Abstract
The cervical spine’s stabilising function is generated by three interacting systems: an active system (the muscles), a passive system (capsules, intervertebral disks and ligaments) and a neutral system (the nervous system). Functional impairment induced by alteration of one or several systems can disturb movement control. Thus, a decrease in the quality of movement control could be directly linked to the cervical spine’s state of impairment. The aim of the present study was to assess the relationship between cervical spine status (measured using a validated questionnaire) and the control of low-amplitude neck movements. Our starting hypothesis was that the more precise the movement, the faster it would be. We devised a test in which a sequence of rotational movements of the neck (to the left and to the right, alternately) was timed while monitoring the targeting of a laser beam (fixed to the right side of a pair of spectacles) on photodetectors placed directly in front of the subject and 30° to the left and to the right of the body line. The test was performed using a system called the “Didren laser”. Fifty-six subjects (of varying ages and both genders, classified as “disabled” or “healthy” according to the Neck Disability Index [NDI] questionnaire score) performed the test. Our results showed that: the score differed from one individual to another but was reproducible for a given subject; the score was age- and gender-independent; the highest scores (i.e. the slowest rotations) were generally produced by individuals classified as “disabled” in terms of the NDI questionnaire score. Our results led us to conclude that there is a relationship between functional disorders of the cervical spine and low-amplitude rotational movement control, although we were unable to define the exact nature of this relationship.
Résumé
La fonction de la colonne cervicale dépend de trois systèmes interdépendants : l’actif (muscles), le passif (capsules, ligaments, disques intervertébraux) et le neural (système nerveux). Une gène fonctionnelle induite par la déficience d’un ou de plusieurs de ces systèmes, pourrait avoir pour conséquence de perturber le contrôle du mouvement. Par conséquence, une diminution du contrôle du mouvement pourrait être donc directement mise en relation avec l’état déficient de la colonne cervicale. Le but de notre étude était d’objectiver la relation entre le statut fonctionnel du rachis cervical, mesurée par un questionnaire validé et le contrôle des mouvements cervicaux de faible amplitude. Pour réaliser cet objectif, nous sommes partis de l’hypothèse que plus le mouvement réalisé était précis, plus il devait être rapide. Nous avons donc imaginé le test suivant : chronométrer une succession de rotations de nuque alternativement à gauche et à droite en dirigeant le rayon d’un laser accroché sur la branche droite d’une paire de lunette vers des capteurs photosensibles écartés de 30° de part et d’autre de l’axe neutre. Le test a été instrumenté par un dispositif, baptisé Didren laser . Nous avons soumis le test à 56 personnes de différents âges et sexes, classées comme « déficientes » ou « saines » selon leurs réponses au questionnaire NDI. Les résultats au test ont montré que : le temps mesuré était différent entre individus mais reproductible pour chaque individu ; le temps mesuré ne dépendait pas de l’âge ni du sexe ; les temps mesurés les plus élevés (rotations les plus lentes) étaient généralement obtenus par les individus classés « déficients » au travers du questionnaire NDI. Notre étude nous mène à conclure qu’il existe une relation entre gène fonctionnelle de la colonne cervicale et contrôle des mouvements de rotation de faibles amplitudes sans pour autant permettre d’identifier la nature de cette relation.
1
English version
1.1
Introduction
In biomechanical terms, the vertebral column:
- •
enables the performance of smooth intersegment movements;
- •
bears load;
- •
protects the spinal cord and the nerve roots.
Correct movement control of the cervical, thoracic and lumbar spinal is essential for performing these functions .
According to Panjabi, the various structures ensuring vertebral function can be classified into three interdependent systems: the passive, active and nervous systems .
The passive system (composed notably of joint capsules and facets and intervertebral disks and ligaments) ensures the intrinsic stability of the vertebral column . However, it does not contribute significantly to vertebral column stability when movement remains with an amplitude range that is close to the static position. It is only in the zone between the onset of the vertebral segment’s rigidity and the latter’s peak rigidity that the passive elements develop a reactive force which opposes the movement performed , i.e., in the mid-range and at the end of the movement. These components are passive in the sense that they do not produce any movement (given the lack of contractile elements) but they do have a proprioceptive role .
The active system corresponds to the spinal muscles and produces dynamic stability .
The nervous system includes the peripheral nerves and the central nervous system. This system receives information from various sensors, determines the requirements for maintaining vertebral stability and coordinates the muscle system’s responses .
