Hydroxyapatite Coated Femoral Components
R. Michael Meneghini
William Capello
James D’Antonio
Introduction
For three decades, calcium hydroxyapatite (HA), a naturally occurring calcium phosphate present in tooth enamel and vertebrate bone, has been investigated as an implant coating for fixation of noncemented prostheses in the hip, knee, and other joints. HA coatings for fixation of hip prostheses first were implanted in 1985 by Furlong and Osborn (1) in the United Kingdom and in 1986 by Geesink in the Netherlands (2). Almost 30 years later, revision rates from HA-coated hip prostheses are reported as very low, while patient satisfaction as judged by activity and pain level has been excellent (5,6,7,8,9). A comprehensive review of hydroxyapatite coatings and their application to hip prostheses published in 1996 by Jaffe and Scott (10) found that HA-coated hip stems had, at about 10 years follow-up, proved to be equal to, and in most instances better than, other cementless prostheses as judged by clinical and radiographic measures. In addition, they reported evidence that HA-coated components required a less intimate initial fit to bone at the time of surgery than ingrowth designs.
Early mechanical pushout and fatigue tests of implants and specimens coated with HA by the plasma spray technique showed that mechanical failure of the implant/coating/bone composite could be induced at the bone interface, or within the coating, or between the coating and the substrate (11). The type of failure induced was dependent on a number of factors, including quality of the HA powder delivered to the plasma gun and the roughness of the implant substrate before coating application. Once these factors were understood, the mechanical performance of the coating was found to be dependent on coating thickness (12) with a thick coating more likely to fail mechanically than a thin HA film (13). Thicker coatings could be induced to delaminate from the implant substrate or could be forced to fail within the coating substance. In a transcortical implant model (14) and an intramedullary implant model (15), HA coatings of 50 μm thickness exhibited significantly higher shear strength than 200-μm thick coatings when implants were pushed out of their bone beds. Based on these and similar findings most, but not all, implant manufacturers produced coatings for clinical use with a thickness in the 50- to 75-μm range (12).
Several early preclinical studies demonstrated substantial attachment strength between coated implants and bone (11,13,16). In many models, uncoated control implants tended to be associated with a surrounding seam of fibrous tissue at sacrifice, while bone proliferation on the surface of an HA-coated implant of the same design was shown to occur within 6 to 10 weeks of implantation (2,17). Bone bridges of up to 2 mm were demonstrated when stable implants had interface regions where bone and coating were not in initial contact (18,19). Animal models were developed by Søballe et al. (22,23) and demonstrated the ability of an HA coating to induce gap filling when implants designed to provide predetermined spaces between coating and bone were implanted in dogs. Even more impressive were the micromotion studies reported by the same group. When spring-loaded HA-coated implants were placed in the femoral condyle of dogs, bone attachment to the coating at levels of interface micromotion of up to 500 μm was achieved. Findings such as these support the contention of Jaffe and Scott (10) that initial fit may not be as crucial with HA-coated clinical devices as other designs.
In vivo studies have demonstrated the biocompatibility of HA coatings, predominantly when used with titanium or titanium alloy substrates (13,16,24,25,26). The versatility and generally benign nature of calcium phosphate materials are widely reported, and few negative reactions have been described. An inflammatory response to HA in suspension was reported in rats (27,28), while a slight inflammatory response in the periprosthetic environment was shown with coated implants in dogs when compared to controls (29). An attractive feature of calcium phosphates, in general, is their overall lack of toxicity (25,30,31). The rapid development of an environment rich in calcium and phosphate ions close to an HA coating has been shown to contribute to early bone ongrowth (32,33) and to enhance the potential for developing a bond between HA and bone (32,33,34,35,36). In some instances, HA coatings appear to promote the growth of bone into areas it would otherwise not occupy (37,38).
