Computer-assisted orthopedic surgery (CAOS) was introduced, developed, and implemented in musculoskeletal tumor surgery recently to enhance surgical precision in resecting malignant and benign tumors. The origins of computer-assisted surgery were in other subspecialties including maxillofacial surgery, spine surgery, and arthroplasty. Early studies have shown that CAOS can also be used safely for bone tumor resection surgery. Additional technological improvements may allow use of CAOS in soft tissue tumor surgery. It has the potential to improve surgical precision and accuracy, but more study is needed to evaluate clinical efficacy and long term results.
Key points
- •
CAOS was implemented in musculoskeletal tumor surgery to enhance surgical precision.
- •
More study is needed to evaluate clinical outcomes in patients where CAOS is used to resect bone tumors.
- •
Future applications for CAOS include resection of soft tissue tumors.
Introduction
Computer-assisted orthopedic surgery (CAOS) was introduced in musculoskeletal tumor surgery recently to enhance surgical precision in resecting malignant and benign tumors. The origins of computer-assisted surgery were in other subspecialties; it has been used for more than 2 decades in maxillofacial surgery, and in neurosurgery for cranial biopsies and tumor resections. More recently, it has been used in orthopedic surgery for trauma, spine surgery for pedicle screw insertion, cup placement in hip arthroplasty, total knee replacement, and reconstructive surgery.
The initial use of intraoperative computer assistance for musculoskeletal tumor surgery was prompted by the difficulty in treating malignant pelvic and sacral tumors. A simulation by Cartiaux and colleagues quantified this well-known difficulty, showing that the probability of experienced surgeons achieving negative surgical margins on plastic pelvic models was only 52%. A subsequent model showed that introducing CAOS improved cutting accuracy, and therefore the precision, of tumor resection.
The first nontumor computed tomography (CT)–based computer-assisted osteotomy of the bony pelvis was performed in 1995 in patients with acetabular dysplasia, with the goal of improving the precision of required cuts for corrective periacetabular osteotomies. Hufner and colleagues, were the first to use computer-navigated instruments (chisels) to perform osteotomies in patients with sacral tumors. They demonstrated the advantage of visualizing the relationship between the chisel and the tumor for more accurate tumor resection. This technique was subsequently improved and expanded by various groups. Krettek and colleagues added K-wires to visualize the preoperatively planned osteotomy levels for resecting pelvic tumors. Wong and colleagues attached navigation trackers to conventional instruments (diathermy handle) to visualize the tumor in real time on the virtual CT images displayed on the navigation monitor. This visualization enabled clear identification of where cuts should be made for negative surgical margins through marking the bone surfaces with the diathermy needle.
The pace of development of CAOS has increased in the last 5 to 7 years as computer technology has become more sophisticated and intraoperative imaging systems have improved. The potential for enhanced surgical precision has remained the impetus for increased interest by musculoskeletal oncologic surgeons. Current developments in CAOS for benign and malignant tumor resection are reviewed elsewhere in this issue. This article describes the technique, examines the limitations of navigation systems, and reviews recent clinical progress.
Introduction
Computer-assisted orthopedic surgery (CAOS) was introduced in musculoskeletal tumor surgery recently to enhance surgical precision in resecting malignant and benign tumors. The origins of computer-assisted surgery were in other subspecialties; it has been used for more than 2 decades in maxillofacial surgery, and in neurosurgery for cranial biopsies and tumor resections. More recently, it has been used in orthopedic surgery for trauma, spine surgery for pedicle screw insertion, cup placement in hip arthroplasty, total knee replacement, and reconstructive surgery.
The initial use of intraoperative computer assistance for musculoskeletal tumor surgery was prompted by the difficulty in treating malignant pelvic and sacral tumors. A simulation by Cartiaux and colleagues quantified this well-known difficulty, showing that the probability of experienced surgeons achieving negative surgical margins on plastic pelvic models was only 52%. A subsequent model showed that introducing CAOS improved cutting accuracy, and therefore the precision, of tumor resection.
The first nontumor computed tomography (CT)–based computer-assisted osteotomy of the bony pelvis was performed in 1995 in patients with acetabular dysplasia, with the goal of improving the precision of required cuts for corrective periacetabular osteotomies. Hufner and colleagues, were the first to use computer-navigated instruments (chisels) to perform osteotomies in patients with sacral tumors. They demonstrated the advantage of visualizing the relationship between the chisel and the tumor for more accurate tumor resection. This technique was subsequently improved and expanded by various groups. Krettek and colleagues added K-wires to visualize the preoperatively planned osteotomy levels for resecting pelvic tumors. Wong and colleagues attached navigation trackers to conventional instruments (diathermy handle) to visualize the tumor in real time on the virtual CT images displayed on the navigation monitor. This visualization enabled clear identification of where cuts should be made for negative surgical margins through marking the bone surfaces with the diathermy needle.