These three systems are closely intermeshed and, under normal conditions, work together in harmony . They provide the mechanical stability required for appropriate movement and monitor the quality of the latter. Dysfunction in one of these systems (following trauma or via a degenerative process) can have an influence on the other systems and endanger the overall stabilization system by perturbing neck movement control .
If the passive system is altered, the role of the muscles becomes primordial in controlling the low-amplitude movements generally used in functional tasks . In neutral positions and low-amplitude rotations, passive resistance to movement is low; the destabilizing force of gravity is countered by the anterior and posterior neck muscles .
According to Falla et al., the deep flexor muscles are primarily responsible for controlling cervical lordosis, maintaining the posture of the cervical spine and thus, stabilizing the vertebral column . In the case of neck pain, the deep muscles are weakened and present a subnormal level of electrical activity, whereas the electrical activity of the superficial muscles increases . The decrease in muscle activity could lead to a reduction in the cortical representation of the stabilizing muscles and thus, hinder automatic contraction during head movement .
Lastly, impairment of the passive system can perturb the nervous system and provoke a decrease in the control exerted by the active system. Consequently, the active system (poorly informed by the nervous system) will have trouble producing adequate muscle force for controlling the movement . The extent of these various interactions shows that the impairment of passive elements (joint capsules and facets and intervertebral disks and ligaments), active elements (spinal muscles) and neural elements (the peripheral and central nervous system) could have an impact on movement control. Thus, impairment of the cervical vertebral column should translate into the imprecise execution of low-amplitude neck movements.
In the present study, we sought to identify a relationship between the neck’s functional status (as measured by a validated questionnaire) and the control of low-amplitude neck movements. Our starting hypothesis was that poor movement control would translate into longer times when performing a series of precise neck movements. Consequently, a decrease in the rapidity of movement execution would be directly related to an impaired cervical spine status. To address this question, a measuring system called the “Didren laser” was developed in order to quantify the time needed to execute a series of low-amplitude neck rotations.
1.2
Methods
1.2.1
Study subjects
The test was performed on 56 subjects of both genders. All had received information on the study’s objectives and procedures and had given their consent to participation. In order to include a population that was as broad as possible in terms of age and functional impairment, subjects were recruited either via an advert displayed at the Haute École Libre paramedical training school in Brussels (Belgium) or from among the patients attending a private physiotherapy practice in the city.
Subjects presenting dizziness, vestibular dysfunction, blindness or deafness were excluded from the study.
Before starting the test, each participant had to fill out the French version of the validated Neck Disability Index (NDI) questionnaire (the intraclass correlation coefficient [ICC] of which is 0.93) . The NDI questionnaire evaluates the functional status of the patient’s cervical spine via ten questions on functional activities, pain intensity, concentration and headaches.
For the purposes of our study, we converted the NDI score into a percentage of the maximum possible score, in order to stratify the subjects into two different groups. Following the classification suggested by Vernon et al. in 1991, subjects with a score between 0 and 8% were considered to be “healthy”. Above 8%, subjects were qualified as “disabled”.
Patients with neck pain had all been diagnosed by their general practitioner. For all participants, we intentionally focused on the NDI score because the medical diagnosis would not have enabled us to quantify functional status in a valid way, as we were able to do with the NDI.
When the test was performed on a subject with neck pain, it was done during the patient’s first visit and did not form part of the treatment programme. Normal medical care was continued after the test.
The subjects were also stratified by gender and age, with “young adult” subjects (20 to 30 years of age) and “older adult” subjects (35 to 61 years of age). The mean ± standard deviation (SD) age of the “young adults” was 23 ± 2.65 and that of the “older adults” was 43.2 ± 6.59).
1.2.2
The test system and software
The test system was named the “Didren Laser” after its designers, Didier Van Oudenhove and Renaud Hage. The software was developed specially for this study, in order to process the data and display them numerically and graphically. The minimum configuration for operating the software is a personal computer running Microsoft Windows 98SE, XP or 2000, with a free USB port. The data were collected and processed using Excel ® and Statistica ® version 6.1.
1.2.3
The pursuit test
The subject sat in a chair facing a wall on which three sensors were aligned horizontally ( Fig. 1 ). The distance between the subject and the wall (52 cm, in our case) is chosen so that the subject produces a 30° rotation either side of the bodyline. Thus, the movement remains within an amplitude range, which does not put the passive system (joint capsules and facets and intervertebral disks and ligaments) under strain but which, in contrast, draws intensively on the active system.