Coating chemistry controls the rate of coating dissolution in the body. Amorphous calcium phosphate is removed more quickly than tetracalcium phosphate (TTCP), which in turn is removed more quickly than tricalcium phosphate (TCP). All dissolve more rapidly than hydroxyapatite. Thus,
the dissolution rate of an “HA” coating is dependent on the fraction of TTCP, TCP, and other calcium salts present in the final product. In addition to chemical composition, the solubility of the coating is determined by its crystallinity; the greater the crystallinity, the more stable the coating. However, the more stable the coating, the less is its biologic activity. In one study, the environment close to low and to high crystallinity coatings placed in dogs was studied at the ultrastructural level using environmental scanning electron microscopy (ESEM) and transmission electron microscopy (TEM) (17). At 3 hours postimplantation, plate-like apatite crystals were visible in the vicinity of the lower crystallinity coatings, but were not visible near the higher crystallinity coatings. These data add support to the proposed mechanism underlying the bioactivity of HA coatings: Dissolution of calcium and phosphate ions result in the supersaturation of the surrounding body fluid and promote the reprecipitation of carbonated apatite crystallites on the surface of the implant. Subsequently, it was proposed that the biologic activity surrounding any coating simply depends on the roughness of the surface presented to the body rather than on the chemistry provided by the presence of a hydroxyapatite coating (39). Bone apposition to canine femoral intramedullary implants coated with a plasma-sprayed hydroxyapatite was compared to bone apposition to identical implants masked with a surface film of titanium placed on top of the hydroxyapatite. The film preserved the surface topography of the HA coating but prevented release of calcium phosphate salts to the implant interface. The authors argued that surface roughness was the dominant effect in bone apposition although the masked implants had less than 80% of the bone apposition as that measured for the implants with exposed HA coating. One caution with their results was that the implants were unloaded. The dental literature shows that even smooth titanium implants can become osseointegrated as long as the implant remains unloaded but will not osseointegrate in the presence of loading and associated micromotion (40).
the dissolution rate of an “HA” coating is dependent on the fraction of TTCP, TCP, and other calcium salts present in the final product. In addition to chemical composition, the solubility of the coating is determined by its crystallinity; the greater the crystallinity, the more stable the coating. However, the more stable the coating, the less is its biologic activity. In one study, the environment close to low and to high crystallinity coatings placed in dogs was studied at the ultrastructural level using environmental scanning electron microscopy (ESEM) and transmission electron microscopy (TEM) (17). At 3 hours postimplantation, plate-like apatite crystals were visible in the vicinity of the lower crystallinity coatings, but were not visible near the higher crystallinity coatings. These data add support to the proposed mechanism underlying the bioactivity of HA coatings: Dissolution of calcium and phosphate ions result in the supersaturation of the surrounding body fluid and promote the reprecipitation of carbonated apatite crystallites on the surface of the implant. Subsequently, it was proposed that the biologic activity surrounding any coating simply depends on the roughness of the surface presented to the body rather than on the chemistry provided by the presence of a hydroxyapatite coating (39). Bone apposition to canine femoral intramedullary implants coated with a plasma-sprayed hydroxyapatite was compared to bone apposition to identical implants masked with a surface film of titanium placed on top of the hydroxyapatite. The film preserved the surface topography of the HA coating but prevented release of calcium phosphate salts to the implant interface. The authors argued that surface roughness was the dominant effect in bone apposition although the masked implants had less than 80% of the bone apposition as that measured for the implants with exposed HA coating. One caution with their results was that the implants were unloaded. The dental literature shows that even smooth titanium implants can become osseointegrated as long as the implant remains unloaded but will not osseointegrate in the presence of loading and associated micromotion (40).
Clinically, hydroxyapatite-coated implants have been used for over 25 years. Reports of clinical results of increasing duration show general clinical success with hip stems in both proximally coated designs (10,41,42,43,44,45) and fully coated designs (1,20,33,45). Reported complications associated with the coating or its dissolution products are scant. Suggestions that coating fragments may be related to third-body bearing wear (47) have been largely refuted (47). In fact, lack or prevention of particle intrusion into fixation interfaces has been one of the key findings of early clinical studies (44,48,49,50). Similar findings have been shown in animals. For example, a study of implants placed in communication with the joint space into which the particles in the size range from 0.2 to 11 μm were injected showed only a few particles of polyethylene around HA-coated implants, but large numbers of particles around uncoated implants (51).
Several histologic examinations on proximally HA-coated implants retrieved at autopsy have shown consistent evidence of new bone formation in the tissue adjacent to the HA coating (1,2,17,47). Evidence of some areas of coating removal in vivo seen in some studies of autopsy and retrieval specimens have not been associated with indications that the long-term clinical outcome is affected. Indeed, Bauer et al. (52) state that the coating becomes part of the bone remodeling that occurs (according to Wolff law) because of the changed state of stress in bone occasioned by the presence of the implant.
On the acetabular side, earlier concerns that HA coatings did not provide adequate fixation for the socket (50) have been resolved largely as a design issue rather than a coating issue. Later reports with interlocked socket designs show survivorship comparable to the best porous devices (49,51,53).
In 1992, we reported initial results of a multicenter clinical trial of HA-coated hip stems (Omnifit-HA, Osteonics, Allendale, NJ) conducted at 15 sites in the United States. (48). Excellent stem survivorship was demonstrated. In 1998 we analyzed the performance of the three acetabular components implanted in this same patient group and reported good results with a threaded HA-coated cup (Omnifit-HA Threaded, Osteonics), good results with a porous cup (Dual-Geometry, Osteonics, Allendale, NJ), and poor results with the smooth HA-coated cups (Dual-Geometry HA/Dual-Radius-HA, Osteonics) (50). This chapter will analyze the performance of the original stems and cups at 11 to 15 years postimplantation and to review a shorter-term study of the second-generation press-fit HA cups implanted with the same stem type.
