the articulations and periarticular soft tissues. With the rapid development and sophistication of this modality, essential information for a better understanding of the pathophysiologic assessment of many disorders has been established, allowing crucial decisions regarding treatment planning and monitoring the effects of therapy.
Major advantages for the diagnostic accuracy of US in sports medicine practice are the ready availability, portability, affordability, speed, real-time and dynamic imaging, high spatial resolution, and absence of ionizing radiation. The possibility of using Doppler imaging is another advantage.
US main disadvantages are the inadequate characterization of bone and deep structures, operator dependency, and the short field of view. Despite the former, ultrasound is sensitive to rule out cortical fractures of superficially located bones and is more accurate to detect rib fractures compared to radiographs [10].
US is able to recognize the internal muscle architecture. Intramuscular vessels coursing within the hyperechoic septa are visible on color and power Doppler imaging. The outer muscle fascia (epimysium) appears as a well-delineated echogenic envelope circumscribing the hypoechoic muscle. Large hyperechoic septa (aponeuroses) directed within the muscle belly can be seen arising from it. In complex muscles, an individual hyperechoic fascial sheath surrounds each muscle belly thus helping the examiner to recognize the different heads. The interstice between juxtaposed fasciae of two adjacent muscles appears as a hypoechoic band and corresponds to loose connective tissue that allows some sliding of the muscles during contraction [11].
Dynamic US scanning performed during muscle contraction can show changes in size and relationship of fascicles and fibro-adipose septa.
High-frequency (7–18 MHz) linear-array probes are used to perform musculoskeletal US examinations. Broadband transducers use a spectrum of frequency distribution (i.e., 12–5 MHz) instead of a single fundamental frequency (i.e., 10 MHz): the high-frequency components tend to increase the intensity maximum in the focal zone but cause a prompt decrease in intensity with depth, whereas the low-frequency components extend the penetration depth [12]. Other systems use the total transducer bandwidth for the transmitted pulse and then adjust the receiver bandwidth to lower frequencies as deeper depths are sampled. These systems give increased flexibility to the US examination, enabling the same transducer to change the image acquisition parameters during scanning based on the desired clinical information. In musculoskeletal imaging, this is particularly important when the study focuses on both superficial (i.e., subcutaneous tissue planes) and deep (i.e., muscle tissue layers) tissues in the same study and body area to be explored.
A variety of linear-array transducers, including large (>40 mm), medium-sized (<40 mm) and small-FOV (hockey-stick-shaped) probes, are currently available in the frequency range used for musculoskeletal examinations. Selection of the adequate transducer primarily depends on the frequency. Hockey-stick probes are the best choice for imaging small superficial structures at sites in which the skin surface does not allow adequate contact with larger probes (i.e., soft tissues adjacent to bony prominences) or while performing dynamic maneuvers, but they are characterized by a restricted field of view. Compared with small transducers, high-frequency large-diameter transducers tend to have a large near-field beam width leading to a poor lateral resolution at shallow depths. Because they maintain beam shape to greater depths with less divergence of the US beam, they have the best potential for imaging deep-seated structures [11].
Recent technologic innovations in US have resulted in improved diagnostic performance for the evaluation of the musculoskeletal system, including wideband Doppler imaging, spatial compound imaging, extended field-of-view imaging, steering-based gray-scale imaging, elastography, and 3D imaging [11].
The ability of high-frequency color and power Doppler to detect low flow states in superficial structures and to correlate hyperemic changes with structural abnormalities has allowed for the noninvasive study of blood flow and vascularity within anatomic structures and lesions, opening new perspectives in the evaluation of a variety of musculoskeletal disorders.
The most studied example is the detection of intra-tendon neovascularization in tendinopathy, considered of diagnostic and prognostic value, related to clinical outcome, and the exclusive target of some therapeutic interventions [13–16], but with discrepant results, and recent studies have been questioning the value of neovascularization in tendinopathy [17–19].
Spatial compound imaging indicates an acquisition mode in which the information is obtained from several angles of insonation and is combined to obtain a single image [20, 21].
The advantages of compound mode are many, including reduction of image artifacts (e.g., speckle, clutter, noise, angle-generated artifacts), sharper delineation of tissue interfaces and better discrimination of lesions over the background, as well as improvement in detail resolution and image contrast. In the musculoskeletal system, compound imaging leads to an improved delineation of structures composed of specular echoes, such as tendons and muscles [21].
The extended field-of-view technique contributes to an improved presentation of the US information for the referring physician [22–25], displaying the full extent of an abnormality and showing its relationship with adjacent structures on a single image.
Three-dimensional acquisition can be achieved with US using either 2D conventional transducers equipped with a small electromagnetic positional sensor or dedicated “3D volume transducers,” which are larger than standard probes and more difficult to handle but have the advantage of providing more exact assessment of each scanning plane.