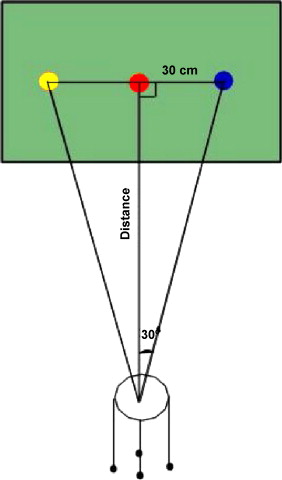
The subject wears a pair of spectacles to which a laser is attached. While the subject fixes his/her gaze on the central sensor, the investigator adjusts the laser beam onto this target by using the ball-and-socket joint system mounted on the right sidepiece of the spectacles. The subject then confirms that the laser is indeed pointing at the spot at which he/she is looking ( Fig. 2 ). Before starting the experiment, each participant is instructed to keep his/her back as straight as possible, place the feet flat on the ground in front, place the hands on the thighs, look straight ahead with the chin slightly lowered, refrain from talking during the test, breathe gently and perform the test as rapidly as possible.
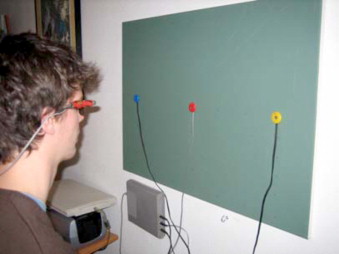
When the laser beam is pointed correctly at the target, the sensor lights up and the system emits a sound signal.
As soon as the central sensor has been “hit”, the subject must turn his/her head to the left in order to hit the left-hand sensor. He/she returns to the central sensor and then moves on to hit the right-hand sensor. The subject completes the cycle by hitting the central sensor for a third time. The software calculates (to the nearest hundredth of a second) the time taken by the subject to go from one “hit” sensor to another.
The measured times were as follows:
- •
the mean time taken to move out to the left (MAG) and to the right (MAD);
- •
the mean time taken to return from the left (MRG) and from the right (MRD);
- •
the mean time spent on the left (MG);
- •
the mean time spent on the right (MD);
- •
the mean left + right time (TmRITouche);
- •
the mean time for 1 cycle (TmR1Cycle) and 5 cycles (TmAbCycle).
Five bar charts displayed the subject’s performance in real time ( Fig. 3 ). Each was composed of four bars (representing the left outward and return journeys and the right outward and return journeys, respectively), above which the time was indicated. The software then calculated the above-mentioned mean times.
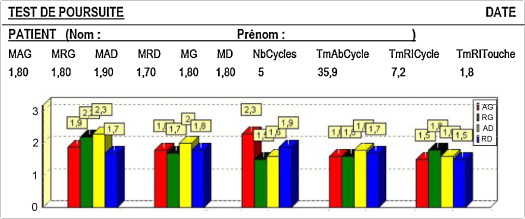
1.2.4
Test procedures
The tests were always performed in the same room with the same light levels. However, the time of day differed for the young adults and the older adults. The younger adult subjects performed the experiments in the evening, whereas the older adults performed them during the day (during consultations at the physiotherapy practice). On arrival, the participant had to fill out the NDI questionnaire (the results of which were not communicated to the participant until after the test). Next, the subject was seated in the test room and given the appropriate instructions. He/she was able to familiarize him/herself by performing a practice cycle prior to the 5 test cycles.
1.3
Results
1.3.1
The study population
The 56 subjects included in the test, distribution is shown in Table 1 .
Healthy subjects | Disabled subjects | Total | |||
---|---|---|---|---|---|
Men | Women | Men | Women | ||
Young adults | 10 | 10 | 5 | 6 | 31 |
Older adults | 8 | 4 | 5 | 8 | 25 |
Total | 32 | 24 | 56 |
1.3.2
Reproducibility of the “Didren laser” test
This calculation was made to check that a subject’s score truly reflected his/her ability, rather than chance. Several healthy and disabled subjects performed the test twice, with an interval ranging from 2 minutes to 10 days. The inter-test interval depended on when the subject was able to return to the investigating centre and repeat the test. The only condition for retesting was the continued absence of dizziness, vestibular dysfunction, blindness and deafness. In all, ten women and 13 men repeated the test (mean ± SD age: 39.87 ± 11.79; range 22 to 61).
