Fig. 1
Grade 1 strain. Axial fat-suppressed T2WI through the leg shows feathery edema (arrow) in the medial gastrocnemius muscle
Tears are graded because the severity of the injury has implications for return to play, and not surprisingly the greater the injury, the longer it takes to recover. A study of professional soccer players with acute hamstring pain found that players with normal MR imaging examinations had an average of 8 days to return to play, players with Grade 1 injuries had an average of 17 days to return, Grade 2 injuries had 22 days to return, and Grade 3 had 73 days to return [19]. The length and area of involvement on MR are the best predictors of time to return to play [20].
Intramuscular hematomas have variable appearances, partly influenced by the time course. Acute hematomas may be low to isointense to muscle on T1WI, and high signal intensity on T2WI. After several days, they may show intermediate to high signal intensity on T1WI due to proteins and methemoglobin. Both acute and subacute hematomas may have heterogeneous signal intensity. After several weeks they may appear more like fluid, with a low signal intensity rim due to deposition of hemosiderin [9, 21].
The optimum outcome for healing of muscle tears is a return to normal architecture and signal intensity, but low signal intensity fibrosis may occur at the site of healed tear. An area of scar or fibrosis in the muscle may place the muscle at risk for recurrent tear because the fibrosis is stronger than the adjacent normal muscle and acts as a stress riser [9] but a recent study of athletes with and without fibrosis following initial hamstring injuries found no statistical difference in rate of recurrent tear [22].
Fascial Herniation
Traumatic tears of the myofascia or regions of fascial weakness may allow the muscle to focally herniate. The lower extremity is the most common site of muscle hernias [9, 15, 23]. The herniation usually presents as a painless bulge that the patient can reproduce with muscle contraction, but occasionally the herniation may be tender or painful. On imaging, the muscle looks normal at rest. Dynamic scanning is usually necessary to demonstrate the herniation, and sonography, by virtue of its real-time nature, is well suited to visualize these abnormalities [23–26].
Myositis Ossificans
Myositis ossificans refers to the development of heterotopic ossification in skeletal muscle. Common causes include contusion, surgery, burns, and paralysis, but in up to 40% of cases the patient is not able to recall a precipitating event [27]. The patient typically presents with pain and swelling. MR imaging in this acute phase will show extensive muscle edema surrounding a poorly defined mass. Although a soft tissue sarcoma might be considered in the imaging differential, the extensive soft tissue edema is much more suggestive of myositis ossificans [9, 15]. Over the course of several weeks the edema will subside and a zone of peripheral ossification will develop (Fig. 2). This mineralization may be detected earlier with CT than with MR imaging. The more mature heterotopic ossification will exhibit areas of T1 hyperintensity reflecting marrow [9, 15, 27]. The maturing focus may incite periosteal reaction in an adjacent bone, and may eventually become incorporated into the adjacent bone [27].


Fig. 2
Myositis ossificans. Axial fat-suppressed T2WI through the thigh shows the low signal intensity zonal ossification (arrow) in the vastus intermedius muscle with surrounding high signal intensity muscle edema
Delayed Onset Muscle Soreness
Delayed onset muscle soreness (DOMS) is the term to describe muscle pain and swelling occurring a day or two after intense eccentric muscle contractions, especially in a non-conditioned muscle. It is an over-use injury that demonstrates feathery or patchy T2 signal hyperintensity in the affected muscle or muscles, similar to a Grade 1 muscle strain (Fig. 3). Clinical history and symptomatic relief over the ensuing week help to differentiate DOMS from a Grade 1 strain [9, 15, 28]. Sonography of DOMS shows muscle enlargement with diffuse well-defined hyperechogenicity of the muscle representing edema, and hyperemia using color or power Doppler sonography [29].