Ultrasound elastography (EUS) is a method to assess the mechanical properties of tissue, by applying stress and detecting tissue displacement using ultrasound. There are several EUS techniques used in clinical practice; strain (compression) EUS is the most common technique that allows real-time visualization of the elastographic map on the screen. There is increasing evidence that EUS can be used to measure the mechanical properties of musculoskeletal tissue in clinical practice, with the future potential for early diagnosis to both guide and monitor therapy [11].
Due to the excellent spatial resolution and definition of muscle structure, US has become an indispensable tool for evaluation of muscle pathology, not only for the diagnosis of the lesion, allowing an accurate characterization most of the times, especially in superficial muscles, but also for the follow-up of lesions during healing process, with detection of healing complications such as fibrosis, hematomas/seromas, hernias, or myositis ossificans.
The ideal time for the US examination of fresh traumatic muscle lesions is between 2 and 48 h after trauma. Before 2 h, the hematoma is still in formation. After 48 h, the hematoma can be spread outside of the muscle [26]. However, with some muscles it can stay for much longer. It is recommended that for lesions in the hamstrings, the US examination be done as soon as possible after the 2 h delay. For rectus femoris and gastrocnemius lesions, the examination can be postponed for as long as 2 or 3 days, or even longer sometimes [27].
Dynamic US study may be very helpful to the correct diagnosis, e.g., to search for muscle hernia (during muscle contraction) or to evaluate the snapping hip syndrome (during hip flexion and lateral rotation). To avoid artifacts or pitfalls, comparison with the contralateral side may be necessary.
US has intrinsic limitations in the assessment of the bone. In some applications, however, it can be useful to assess selected bone disorders, especially if performed as a complement to standard radiographs [28]. With US, the interface between the soft tissue and cortical bone is highly echogenic because of an inherent high acoustic impedance mismatch [29]. The bone cortex appears as a regular continuous bright hyperechoic line with strong posterior acoustic shadowing and some reverberation artifact.
US can detect cortical outgrowths (exostoses, anatomic variants), defects (fracture, osseous tunnels, impact lesions), and erosions. Some authors have suggested that the process of fracture healing can be followed with color Doppler imaging and spectral analysis [30].
Bone abnormalities seen at US can easily be correlated with clinical findings and can suggest the requirement for additional radiographic views or other imaging studies if further evaluation is warranted.
The indications for joint US are rapidly expanding due to the refinement of high-resolution transducers and to the fact that both radiologists and clinicians are increasingly aware of the potential of US [31].
US allows visualization and characterization of superficial ligaments, intra-articular fat pads, and intra-articular fluid. Some ligaments located in the central portion of joints (i.e., the interosseous tarsal sinus ligaments and the cruciate ligaments of the knee) cannot be visualized with US because of the overlying osseous structures.
In most joints, small amounts of normal intra-articular fluid can be detected in the articular cavity by means of high-resolution US.
Many joints contain fibrocartilaginous structures, including the meniscus in the knee, the labrum in the hip and the shoulder, the triangular fibrocartilage in the wrist, and the volar and plantar plates in the hand and foot. Because of their deep location and close contact with the bone, these structures can be evaluated with US only in part and not reliably. However, some conditions involving the superficial part of these structures, such as an extruded meniscus, a meniscocapsular detachment with fluid intervening between the capsule and the fibrocartilage or a meniscal ossicle can be inferred on US.
In contrast to the results of fibrocartilage evaluation, US has proved to be an effective modality for diagnosing parameniscal and paralabral cysts [32–34].
Ligament tears can be demonstrated with US at different sites, including the ankle and foot [35, 36], the wrist and hand [31, 37–39], the knee [40–42], and the elbow [43]. The US features of a torn ligament vary depending on whether the lesion is acute or has healed. In acute phases, a partially torn ligament appears swollen and hypoechoic but continuous; an anechoic band over the superficial aspect of the ligament may be observed representing reactive soft tissue edema [35]. In complex ligaments, US can distinguish the abnormal hypoechoic portion of the ligament from the unaffected one retaining a normal appearance. In acute complete ruptures, a hypoechoic cleft reflecting the hematoma can be detected through the ligament substance, and the free ends of the severed ligament may appear retracted and wavy. In doubtful cases, the ability to assess the ligament dynamically is a definite advantage of US: under stress, a normal ligament tightens preventing excessive widening of the joint space; if the ligament is torn, a paradoxical movement is obtained reflecting joint instability [44, 45]. In chronic partial tears, the ligament always appears thicker than normal on US images. Calcifications within the ligament substance in old tears and irregularities of the bony insertions in avulsion injuries may be observed [45]. A typical example is the Pellegrini-Stieda syndrome (calcification of the proximal end of the medial collateral ligament of the knee).
One of the major limitations of US in evaluating osteoarthritis is the incomplete evaluation of the cartilage surface, which is, for the most part, masked by the ends of opposing bones. This is true for both tight and large joints. In the knee, for instance, articular cartilages that are vulnerable to tears and ulcerations are mainly located at the posteroinferior aspect of the femoral condyle and on the lateral facet of the patella: both surfaces are barely evaluated with US. Similarly, geodes (subchondral cysts) are not visible at US because they are completely surrounded by the bone. On the other hand, osteophytes can be readily appreciated as beak-like bone projections covered by hypoechoic cartilage adjacent to the joint line. They increase the surface area of the articular cartilage, thus lessening the stress and loading forces that are experienced by the joint and, at the same time, increasing its stability: typical locations of osteophytes are the posterior humeral head, the internal femorotibial, and the anterior tibiotalar joints [46].