The pace of development of CAOS has increased in the last 5 to 7 years as computer technology has become more sophisticated and intraoperative imaging systems have improved. The potential for enhanced surgical precision has remained the impetus for increased interest by musculoskeletal oncologic surgeons. Current developments in CAOS for benign and malignant tumor resection are reviewed elsewhere in this issue. This article describes the technique, examines the limitations of navigation systems, and reviews recent clinical progress.
CAOS principles and methods
Computer-assisted navigation provides a real-time, interactive, 3-dimensional (3D) digital map of a patient’s anatomy, and helps track surgical instruments during the procedure. CAOS methods increase spatial accuracy during dissection, surgical resection, instrumentation, or other actions. The principles of CAOS are straightforward and similar to real-time interactive maps that use GPS (global positioning system) technology. Namely, a virtual or digital 3D patient is created from imaging studies, which is used to guide surgical instruments that are tracked and mapped to this simulation. The essential steps for using CAOS are as follows:
- 1.
Creation of a digital patient image, most commonly using magnetic resonance imaging (MRI) or CT scan of the anatomic region of interest, which will be used as a template. For oncology, the tumor is included in the image, and can be highlighted and distinguished from normal tissue to aid in visualization.
- 2.
Preoperative planning of the surgical resection planes (ie, cuts in the bone and soft tissue) on the virtual patient image.
- 3.
Viewing of the digital patient image intraoperatively by the surgeon on a video monitor.
- 4.
Intraoperative matching of the patient anatomy with the digital patient image using a navigated device to localize anatomic landmarks on the patient—a process called registration . The surgeon validates the registration to ensure that an accurate matching has occurred between actual and virtual patient anatomy.
- 5.
Use of navigated instruments whose positions are transferred into the digital patient image in real time to carry out the preoperative plan.
The source of errors in using CAOS systems are primarily caused by mismatch between the preoperatively acquired image and the real-time intraoperative patient anatomy. Consequently, the most recent process optimization strategies have focused on acquiring patient imaging intraoperatively via CT or MRI. Even without the intraoperative imaging, navigated accuracy errors of less than 0.5 mm are routinely reported.
Image-free navigation systems (eg. where no preoperative imaging is performed) are also available, but for tumor resections, only image-based systems have been used, because patient-specific surgical planning is required. Typical components of a navigation system include a stereoscopic optical tracking system, positioning sensors placed on the patient, tracked instruments, a video monitor to display virtual information, and a computer ( Fig. 1 ). The centerpiece of a navigation system is the tracking system.
Tracking can be performed optically or electromagnetically. The most common systems are optical, and use either passive infrared light–reflecting spheres, or active infrared light–emitting diodes in the surgical field. Electromagnetic tracking systems have fallen into disfavor for CAOS because of their inferior accuracy and the risk of malfunction from ferromagnetic and electromagnetic fields from other instruments commonly present in operating rooms. The primary disadvantage of optical systems is the need for an uninterrupted line-of-sight between the sensors and tracked devices/instruments. Before surgery, the computer-navigated instruments must be calibrated for their geometry and shape to be incorporated in the virtual image of the patient. Current navigation systems are capable of tracking common surgical instruments, including osteotomes, diathermy handles, and drills.
Preoperative planning
Preoperative planning has been emphasized to achieve the intended surgical goal. Current systems allow the surgeon to view the patient images in 3D, and therefore accurately identify the tumor’s spatial relationship to vital structures (eg, nerves, blood vessels). Recent recommendations also include using a large high-definition video monitor to minimize errors while viewing patient images.
During preoperative planning, the appropriate patient images (most commonly CT and MRI scans) are selected and can be combined or overlapped. The MRI adds soft tissue information to a CT scan, whereas other modalities, such as positron emission tomography (PET), can add physiologic information. The major steps in planning include highlighting the tumor, selecting reference markers for registration, and planning and placing directional planes of reference for osteotomies ( Fig. 2 ). Annotations can be added to the images that will aid subsequent intraoperative execution of the plan. Finally, sites can be selected for the placement of patient tracking devices.