Clinical Experience—Hydroxyapatite-Coated Femoral and Acetabular Components
Materials and Methods
Between January 1988, and November 1990, 436 HA-coated femoral components were placed in 380 patients in a multicenter, IDE study involving 18 surgeons at 15 sites in the United States. Early clinical data have been previously reported (42,48,54). At the end of an initial 5-year commitment to the study, five surgeons at four sites who contributed 66 hips in 52 patients elected to leave the study. We later reported data on 274 patients (314 hips) with a 10-year minimum follow-up (43
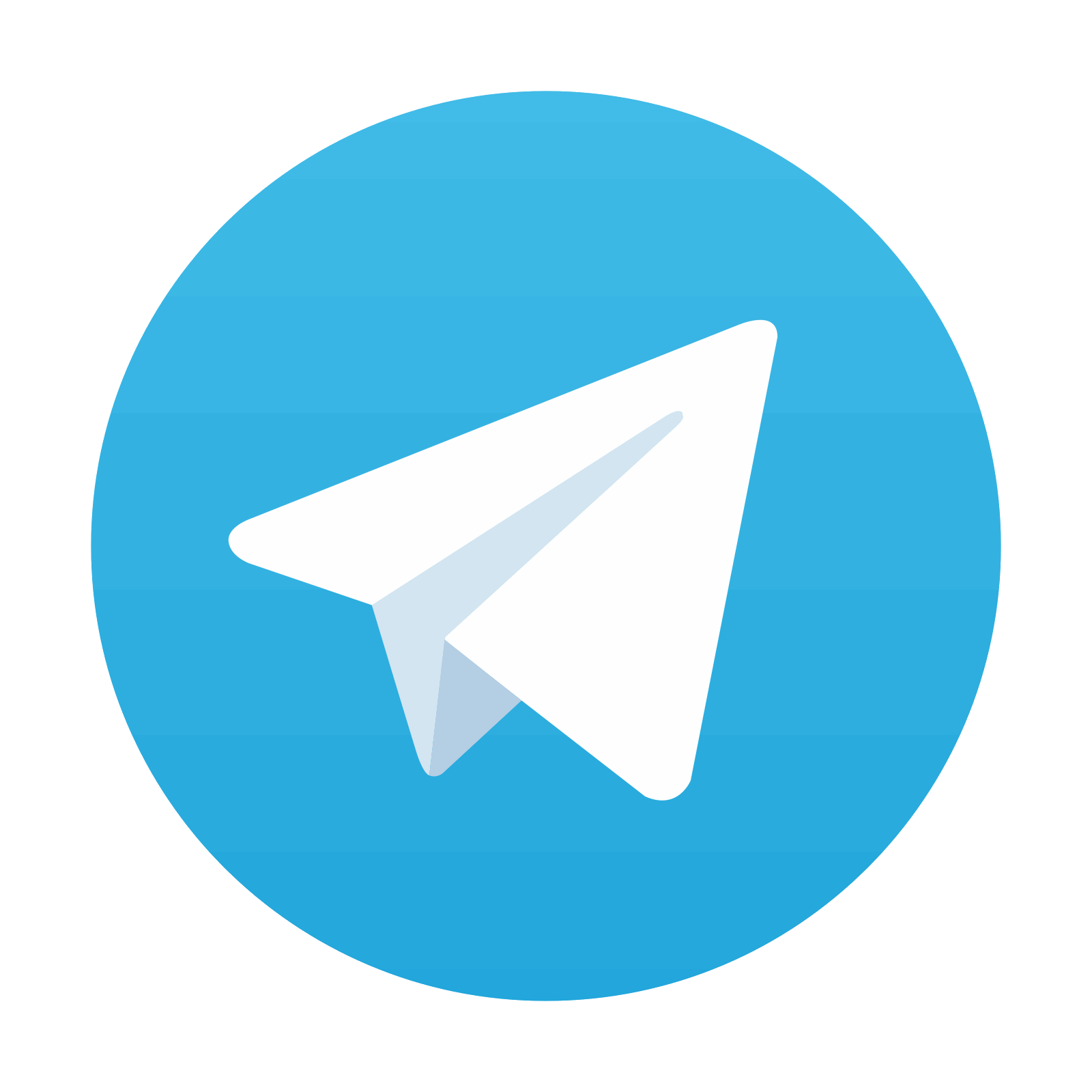
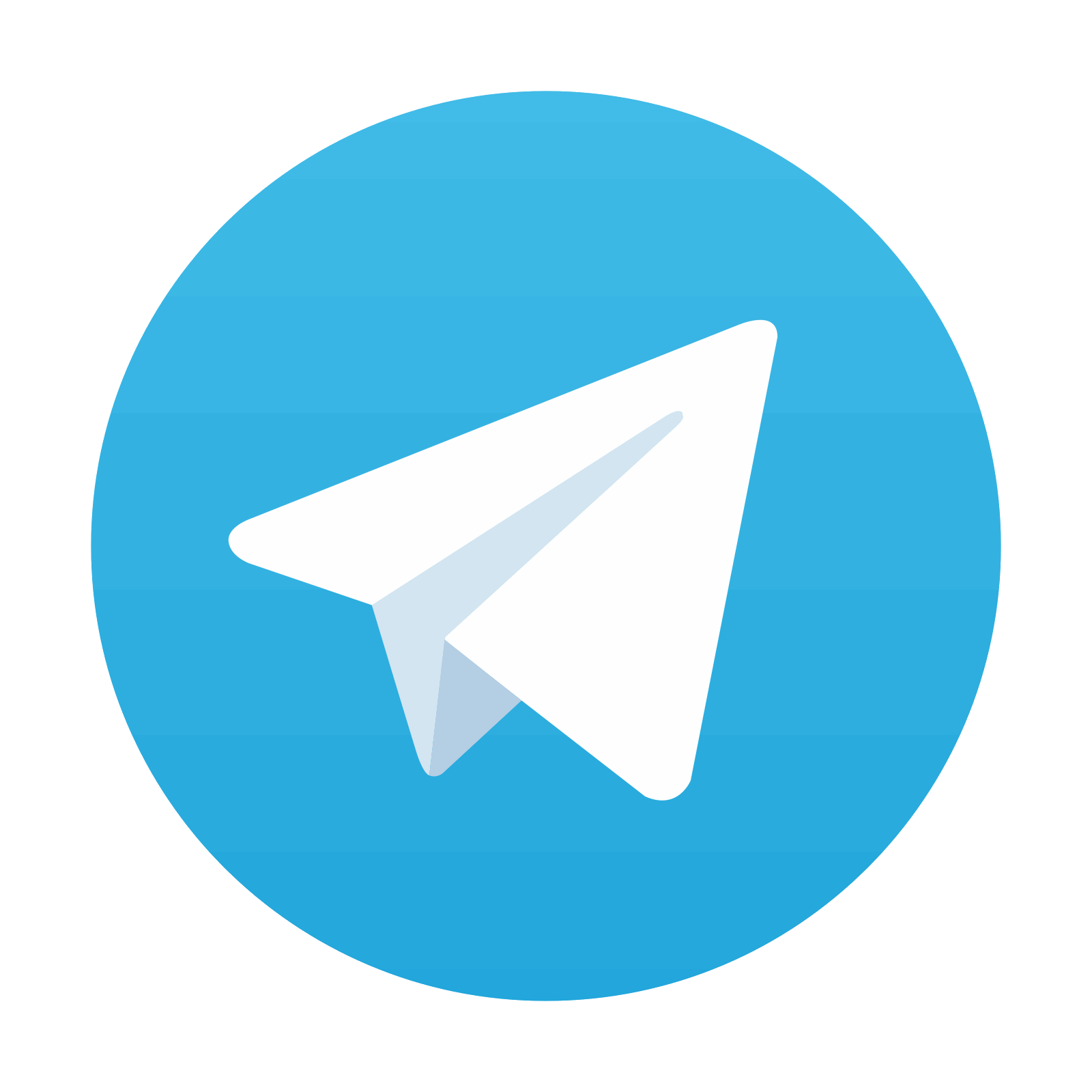
Stay updated, free articles. Join our Telegram channel

Full access? Get Clinical Tree
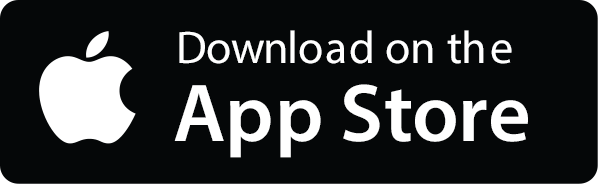
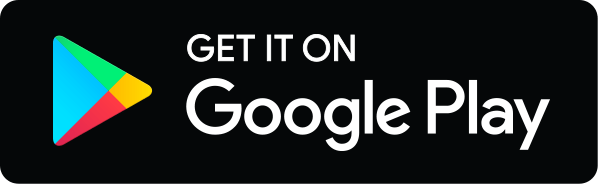