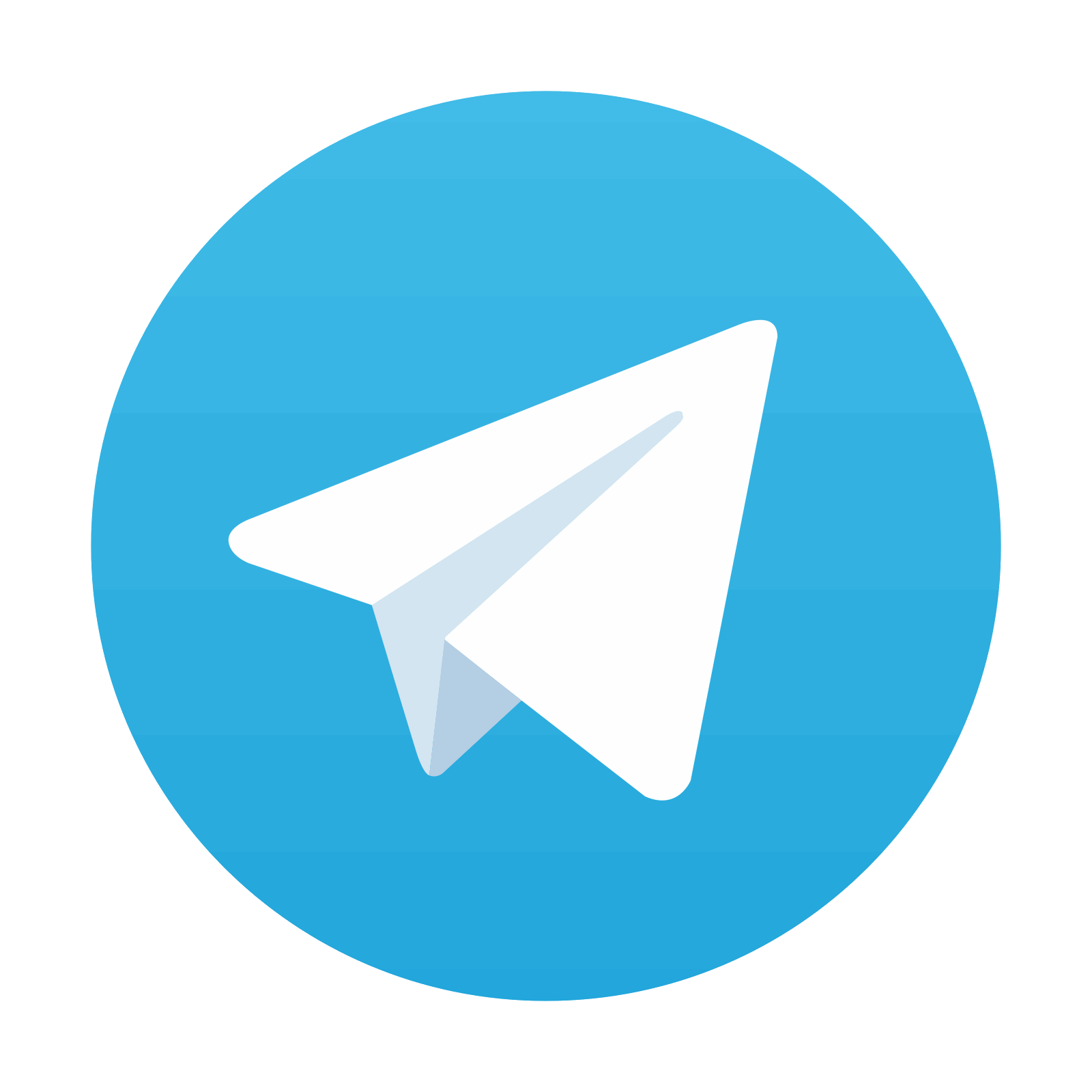
Stay updated, free articles. Join our Telegram channel

Full access? Get Clinical Tree
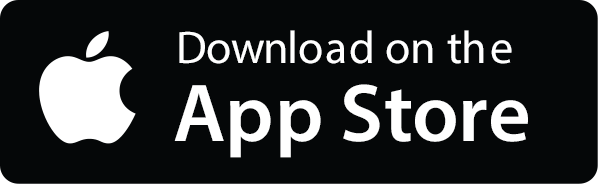
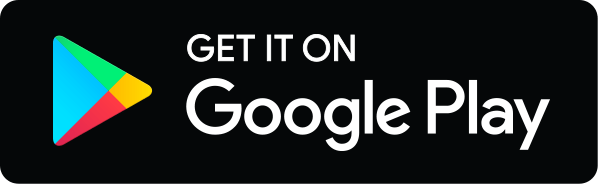