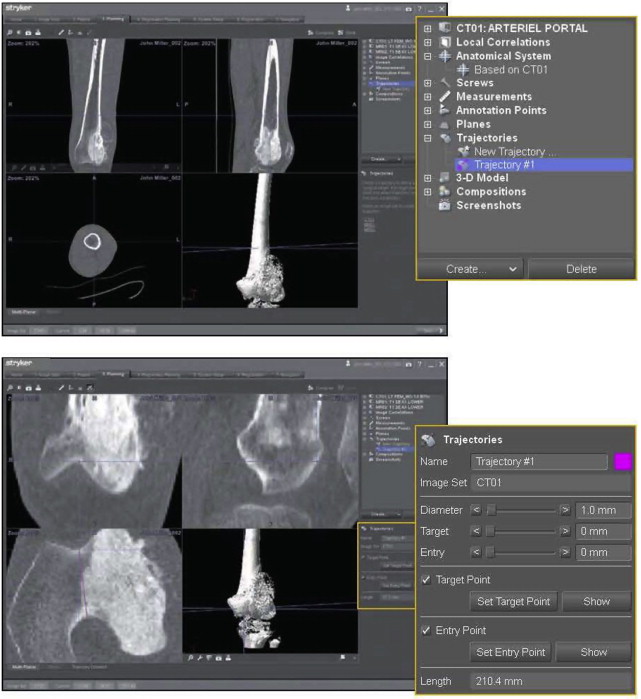
Registration of patient to image
Intraoperative registration of the patient to the patient images is the key step in the navigation process. Numerous registration concepts have been applied successfully for CAOS, using associated algorithms and hardware. The goal of registration is to provide a one-to-one correspondence between the anatomic region of the patient and the virtual 3D model that was constructed from patient images. To compensate for intraoperative real-time patient movement, a tracking mechanism is also established, which creates a local coordinate system (dynamic reference frame) that moves with the patient. Calibration is subsequently performed with pointers, tracked surgical instruments, patient trackers, and intraoperative imaging devices to define the projection of these objects within the virtual coordinate system in real time, and therefore execute the intended surgical plan ( Fig. 3 ).
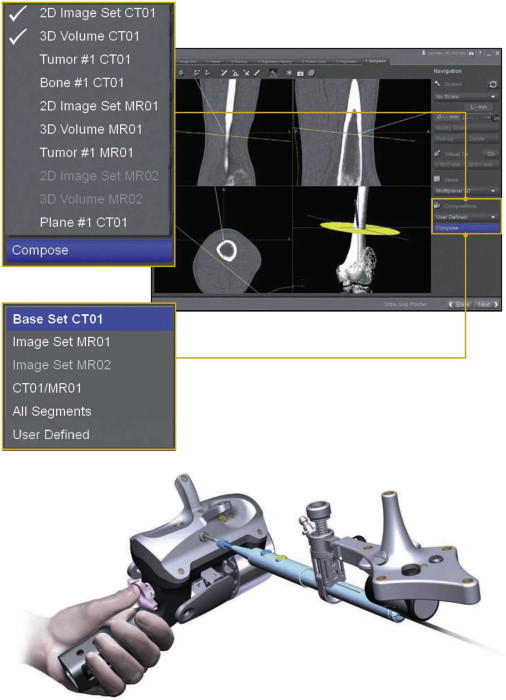
Three-dimensional intraoperative registration is typically performed through identifying anatomic features that are clearly represented in the virtual patient image. Two methods are most often used:
- 1.
Paired point matching (PPM): this is an invasive method that uses fixed markers implanted preoperatively in a separate procedure. These markers are radiopaque, and therefore identifiable on both the patient and the patient images. For registration, the paired points are localized using a pointer on the patient, and matched with the image of the paired points. This approach provides the best accuracy possible. However, the risks include infection, pain, and the time associated with a separate procedure. A noninvasive variation of this technique can also be used, whereby the surgeon finds anatomic points that are precisely identifiable on the patient image. This technique is performed in the preoperative planning stage, and tends to be time-consuming. With both invasive and noninvasive PPM, the registration error decreases significantly when more than 4 pair points are used.
- 2.
Intraoperative imaging: registration can also be performed using an intraoperative imaging device. A dynamic reference frame is fixed to the patient, imaging is performed, and the system completes the registration through matching intraoperative features with features from the preoperative patient images. The benefits of this method are improved accuracy, speed, and reduced patient morbidity from the surgical exposure required for PPM registration. The disadvantages include equipment costs, additional radiation exposure from radiographs, and the typical poorer quality of intraoperative images compared with preoperative images.
In order to improve the accuracy of feature based registration, surface matching is routinely performed ( Fig. 4 ). For this technique, the surgeon identifies 20 or more bone surface points using a pointer tracked by the navigation system. This “real” bone surface is then matched to the imaged bone surface. Increased accuracy is achieved by matching a greater number of points, and if more complex surfaces are selected. Some commercial navigation systems can bypass this step by using imaging data acquired intraoperatively ( Fig. 4 b). After successful patient registration and instrument calibration, the patient anatomy and movements of calibrated instruments in the region of interest are navigated in real time using a video monitor ( Figures. 5 and 6 ).