Fig. 3
Delayed onset muscle soreness. Axial fat-suppressed T2WI through the thigh in a woman 2 days after doing lunges in the gym for the first time. There is patchy hyperintense edema predominantly in the quadriceps muscles, and to a lesser extent in the hamstrings
Compartment Syndrome
Compartment syndrome is swelling of a muscle in a closed anatomic compartment with resultant elevated pressure causing a disturbance in the muscle’s microcirculation, which in turn can lead to muscle ischemia [15, 28, 30]. Acute compartment syndrome is usually due to fracture or less commonly a muscle contusion (direct blow) or strain, and is a surgical emergency requiring fasciotomy. It is a clinical diagnosis, based on severe pain out of proportion to the initial injury, pain to palpation and passive stretching, and increased direct intracompartmental pressure measurements. MR imaging is usually not indicated in acute compartment syndrome, as time is of the essence. Delayed or missed diagnosis will lead to irreversible muscle ischemia and necrosis with eventual fibrosis (Volkmann’s contracture) [15].
Chronic exertional compartment syndrome refers to exercise-related or activity-related pain due to elevated intramuscular pressure and transient ischemia, which resolves when the offending activity is stopped [15, 30]. The leg is the most common site of involvement, affecting the anterior compartment most commonly, followed by the deep posterior, lateral, and superficial posterior compartments [30]. Post-exertional MR imaging shows T2 hyperintensity in the affected muscle [30, 31]. Longstanding cases may exhibit fascial thickening, muscle atrophy, and fibrosis [15, 28].
Denervation
A predictable series of events occurs when the peripheral nerve supply to skeletal muscle is abruptly interrupted (say by a complete laceration or experimental sectioning) [32]. For the first week, the muscle maintains its normal gross and microscopic appearance. Additionally, electromyography of the affected muscles is relatively insensitive in the acute setting [33]. No morphologic changes initially occur on MR or ultrasound during this period [34]; nevertheless, early shifts of fluid from the intracellular to the extracellular spaces in the muscle can be detected with MR diffusion techniques [35, 36].
After approximately two weeks, subacute denervation will become apparent on standard MR images. Specifically, increased extracellular fluid results in prolongation of both T1 and T2 values in muscle [37], which will be most conspicuous on STIR images [34]. This edema pattern will involve the entire muscle belly relatively homogeneously (an exception is in muscles that have a dual nerve supply) and does not affect the fascia (a distinguishing characteristic from traumatic and many inflammatory muscle etiologies). However, what best distinguishes muscle denervation from other conditions is its distribution: denervation will involve the muscles supplied by the affected motor nerve (Fig. 4) [38]. Thus mapping the muscles involved and those spared, can predict the location of a lesion in the neuroaxis [39]. At that point, electrophysiology or imaging studies can be directed to that area for diagnosis of extrinsic nerve compression (due to a tunnel syndrome or a mass like a ganglion cyst, for example) or intrinsic nerve lesions (e.g., a nerve sheath tumor).


Fig. 4
Subacute denervation in a 47 year-old woman. A fat-suppressed T2-weighted image shows high signal intensity throughout the forearm extensor muscles and the supinator, corresponding to the radial nerve distribution. Note the lack of fascial edema. Compression of the nerve was found proximally, at the lateral intermuscular septum or the triceps
This approach of predicting the location of a nerve insult based on the pattern of muscle involvement works for single peripheral nerve lesions as well as more complex injuries [38]. Insults to the spinal cord (Guillain-Barré syndrome or transverse myelitis, for example), limb nerve supply (polio, traumatic nerve root avulsions), brachial or lumbar plexus (Parsonage-Turner syndrome, radiation plexopathy), individual nerve roots (disc herniation), and even peripheral nerve branches (nerve lacerations) will produce predictable distributions of muscle involvement. Similarly, diseases that cause polyneuritis or mononeuritis multiplex will involve several motor nerve distributions.