The ICC for two successive measurements was 0.9264 ( p < 0.05), attesting to good reliability ( Tables 2–5 ).
Mean time in the first test (seconds) | Mean time in the second test (seconds) | Time interval between the tests | Gender | Age | NDI score (%) |
---|---|---|---|---|---|
2.0 | 2.0 | 30 minutes | Female | 35 | < 8 |
2.9 | 2.7 | 2 minutes | Female | 55 | < 8 |
1.6 | 1.5 | 4 hours | Male | 43 | < 8 |
2.1 | 2.0 | 2 minutes | Male | 55 | < 8 |
1.8 | 1.8 | 2 days | Female | 25 | < 8 |
2.3 | 2.2 | 1 minute | Male | 25 | < 8 |
1.8 | 1.8 | 60 minutes | Male | 45 | < 8 |
2.3 | 2.3 | 1 month | Male | 45 | < 8 |
2.2 | 2.2 | 1 minute | Female | 58 | < 8 |
1.8 | 1.7 | 30 minutes | Male | 61 | < 8 |
2.3 | 2.1 | 4 hours | Female | 23 | < 8 |
2.1 | 2.2 | 45 minutes | Female | 52 | < 8 |
2.0 | 1.9 | 60 minutes | Female | 41 | < 8 |
1.6 | 1.5 | 10 minutes | Male | 22 | < 8 |
2.2 | 1.9 | 90 minutes | Male | 31 | < 8 |
1.9 | 1.7 | 90 minutes | Male | 30 | < 8 |
1.8 | 1.7 | 90 minutes | Male | 28 | < 8 |
2.0 | 1.9 | 90 minutes | Male | 45 | < 8 |
2.4 | 2.5 | 30 minutes | Female | 32 | > 8 |
2.5 | 2.4 | 10 days | Male | 51 | > 8 |
2.5 | 2.6 | 8 days | Female | 36 | > 8 |
2.5 | 2.3 | 40 minutes | Female | 40 | > 8 |
1.7 | 1.7 | 8 minutes | Male | 39 | > 8 |
1st test | 2nd test | |
---|---|---|
Females | 0.261861468 | 0.256347978 |
Males | 0.292464894 | 0.259807621 |
1st test | 2nd test | |
---|---|---|
Healthy | 0.201809992 | 0.205382127 |
Disabled | 0.349284984 | 0.353553391 |
1st test | 2nd test | |
---|---|---|
Older adults | 0.287623491 | 0.302890119 |
Young adults | 0.258843582 | 0.251661148 |
1.3.3
Effect of the “impairment”
The analysis of variance (ANOVA) showed that there was significant overall time difference between healthy and disabled subjects ( p < 0.05) for all movements in the pursuit test ( Table 6 ). Thus, the disabled subjects took longer to complete the pursuit test than the healthy subjects did. The post-hoc test (Fischer’s least significant difference, Table 7 ) indicated that the significant time difference between healthy and disabled subjects was slightly greater for young adults than for older adults ( Table 8 ).
Type of movement | Healthy ( n = 32) | Disabled ( n = 24) | Difference between healthy and disabled | p value |
---|---|---|---|---|
Mean outward L | 2.33 | 2.65 | 0.32 | 0.003 a |
Mean return L | 2.10 | 2.35 | 0.25 | 0.002 a |
Mean outward R | 2.27 | 2.51 | 0.24 | 0.013 a |
Mean return R | 2.10 | 2.28 | 0.18 | 0.015 a |
Mean L | 2.22 | 2.51 | 0.29 | 0.001 a |
Mean R | 2.18 | 2.40 | 0.22 | 0.003 a |
Mean time | 2.20 | 2.45 | 0.25 | 0.0009 a |
Time for 1 cycle | 8.83 | 9.83 | 1.00 | 0.001 a |
Time for 5 cycles | 44.17 | 49.14 | 4.97 | 0.001 a |
Type of movement | Times for the young adults ( n = 31) | Times for the older adults ( n = 25) | ||||
---|---|---|---|---|---|---|
Healthy | Disabled | Difference | Healthy | Disabled | Difference | |
Mean outward L | 2.36 | 2.75 | 0.3 | 2.29 | 2.57 | 0.28 |
Mean return L | 2.11 | 2.47 | 0.3 | 2.09 | 2.24 | 0.15 |
Mean outward R | 2.27 | 2.65 | 0.3 | 2.27 | 2.39 | 0.12 |
Mean return R | 2.12 | 2.41 | 0.2 | 2.06 | 2.17 | 0.11 |
Mean L | 2.24 | 2.61 | 0.3 | 2.20 | 2.42 | 0.22 |
Mean R | 2.19 | 2.54 | 0.3 | 2.16 | 2.28 | 0.12 |
Mean time | 2.21 | 2.58 | 0.3 | 2.17 | 2.35 | 0.18 |
Time for 1 cycle | 8.88 | 10.33 | 1.4 | 8.75 | 9.40 | 0.65 |
Time for 5 cycles | 44.42 | 51.63 | 7.2 | 43.77 | 47.03 | 3.26 |
Young adults | Older adults | |
---|---|---|
Healthy | 0.22070461 | 0.35960837 |
Disabled | 0.32192602 | 0.25695455 |
SD (in seconds) of the mean times of healthy subjects was 0.2759149 and for the “disabled” subjects 0.30491149.