If reinnervation does not occur, after several months chronic denervation sets in. The muscles become atrophic with decreased bulk and replacement of the muscle fibers by fat, which is recognizable as high signal intensity tissue, isointense to subcutaneous fat, on T1-weighted and other short-TE MR sequences [34]. It is important not to rely on an MR protocol that consists entirely of fat-suppressed sequences (which is not uncommon in some practices for MR arthrograms), because fat-replaced and normal muscle appear essentially identical on these images. On ultrasound, fatty replacement is characterized by generalized increased echogenicity of the muscles compared to spared muscles and compared to the internal septa [3]. Note that fatty muscle atrophy is a common end-stage process in multiple conditions including following complete ruptures of either the myotendinous junction or the tendon attaching the muscle to bone, for example in chronic rotator cuff tears. End-stage ischemia, inflammatory, and myopathic disorders also lead to fatty replacement (see below). In most cases replaced muscle will be decreased in bulk, but there are circumstances where fatty infiltration is so great that the muscle appears enlarged, a condition called pseudohypertrophy [40–42]. However, as is the case for muscle edema due to denervation, denervation atrophy is recognizable because it occurs in a given nerve distribution.
In reality, the transition from subacute to chronic denervation is not always predictable. Most nerve lesions do not act like acute, complete lacerations. Nerve compression due to a tunnel syndrome or mass occurs gradually over time and may affect the peripheral motor neurons in a nerve earlier and more severely than the central ones. Stretching injuries occur on a spectrum that includes partial or complete disruption of the myelin, endoneurium, axon, and epineurium in varying combinations [43]. Viral, drug, metabolic, nutritional, radiation, ischemic, and other insults can involve different nerves to varying degrees and with an unpredictable time course. And each of these conditions has different potential for nerve healing, either spontaneously or following removal of the causative agent or nerve repair. Thus, muscle changes of subacute and chronic denervation often coexist on a single imaging study. Research shows that the calculated T2 value of the affected muscles correlates with the severity and reversibility of the nerve insult [44]. Furthermore, sequential MR examinations are useful for predicting the success or failure of interventions like nerve repair, and have added value compared with clinical and electrophysiological monitoring [44, 45]. Specifically, normalization of muscle signal on STIR sequences before fatty infiltration occurs is a sign of successfully reinnervation [46, 47].
Inflammatory Myositis
Inflammatory myositis describes a group of related diseases that are characterized by autoimmune inflammation affecting skeletal muscle, and in some subtypes, other tissues including skin, fascia, cardiac muscle, and lung [48]. Clinically the conditions all share muscle weakness as a cardinal symptom. Age-of-onset, time course, distribution, serologic markers, and extra-muscular involvement differ among the various disorders [49, 50].
There are four or five major categories of inflammatory myositis, several with recognized subtypes [48]. The main types are dermatomyositis, juvenile dermatomyositis, polymyositis, immune-mediated necrotizing myopathy, and sporadic inclusion body myositis. In addition to the clinical and serologic differences among them, each also carries a different prognosis and treatment regimen, making positive identification a key tenet in management [50, 51]. As discussed below, exact diagnosis usually requires muscle biopsy.
Dermatomyositis includes skin involvement, with several possible rashes, most commonly a violaceous discoloration of the eyelids [52]. Proximal thigh weakness usually develops over a course of weeks. Women are affected more often than men. Disease onset may be a harbinger of a carcinoma in the body (with an overall increase risk of 3×) that presents in the first few years after dermatomyositis is recognized [53]. An amopathic form has the typical skin findings without overt muscle weakness. Juvenile dermatomyositis has onset before age 18 [54]. In the juvenile form, skin and subcutaneous involvement may be clinically occult, or may take the form of cutaneous calcification with or without ulceration [55]. Vasculitis in the gastrointestinal tract is also a prevalent feature of juvenile dermatomyositis [54]. Polymyositis is less common than (and lacks the skin findings of) dermatomyositis. Compared to dermatomyositis, it occurs in an older age group, and usually has a more insidious, slower onset. Symmetric weakness of both the proximal upper and lower extremity muscles is typical of polymyositis [49, 50]. The risk of associated cancer is lower in polymyositis compared to dermatomyositis [53].
Sporadic inclusion body myositis is more common than dermatomyositis and polymyositis after age 50 [48]. It has a much slower onset (often years) than the other conditions and is more likely to present asymmetrically and involve the distal extremity muscles early in the disease. There is evidence that buildup of tau and amyloid proteins in the muscle occurs in this condition, analogous to Alzheimer’s disease in the brain [56]. Immune-mediated necrotizing myopathy occurs after a triggering event, like viral infection or drug (including statin) administration [57].
Because of overlap in symptoms and serologic markers (which are only present in 50% of patients), it is frequently impossible to distinguish the various inflammatory myositis conditions from each other [51]. Furthermore, many other conditions—which have different treatments and prognoses—are also characterized by insidious onset muscle weakness. The differential diagnosis is broad and includes metabolic conditions, endocrinopathies (e.g., disorders of thyroid hormone), infectious causes (viral, parasitic, and bacterial pyomyositis), some muscular dystrophies and mitochondrial myopathies, and collagen-vascular conditions like scleroderma [50]. While some of these disorders can be diagnosed or excluded based on clinical, laboratory, and electromyographic criteria, muscle biopsy is always required for a certain diagnosis and classification of inflammatory myositis [51]. A prompt biopsy-derived diagnosis is especially important because institution of specific immunosuppressive therapy early in the disease course can prevent severe, often irreversible muscle damage and weakness in conditions like dermatomyositis and polymyositis [58, 59]. However, because histologic involvement of specific muscles is inconsistent and difficult to predict with certainty, biopsies that are directed at muscles based on clinical weakness are falsely negative in approximately 25% of cases [60–63].
Imaging has three important roles in patients with suspected or confirmed inflammatory myositis. At presentation, MR (or less commonly ultrasound) can guide biopsy to muscles that are most likely to yield diagnostic pathologic findings. Second, once a diagnosis is established and treatment has begun, imaging may be used to monitor the disease for response. Third, in longstanding myositis when a patient develops increasing weakness the clinical dilemma is to distinguish whether they are having a flare of their active inflammation (in which case an increase in medication may be warranted), or whether the disease has progressed to the chronic, burned-out stage, in which case more aggressive medical therapy is not indicated. The key observation in each of these scenarios is whether active or chronic myositis is present.
During the active inflammatory phase of myositis, regardless of the subtype, imaging shows edema in the affected muscles. On ultrasound, edematous muscle fibers are more hypoechoic than usual compared to the hyperechoic septa [2, 3]. However, this ultrasound appearance is neither specific for diagnosis of myositis nor its subtype [64]. Use of an ultrasound contrast agent to separate inflammation from other causes of edema has been suggested to improve specificity [65, 66]. MR is more sensitive than ultrasound for identifying active disease, even with the use of ultrasound contrast agents [51, 65]. Active myositis on MR appears as increased signal intensity in the affected muscles on water-sensitive sequences, especially STIR or chemically fat-suppressed T2-weighted sequences (Fig. 5) [67–69]. High signal intensity in the superficial and deep fascia may be present early in the course of dermatomyositis, including in the amyopathic form [70]. The use of intravenous contrast for MR is controversial: while it does not appear to increase sensitivity compared to STIR imaging, contrast enhancement may increase diagnostic confidence and specificity, allowing distinction of inflammatory conditions from metabolic and neurodegenerative ones [62, 71]. Conversely, in chronic, burned-out disease, fatty atrophy is the predominant imaging finding. Atrophied muscles on ultrasound are increased in echogenicity and decreased in size [66]. On MR, muscle atrophy appears as decreased bulk and replacement of muscle tissue with high signal intensity fat on T1-weighted images, without edema on water-sensitive sequences [67, 72].