SD (in seconds) of the mean times for the “older adults” and “young adult” subjects are shown in Table 5 .
1.3.4
Relationship between the NDI score and test performance
For the healthy subjects, the Pearson coefficient did not indicate the presence of a significant relationship between the NDI questionnaire score and the times recorded in the pursuit test ( p > 0.05) ( Fig. 4 ).
For the mean times, the SD was 0.276 seconds. In contrast, for disabled subjects, the relationship between the NDI questionnaire score and the values of the pursuit test times was significant. The relationship was positive and non-perfect (< 1), indicating proportionality ( Fig. 5 ). For the mean times, the SD was 0.305 seconds.
1.3.5
Effect of age
An ANOVA did not reveal an effect of age on the pursuit test results ( p > 0.05). There was no significant difference in test times between the young adults and the older adults in either the healthy group ( p = 0.62) or the disabled group ( p = 0.28) or the two groups taken together as shown in Table 9 . SD (in seconds) of the mean times of the adults was 0.31717503 and for the younger adults 0.31181053.
Type of movement | Time for the young adults ( n = 31) | Standard deviation | Time for the older adults ( n = 25) | Standard deviation | Difference in times between young adults and older adults | p value |
---|---|---|---|---|---|---|
Mean outward L | 2.50 | 0.41 | 2.44 | 0.45 | 0.06 | 0.26 |
Mean return L | 2.30 | 0.33 | 2.17 | 0.29 | 0.06 | 0.13 |
Mean outward R | 2.40 | 0.39 | 2.33 | 0.35 | 0.07 | 0.18 |
Mean return R | 2.22 | 0.32 | 2.12 | 0.30 | 0.1 | 0.07 |
Mean L | 2.37 | 0.34 | 2.31 | 0.36 | 0.06 | 0.19 |
Mean R | 2.31 | 0.31 | 2.22 | 0.28 | 0.09 | 0.06 |
Mean time | 2.34 | 0.31 | 2.26 | 0.31 | 0.08 | 0.09 |
Time for 1 cycle | 9.40 | 1.25 | 9.09 | 1.24 | 0.31 | 0.09 |
Time for 5 cycles | 46.98 | 6.22 | 45.46 | 6.19 | 1.52 | 0.09 |
1.3.6
Effect of gender
The ANOVA did not reveal any influence of gender on the various pursuit test results ( p > 0.05) ( Table 10 ). SD (in seconds) is 0.31578826 for the women and 0.30365934 for the men.
Type of movement | Healthy women ( n = 14) | Healthy men ( n = 18) | Disabled women ( n = 14) | Disabled men ( n = 10) |
---|---|---|---|---|
Mean outward L | 2.39 | 2.34 | 2.82 | 2.68 |
Mean return L | 2.09 | 2.13 | 2.53 | 2.40 |
Mean outward R | 2.32 | 2.23 | 2.65 | 2.66 |
Mean return R | 2.12 | 2.12 | 2.43 | 2.40 |
Mean L | 2.24 | 2.24 | 2.68 | 2.54 |
Mean R | 2.22 | 2.17 | 2.55 | 2.54 |
Mean time | 2.23 | 2.20 | 2.54 | 2.54 |
1.4
Discussion
Our results revealed a significant difference in rotational movement execution time when comparing subjects presenting neck disability (i.e., with an NDI score > 8%) with healthy subjects (2.45 seconds vs 2.20 seconds, respectively).