Fig. 5
Active polymyositis in a 19-year-old man. There is asymmetric, patchy edema of the muscles of both thighs on this fat-suppressed T2-weighted image. Based on the MR findings, biopsy of the left vastus medialis muscle was performed, leading to the diagnosis
In all forms of inflammatory myositis, muscle involvement—whether in the active or burned-out stages—is patchy in distribution, with some muscles involved and some spared in individual cases. While certain patterns of involvement may be more characteristic of one disease subtype or another, no pattern is characteristic enough to eliminate the need for biopsy [73]. For example, involvement of the vastus lateralis, rectus femoris, and biceps femoris with sparing of the vastus intermedius is frequent in immune-mediated necrotizing myopathy [74, 75], but can be encountered conditions as well. Similarly, disease preferentially affecting the anterior compartment musculature of the thighs and calves but sparing of the rectus femoris, or involving the flexor digitorum profundus muscle in the forearms is characteristic of inclusion body myositis, but not pathognomonic [76, 77]. One exception is in dermatomyositis: edema in the skin and/or subcutaneous tissues in addition to some skeletal muscles suggests dermatomyositis or juvenile dermatomyositis, and in the latter condition, the cutaneous involvement may be clinically occult [55]. Additionally, superficial and deep fasciitis present on MR in the first few months of disease—with or without muscle involvement—appears to be a typical feature of dermatomyositis not shared by the other subtypes [70].
At presentation, the most important role of imaging is to direct muscle biopsy. Because of the patchy nature of these conditions, the false negative rate of a non-imaging-directed muscle biopsy is approximately 23%; this rate can be reduced to 6% by using MR imaging to target a high-yield biopsy target [78]. Muscles most likely to provide diagnostic material in inflammatory myositis are those that are edematous but not fatty infiltrated on MR imaging, and which are close to the surface and easily accessible [78, 79]. Using MR to guide initial muscle biopsies may be cost effective as well [60].
Once treatment has begun, the extent of edema in the thigh muscles on STIR images correlates with serum creatine kinase levels and clinical assessments of disease activit1y [80]. Compared with repeat muscle biopsy, MR findings correlate better with clinical response after 2–6 months of treatment [81]. Some investigators have advocated whole body MR—either using a body coil or multiple smaller coils in an array—to better gauge overall disease activity in inflammatory myositis [63, 73, 82, 83]. A relatively fast screening examination for active disease can be done with large field-of-view, low resolution fast (turbo) spin echo STIR images alone [82]. Adding T1-weighted images and contrast-enhanced images prolongs the study but may add specificity; some of the excess time can be regained by not including the trunk muscles, which does not seem to increase sensitivity [71]. Nevertheless, in clinical practice, a screening examination of just the thighs and calves (or the shoulder girdles, if weakness is much more profound in the upper extremities) probably suffices.
Several experimental MR techniques have been investigated as potential adjuncts in the evaluation of inflammatory myositis. These procedures include diffusion MR imaging, diffusion tensor imaging, MR elasticity, and MR spectroscopy [80, 84–88]. While each has promise, it is unclear whether any currently has added value over morphologic imaging using STIR technique. More recent studies suggest that using MR to calculate T2 measurements and fat fractions in affected muscles might be a way to quantify the subjective information gained from STIR and T1-weighted images, respectively [89].
Congenital Muscle Disorders
Genetic mutations are responsible for myriad congenital myopathies, muscular dystrophies, and metabolic disorders affecting skeletal muscle, each with varied clinical manifestations. In the last decade, researchers have applied modern DNA sequencing and protein synthesis techniques to better sort and characterize these conditions, which now probably number in the hundreds. Management, prognostication, and genetic counseling depend on making the most accurate and specific diagnosis possible. While phenotype analysis, family history, and genetic testing are now the mainstays of the work-up, imaging can play a role in some conditions by limiting the differential diagnosis to streamline laboratory testing. A second emerging role of imaging is in follow-up of the disease course to track severity, response to treatment, and planning of physical therapy.
The hallmark imaging finding for most congenital muscle disorders is fatty infiltration of (selected) muscles groups, identified as replacement of muscle tissue by fat on T1-weighted MR sequences (Fig. 6) or echogenic material on ultrasound [90–92]. Characteristically, many disorders also predictably spare certain muscle groups, which will appear normal or may even show compensational hypertrophy on imaging studies. Pseudohypertrophy of the affected muscles may occur when the amount of fatty infiltration enlarges the girth of the muscle [41, 42]. Clinically, pseudohypertrophy may be difficult to separate from true hypertrophy, but the distinction is trivial with cross-sectional imaging. Much less commonly, muscle edema—easiest to appreciate on STIR images—may be a minor finding in some congenital conditions, or may transiently occur before fatty infiltration.


Fig. 6
Limb-girdle muscular dystrophy in a 28-year-old man. The T1-weighted image of both thighs shows profound fatty infiltration of multiple muscle groups, most severe in the adductor compartment. There is relative sparing of the rectus femoris muscles
Congenital myopathies usually present at or near birth with weakness and hypotonia, but don’t progress or progress very slowly over time; they are most typically classified according to the pattern of morphological muscle abnormality seen microscopically [93]. At least 35 different genes are implicated in the myopathies, with several different mutations in one gene, or combinations of mutations in several genes leading to more than 35 distinct diseases. Muscular dystrophies are characterized by muscle wasting pathologically (in the absence of histologic evidence of another muscle or nerve disorder) [94]. They progress throughout life and are frequently fatal. Dystrophies are grouped according to the incorrectly coded protein complex (at least seven main subgroups exist), with at least 25 responsible genes identified thus far. Inborn errors of metabolism can also negatively affect the function (primarily energy metabolism) in skeletal muscle, usually resulting in some degree of exercise intolerance. These metabolic myopathies include glycogen storage disorders like Pompe disease, conditions where mitochondria do not function correctly including those where the mitochondrial DNA is faulty, and syndromes where proteins involved in oxidative metabolism are faulty; at least eight enzymes and 25 responsible genes have been identified as culprits [95].
For reasons that are not clear, many of the metabolic conditions have particular patterns of muscles that are severely involved, variably involved, and spared [41, 91, 92, 96]. For example, in Duchenne muscular dystrophy—a relatively common X-linked recessive disease due to a mutated gene coding for the dystrophin protein complex [97]—the gluteus and adductor magnus muscles are affected earliest and most severely, other thigh and calf muscles are involved to a lesser degree, and the gracillis is always spared (and frequently hypertrophied) [98, 99]. Other myopathies, dystrophies, and metabolic conditions will characteristically target other sets of muscle groups, often varying with the disease phenotype [100–103].
While whole body MR has been advocated as potentially useful in specific conditions [104], much information can be gained by a simple MR examination of both thighs for most conditions. As is the case for inflammatory myositis, relatively thick-section transverse T1-weighted and STIR sequences usually suffice. The roles that imaging plays in congenital muscle disorders are expanding. Before final diagnosis, demonstrating a characteristic pattern of involvement can suggest specific genetic testing to complement biopsy results [92]. Subtle muscle edema on STIR images may be a sign of very early disease (before fatty infiltration occurs) in some diseases like limb-girdle and Duchenne muscular dystrophy, at a stage where medical therapy may have a greater chance of success [91, 98]. Similarly, in late-onset Pompe disease, enzyme replacement therapy seems to prevent disease progression best when treating patients who show little-to-no fatty muscle infiltration before therapy [105]. In disorders that affect muscles selectively and asymmetrically, MR or ultrasound can direct muscle biopsy [42, 99]. Additionally, progression or remission on sequential imaging studies may be more sensitive than (or at least complementary to) clinical functional assessments [99, 104]. Last, physical therapy can be tailored to strengthening muscles that are shown to be spared on imaging studies [42].