Moreover, we noted that for disabled subjects only, there was a proportional relationship between the NDI questionnaire score and the time recorded in the pursuit test. Hence, the test result reflects well the severity of the impairment, as assessed by the NDI.
When considering the intra-individual reproducibility of the “Didren laser” pursuit test, we note that the ICC for two successive measures (0.9264; p < 0.05) indicated a fair degree of reliability in view of the small sample of subjects tested; this is almost the same value that the individual would obtain if he/she took the test a second time. In this respect, we can consider that the pursuit test time could be a characteristic of the individual (just like age or weight) and would serve as an index of movement control during neck rotation.
Whereas use of the NDI questionnaire enabled us to objectively classify subjects into “disabled” and “healthy” groups, this was also the case for use of the “Didren laser” pursuit test; there is a correlation between the questionnaire score and the pursuit test time.
As specified above (in section 1.2.1), our goal was to evaluate the subjects’ functional status, rather than their degree of neck pain. This is why we classified the subjects according to their score in the NDI – a validated questionnaire for evaluating the cervical spine’s functional status – and not a medical diagnosis. The latter would only have enabled us to evaluate subjects with neck pain, whereas the NDI allowed us to broaden our “disabled” group out to a population of subjects who were not in pain at the time of the study but in whom “disabled” status could be detected. In fact, even though it is common to observe perturbed neck function in a patient with neck pain, it is less easy (but nevertheless relevant) to be able to evaluate a subject during a pain-free or reduced-pain period. Motor control of the neck is not restricted to pain alone. This is why we considered the NDI to be a better guide to the neck’s functional status.
Taken as a whole, the results obtained here with the “Didren laser” system enabled us to identify a relationship between function and movement control during neck rotation. However, observing a relationship does not necessarily mean establishing the nature of this relationship. In fact, on the basis of this test alone and in the absence of a correlation with diagnosis, we cannot identify which of the various systems (active, passive, neural systems) involved in neck control was impaired. Nevertheless, we are tempted to define a pursuit test cut-off time that could enable us to screen for individuals with impaired neck function. This idea is especially attractive, in as much as the study results showed that test performance was independent of the subject’s gender ( p > 0.05) or age ( p > 0.05), even though it has been clearly shown that age limits joint mobility. On the basis of our current statistical analysis, this cut-off value would be situated somewhere between the two mean values (2.20 and 2.45 seconds) for the two categories of subjects, i.e. about 2.3 seconds. However, due to inter-individual differences in performance, some “disabled” subjects recorded better times than some “healthy” subjects ( Figs. 4 and 5 ), which means that this approach would have to be interpreted with caution. Hence, at this stage, using the measurement generated by the “Didren laser” test to characterize a subject when he/she moves from one side of the healthy versus disabled cut-off value to the other would be far too “individual-dependant”, i.e., potentially dependent on a range of personal factors such as concentration, dexterity, etc. This cut-off value could perhaps be refined by testing a larger number of subjects.
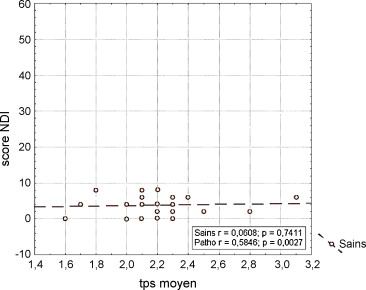
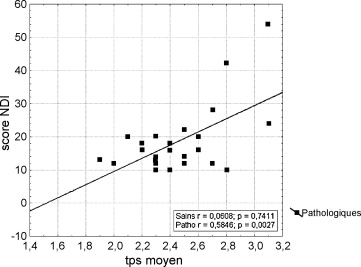
Hence, given that the pursuit test is not intended to classify the individuals as “healthy” or “disabled”, it will perhaps be more useful to monitor the tests from a given subject as part of his/her treatment follow-up. Personal factors should remain constant and so the test result should reflect the severity of the impairment. By monitoring the change over time in the patient’s test result, it should be possible to evidence the recovery of neck function and/or modify the treatment accordingly. However, this application requires further investigation.