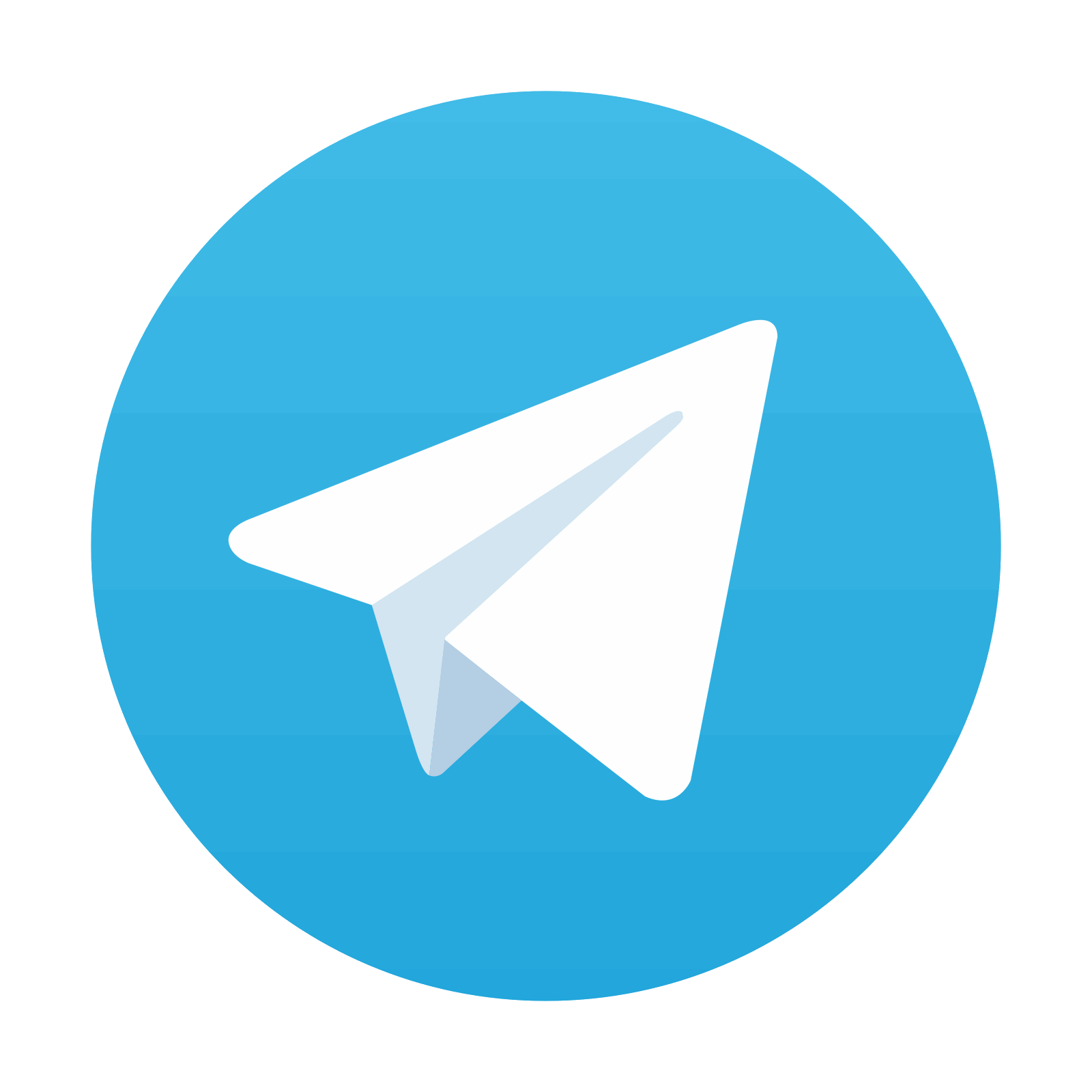
Stay updated, free articles. Join our Telegram channel

Full access? Get Clinical Tree
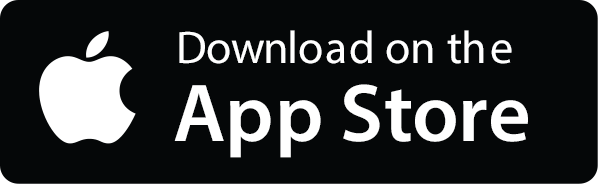
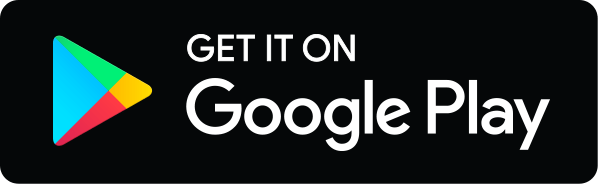