Another application for the “Didren laser” test would be as a rehabilitation tool. In fact, Treleaven demonstrated the importance of vision in recovering from sensorimotor impairment of the neck. She recommended rehabilitation that covered movement control of both the neck and the eyes. Furthermore, this approach is comparable to the proprioceptive work implemented for rehabilitation of a sprained ankle. If used as a rehabilitation tool, the “Didren laser” would need to be slightly modified: the sensors would be set further apart or even placed in a triangle, in order to force the patient to make greater rotations or combine the rotations with flexion and extension. The numerical data recorded in the test could be used as a stimulant and the lights on the sensors and the sound signals could be used as “feedback”. However, the value of this method in rehabilitation requires further study.
1.5
Conclusion
Our results prompt us to conclude that there is a relationship between functional impairment of the cervical vertebral column and the control of low-amplitude rotation movements. The “Didren laser” provides a parameter for grading the functional status of a subject’s cervical spine but does not enable establishment of a causal link. In fact, one cannot identify which system or systems (be they active, passive or neural) are impaired and the latter’s impact on control of neck function. It would perhaps be of greater value to build on this result as part of individual patient monitoring or rehabilitation programmes.
Appendix A
This system described here was developed as a prototype. However, in view of the test’s results and its potential value in rehabilitation, commercialisation of the system could be envisaged.
2
Version francaise
2.1
Introduction
Les principales fonctions biomécaniques de la colonne vertébrale sont de permettre le mouvement harmonieux entre les différentes parties du corps, de porter des charges ainsi que de protéger la moelle épinière et les racines nerveuses . Un contrôle correct du mouvement de la colonne aux niveaux cervical, thoracique et lombaire est essentiel pour pouvoir assurer ces fonctions .
Selon Panjabi, nous pouvons classer les différentes structures assurant la fonction vertébrale en trois systèmes interdépendants : le passif, l’actif et le neural .
Le système passif, composé entre autres des capsules articulaires, de facettes articulaires, des disques intervertébraux et des ligaments, assure la stabilité intrinsèque de la colonne vertébrale . Il ne contribue pas significativement à la stabilité de la colonne vertébrale lorsque le mouvement reste dans une amplitude de mouvement vertébral proche de la neutralité statique. C’est seulement dans la zone entre le début de la rigidité du segment vertébral et la rigidité maximale de celui-ci que les éléments passifs développent une force réactive s’opposant au mouvement réalisé , donc en piste moyenne et en fin d’amplitude de mouvement. Ils sont donc passifs dans le sens où ils ne produisent aucun mouvement (pas d’éléments contractiles) mais ils ont un rôle proprioceptif .
Le système actif rassemble les muscles spinaux ; il produit la stabilité dynamique . Le système neural comprend les nerfs périphériques et le système nerveux central. Ce système reçoit les informations en provenance des divers capteurs sensoriels et détermine les différents besoins nécessaires à la stabilité vertébrale ; il coordonne les réponses musculaires .
Ces trois systèmes sont étroitement imbriqués les uns dans les autres et, dans des conditions normales, travaillent en harmonie . Ils fournissent la stabilité mécanique nécessaire au bon déroulement du mouvement et par conséquent assurent une bonne qualité de celui-ci. Le dysfonctionnement de l’un de ces systèmes, suite à un traumatisme ou par dégénérescence, pourrait avoir une influence sur les autres systèmes et mettre en péril tout le système stabilisateur en perturbant le contrôle des mouvements cervicaux .
Lorsqu’il existe une altération du système passif, le rôle des muscles devient primordial pour le contrôle du mouvement dans des petites amplitudes généralement adoptées pendant des tâches fonctionnelles . Dans des positions neutres et pour des rotations de faible amplitude, la résistance passive au mouvement est minimale ; les forces gravitationnelles déstabilisantes sont neutralisées par les muscles cervicaux antérieurs et postérieurs .
D’après Falla D et al., ce sont principalement les muscles fléchisseurs profonds qui assurent le contrôle de la lordose cervicale et le maintien de la forme posturale du rachis cervical, apportant toute la stabilité à la colonne . Dans le cas de cervicalgies, les muscles profonds sont affaiblis et présentent une activité électrique nettement inférieure à la normale alors que les muscles superficiels ont une activité électrique amplifiée . La diminution de l’activité musculaire pourrait engendrer une réduction de la représentation corticale des muscles stabilisateurs et ainsi entraver leur contraction automatique lors d’un mouvement de la tête .
Enfin, l’altération du système passif peut perturber le système neural et provoquer une diminution du contrôle effectué par le système actif. En conséquence, le système actif mal informé par le système neural aura des difficultés à produire une tension musculaire adéquate pour contrôler le mouvement .
Nous constatons par toutes ces interactions que l’altération des éléments passifs (capsules articulaires, facettes articulaires, disques intervertébraux et ligaments), actifs (muscles spinaux) et neural (système nerveux périphérique et central) pourrait avoir des effets sur le contrôle du mouvement. Ainsi, la conséquence d’un état déficient de la colonne cervicale devrait pouvoir s’observer par une imprécision d’exécution d’un mouvement cervical dans une petite amplitude.
Le but de notre étude était de rechercher une relation entre la fonction, mesurée par un questionnaire validé et le contrôle des mouvements cervicaux de faibles amplitudes. Nous sommes partis de l’hypothèse qu’un temps accru pour effectuer avec précision un mouvement cervical pourrait indiquer un moins bon contrôle du mouvement. Par conséquence, une diminution de la rapidité d’exécution du mouvement pourrait être directement mise en relation avec l’état déficient de la colonne cervicale.
Pour réaliser cet objectif, nous avons développé un appareil de mesure, le Didren laser , afin de quantifier le temps mis pour exécuter une petite rotation cervicale.
2.2
Méthode
2.2.1
Participants à l’étude
Le test a été effectué sur 56 sujets. Ils avaient tous reçu une information sur les principes et les objectifs de l’étude et étaient tous consentent.
Dans le but d’élargir le plus possible la population de l’expérimentation au niveau de l’âge, du sexe et de la déficience, les sujets ont été recrutés soit par annonce au sein de la Haute École Libre de Bruxelles, soit parmi les patients d’un cabinet libéral de kinésithérapie à Bruxelles.
Pour être acceptés, les sujets ne devaient présenter ni vertiges, ni dysfonctionnements du système vestibulaire, ni cécité, ni surdité.
Avant de procéder à l’expérimentation, chaque participant a dû remplir un questionnaire d’incapacité cervicale validé (dont l’ICC = 0,93) à savoir la version française du Neck Disability Index (NDI) . Ce questionnaire évalue le statut fonctionnel de la colonne cervicale du patient à l’aide de dix questions relatives aux activités fonctionnelles, l’intensité de la douleur, la concentration et les maux de tête.
Pour notre étude, nous avons ramené le score du questionnaire à un pourcentage afin de cataloguer les sujets dans les différents groupes. Selon la classification de Vernon et al. en 1991, les sujets dont le score était compris entre 0 et 8 % étaient considérés comme « sains ». Au-delà de 8 %, les sujets étaient qualifiés de « déficients ».
Les patients cervicalgiques testés étaient tous diagnostiqués par leur médecin généraliste. Pour tous les participants, nous avons intentionnellement privilégié le questionnaire NDI puisque le diagnostic médical ne nous aurait pas permis de quantifier de façon valide la fonction comme nous avons su le faire avec le NDI.
Quand le test était effectué par un patient cervicalgique, il l’était lors de sa première visite et hors du contexte de traitement. Le traitement se poursuivait par la suite tout à fait normalement.
Les sujets ont également été différenciés selon leur sexe et selon leur âge : les sujets « jeunes » (de 20 à 30 ans) et les sujets « adultes » (de 35 à 61 ans). La moyenne d’âge des « jeunes » était de 23 ans (écart-type 2,65) ; la moyenne d’âge des « adultes » était de 43,2 ans (écart-type 6,59).
2.2.2
Le logiciel
Le nouveau logiciel, appelé Didren Laser du nom de leurs concepteurs Did ier Van Oudenhove et Ren aud Hage, a été développé spécialement pour cette étude afin de traiter les données et de les présenter sous forme de valeurs numériques et graphiques. Ce logiciel demande une configuration minimale à savoir un ordinateur PC avec un port USB libre et un système d’exploitation 98SE, XP, 2000.
Les données sont recueillies et traitées par les logiciels Excel ® et Statistica ® v 6.1.
2.2.3
Le test de poursuite
Le sujet est assis sur une chaise face à un tableau sur lequel sont disposés à l’horizontal trois capteurs ( Fig. 1 ). La distance entre le sujet et le tableau (ici 52 cm) sera choisie de telle façon que la rotation que devra faire le sujet vaudra 30°. Ainsi, le mouvement restera dans une amplitude de mouvement qui devrait mettre moins en tension le système passif (capsules articulaires, facettes articulaires, disques intervertébraux et ligaments) mais qui, en revanche, sollicitera intensivement le système actif.
